Abstract
This study was conducted to determine the effects of diet supplementation of laying hens with Ligustrum lucidum (LL) on egg production, egg quality and caecal microbiota. A total of 360 72-week-old Hy-Line Brown laying hens were divided into three groups with four replicates of 30 birds each. The laying hens were fed with basal diet (control group), basal diet + 1% LL (group I) and basal diet + 2% LL (group II). The experiment lasted for 45 d. Eggs were collected daily and caecal samples were collected at the end of the experiment. Results showed that dietary supplementation with LL did not affect the average daily egg weight, the average daily feed intake, the cracked egg rate, the mortality and the egg quality (p>.05). However, groups I and II showed significantly increased laying rate and decreased feed/egg ratio (p<.05). The differences in caecal microbiota between group II and the control group were significant. The relative abundance of Bacteroidetes, Firmicutes, Saccharibacteria and Verrucomicrobia at the phylum level; Ruminococcaceae, Rikenellaceae, Acidaminococcaceae, unclassified_Bacteroidales, S24-7 group and ODP1230B8.23 at the family level; RC9 gut group, Phascolarctobacterium, unclassified_Bacteroidales, Butyricicoccus, norank_f__Ruminococcaceae, Ruminococcaceae_UCG-014, coprostanoligenes group and Ruminococcaceae_UCG-005 at the genus level in group II changed significantly compared with that in the control group (p<.05). Dietary supplementation with 1% and 2% LL could improve the laying performance and affect the caecal microbial community structure of laying hens during the late laying period.
Dietary supplementations with Ligustrum lucidum significantly increased laying performance of hens during the late laying period.
Dietary supplementations with Ligustrum lucidum not affected the egg quality of laying hens.
Dietary supplementations with Ligustrum lucidum affected the microbial structure of caecum of laying hens.
Highlights
Introduction
The trend in the use of phytobiotics in animal feed has been increased during last two decades. Phytobiotics includes a wide range of plant-derived products such as essential oils, herbs and oleoresins (Mohammadi Gheisar and Kim Citation2018). Ligustrum lucidum (LL) is a crucial Chinese herb rich in oleanolic acid, ursolic acid, triterpenoids, iridoids, flavones and phenolic glucosides (Huang and Wang Citation2011). Oleanolic and ursolic acids are well known for their anti-oxidant and anti-inflammatory activities (Liu Citation2005). Oleanolic acid also has higher antibacterial activity than ursolic acid (Ayeleso et al. Citation2017). In China, LL is traditionally used as a tonic agent to nourish the liver and kidney, strengthen the bone and muscle and prevent some diseases, such as diabetes (Gao et al. Citation2009; Li et al. Citation2015). Modern medical studies have demonstrated that LL possesses the functions of immune regulation, antitumor and anti-inflammatory; it can also protect cells from lipid peroxidation damage stimulated by oxidative stress (Huang et al. Citation2010; Hu et al. Citation2014; Liu et al. Citation2014). Recent studies on LL supplementations illustrated that LL could increase the laying rate; decrease the mortality and cracked-egg rate of laying hens; improve the biochemical blood markers, immune function, and antioxidant status and reduce the harmful effects of heat stress on hens (Ma et al. Citation2005; Ma et al. Citation2007; Li et al. Citation2017). They could also improve the growth performance of broilers and growing–finishing pigs (Chen et al. Citation2009; Zhang et al. Citation2012).
Gut microbial communities play a vital role in the health and function of the host. Animal gut microbiota are a complicated and diverse system easily affected by many factors, such as the environment, age, diet, feed additive, and hygiene level. Diet-related differences are the main cause of the total variations, indicating that diet components could influence the composition and diversity of gut microbiota (Zhang et al. Citation2010).
Hen egg production and egg quality generally decrease with age, thereby reducing the forming profits of poultry farmers. Therefore, keeping the egg production at a high rate is important. Few reports on the effects of LL as a feed additive on poultry diets are available, particularly on the intestinal microbiota in late laying period. Thus, the present study was conducted to determine the effects of dietary supplementation with LL on the egg production, egg quality, and caecal microbiota of laying hens during the late laying period to provide valuable references for the application of LL as a feed additive.
Materials and methods
The animal experimentation procedures were approved by the Institutional Animal Care and Use Committee of Henan University of Science and Technology, Luoyang, China.
LL Preparation
LL fruits were purchased from a Chinese herbal market in Luoyang, Henan Province, China. The main active constitutes of fresh LL fruits were investigated using HPLC-photodiode array detection previously (Li et al. Citation2017) in our institute: oleanolic acid (7.49 mg/g), ursolic acid (1.607 mg/g) and total flavonoids (78.142 mg/g). After the LL was dried at 60 °C, it was grounded into fine powder in a knife mill and strained through a 40-mesh sieve.
Diets, experimental design and laying hens
A total of 360 72-week-old Hy-Line Brown laying hens were assigned to three groups, with four replicates of 30 hens in each group. The hens were housed in 120 cages with a size of 64 cm × 35 cm × 35 cm (three hens each). The hens had free access to feed and water during the experiment and exposed to a 16:8 light–dark cycle. The average room temperature was 20 °C ± 3 °C. The differences in egg production rate among the three groups before the start of the experiment were insignificant.
The laying hens were fed with basal diet (control group), basal diet + 1% LL (group I) and basal diet + 2% LL (group II). The diets were formulated to be isocaloric and isonitrogenous and to meet the nutrient requirements of laying hens according to the Management Guide of National Research Council (NRC, 1994). Table shows the composition and nutrient levels of the basal diet. Crude protein (method 976.06), available phosphorus (method 993.31), calcium (method 927.02) and amino acid composition (method 994.12) were analysed in accordance with the method of AOAC (1999). The experiment lasted for 45 d.
Table 1. Ingredient composition and analysed nutrient contents of the basal diet [g/kg] (data is based on feed).
Data collection
Egg production
Daily records of egg production, cracked-eggs, egg weight, hen mortality and feed consumption were maintained during the experimental period. Laying rate (including cracked eggs), average daily egg weight, average daily feed intake, cracked-egg rate and feed/egg ratio were analysed.
Egg quality
Six saleable eggs (i.e., no shell defects or cracks) were randomly selected from each replication on day 45 and utilised to determine the egg quality. Egg albumen height and Haugh units were determined using a digital egg tester (TSS, England, UK), and eggshell breaking strength was determined using In-Spec 2200 (Instron Corporation, Canton, Massachusetts, USA). Egg shape index was assessed using the following formula: vertical diameter/transect diameter. Eggshell thickness was assessed using a propeller micrometre and a spiral rangefinder.
Caecal microbiome
Four hens were randomly selected from the control group and group II (one for each replication) and sacrificed on the last day of the experiment. Caecum samples were aseptically collected from each bird, immediately stored at −80 °C and promptly processed.
DNA extraction and PCR amplification
Microbial DNA was extracted from the caecum samples by using the EZNA Soil DNA Kit (Omega Bio-tek, Norcross, GA, USA) in accordance with the manufacturer’s protocols. Final DNA concentration and purification were determined using a NanoDrop 2000 UV vis spectrophotometer (Thermo Scientific, Wilmington, USA), and DNA quality was checked via 1% agarose gel electrophoresis. The V3–V4 hypervariable regions of the bacterial 16S rRNA gene were amplified with primers 341 F (5′-CCTAYGGGRBGCASCAG-3′) and 806 R (5′-GGACTACNNGGGTATCTAAT-3′) on a thermocycler PCR system (GeneAmp 9700, ABI, USA). PCRs were conducted as follows: denaturation at 95 °C for 5 min, followed by 27 cycles at 95 °C for 30 s, 55 °C for 30 s, and 72 °C for 45 s and a final extension at 72 °C for 10 min. They were performed in triplicate with 20 μL of mixture containing 4 μL of 5 × FastPfu buffer, 2 μL of 2.5 mM dNTPs, 0.8 μL of each primer (5 μM), 0.4 μL of FastPfu Polymerase and 10 ng of template DNA. The PCR products were electrophoresed using 2% agarose gel, further purified using the AxyPrep DNA Gel Extraction Kit (Axygen Biosciences, Union City, CA, USA) and quantified using QuantiFluor-ST (Promega, USA) in accordance with the manufacturer’s protocol.
Illumina MiSeq sequencing
The purified amplicons were pooled in equimolar and paired-end sequenced (2 × 300) on an Illumina MiSeq platform (Illumina, San Diego, USA) in accordance with the standard protocols of Majorbio Bio-Pharm Technology Co., Ltd (Shanghai, China). The raw reads were deposited into the NCBI Sequence Read Archive database (accession no.: SRP158075).
Sequencing data processing
Raw fastq files were demultiplexed, quality filtered using Trimmomatic and merged using FLASH with the following criteria: (i) the reads were truncated at any site receiving an average quality score < 20 over a 50-base pair (bp) sliding window; (ii) the primers were exactly matched, thereby allowing two-nucleotide mismatching, and the reads containing ambiguous bases were removed; and (iii) sequences with overlaps longer than 10 bp were merged on the basis of their overlap sequences.
Operational taxonomic units (OTUs) were clustered with 97% similarity cut-off using UPARSE (version 7.1, http://drive5.com/uparse/). Chimeric sequences were identified and removed using UCHIME. The taxonomy of each 16S rRNA gene sequence was analysed using the RDP classifier algorithm (http://rdp.cme.msu.edu/) against the Silva (SSU123) 16S rRNA database with a confidence threshold of 70%.
Statistical analysis
Data were analysed using one-way ANOVA on SPSS 22.0 (SPSS Inc., Chicago, IL, USA). The significance of the mean differences among the groups was identified using the Tukey test. Data significance was determined at p<.05. Alpha diversity and rarefaction curve analyses consisting of community diversity (Simpson and Shannon indices) and richness indices (Sobs, Chao and ACE) were performed using mothur that is based on summary single command. Beta diversity analysis was performed to investigate the diversity among the samples. Principal component analysis (PCA) was calculated using mothur to describe the distances among the samples, and permutational multivariate ANOVA (PERMANOVA) was used to conduct statistically significant analysis on the OTU level. Welch’s t-test was used to determine the significant differences in terms of bacterial relative abundance at the phylum, family and genus levels between the LL and the control group.
Results
Egg production
Table shows the laying performance of hens. Compared with the control group, groups I and II exhibited significantly increased laying rate and decreased feed/egg ratio (p < 0.05), respectively. However, the differences in average daily egg weight, daily feed intake, broken egg rate and mortality among the three groups were insignificant (p > 0.05).
Table 2. Effects of LL* supplemental on laying performance of laying hens.◊.
Egg quality
Table shows the egg quality of hens. The differences in egg albumen height, Haugh units, egg shape index, eggshell breaking strength and eggshell thickness among the three groups were insignificant.
Table 3. Effects of LL* supplemental on egg quality of laying hens ◊.
Caecal microbiota
A total of 383,098 DNA sequence reads with an average length of 416 bp were generated from all samples. The average number of sequence reads per sample was 47,887 (minimum of 37,571 and maximum of 57,048). The sequences were further clustered into 781 OTUs by using a 97% similarity cut off. The rarefaction curves generated from the OTU suggested that a high sampling coverage (∼99%) was achieved in all samples (Supplementary Figure S1). Supplementary Table S1 shows the Alpha diversity indices of the caecal microbiota. The average values of microbial diversity indices in the LL group were lower than those in the control group.
Figure 1. Principal Component Analysis (PCA) of the community membership using Bray-Curtis distance. CON, control group; LL, group II. The abscissa and ordinate represent the two selected principal components, and the percentage represents the contribution of the principal component to the difference in sample composition. Points of different colours and shapes represent samples of different groups, and the closer the two sample points are, the more similar the composition of the two samples species is.
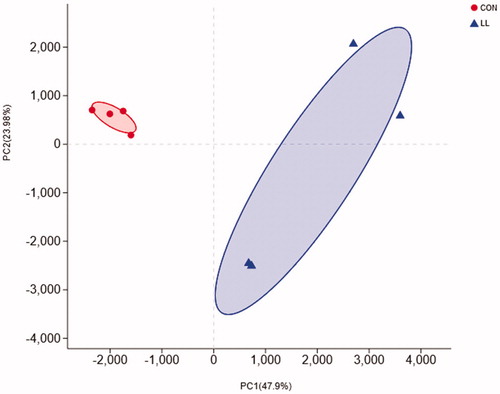
PCA on the OTU level showed the changes in the community structure (Figure ). The microbial community of the LL group was clearly separated from that of the control group. The results revealed a significant difference in bacterial structure in the caecum between LL and control groups (PERMANOVA, p = .031).
Figure and Supplementary Table S2 demonstrate the phylum distributions of the microbial composition. The predominant phyla in the LL and control groups were Firmicutes, Bacteroidetes and Proteobacteriain. The relative abundance of Bacteroidetes and Saccharibacteria was significantly increased and that of Firmicutes and Verrucomicrobia was significantly decreased in the LL group compared with those in the control group (p < .05).
Figure 2. Welch’s t-test bar plot on Phylum level. CON, control group; LL, group II. The ordinate (left) represents the phyla name and the ordinate (right) represents the p-Value.
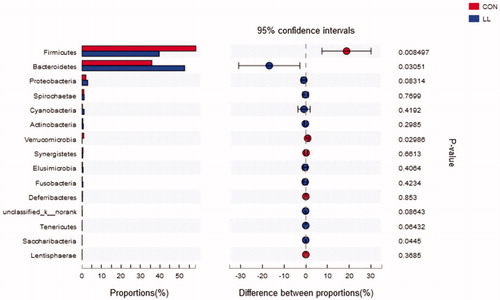
The family distribution results of the microbial composition are shown in Figure and Supplementary Table S3. The main families of the LL and control groups were Ruminococcaceae, Rikenellaceae, Bacteroidaceae and Lachnospiraceae, with Ruminococcac eae as the most abundant one. The average proportion of Ruminococcaceae was significantly decreased and that of Rikenellaceae was significantly increased in the LL group compared with those in the control group (p<.05). In addition, several families with small relative abundance (Acidaminoco ccaceae, unclassified_Bacteroidales, S24-7 group and ODP1230B8.23) in the LL group changed significantly compared with those in the control group (p<.05).
Figure 3. Welch’s t-test bar plot on Family level. CON, control group; LL, group II. The ordinate (left) represents the family name and the ordinate (right) represents the p-Value.
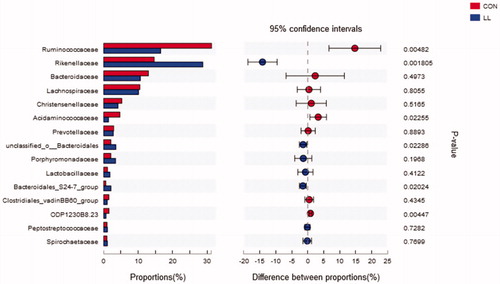
The microbial composition was also comparable at the genus level (Figure and Supplementary Table S4). The main genera of LL and the control groups were RC9 gut group and Bacteroides. Between these genera, the relative abundance of the RC9 gut group was significantly increased in the LL group compared with those in the control group (p<.05). Dietary with LL also significantly effected the average proportion of some low relative abundance genus (Phascolarctobacterium, unclassified_Bacteroidales, Butyricicoccus, norank_f__Rumi nococcaceae, Ruminococcaceae_UCG-014, coprostanoligenes group and Ruminococcaceae_UCG-005) compared with the control group (p<.05).
Discussion
Effects of LL on egg production
Herbs possess beneficial effects on growth performance, antioxidation as well as nutrient and energy utilisation for animals (Wenk Citation2003). The dietary supplement of LL can significantly increase the egg production rate (Ma et al. Citation2005), and decrease the mortality rate of laying hens (Li et al. Citation2017). Similar to previous research, our results demonstrated that the dietary inclusion of 1% and 2% LL significantly increased the egg production rate and decreased the feed/egg ratio of hens in late laying period. These changes may be related to the positive effects in the biological activity of bioactive components, such as oleanolic acid, ursolic acid and flavonoids present in LL, which can improve the efficiency and utilisation of nutrients, thereby enhance the productive performance (Gao et al. Citation2009).
Effects of LL on egg quality
Eggs are an excellent source of many essential nutrients. Egg quality is still a topic of concern in the poultry industry and for consumers worldwide. In the present study, the differences of egg quality among the three groups were insignificant.
Effects of LL on caecal microbiota
Chicken’s intestinal tract microbiota is important for their health, nutrient absorption and immune system. Some authors suggested that almost 10% of energy needs is recovered from a well-functioning caecum (Hegde et al. Citation1982; Józefiak et al. Citation2004).
The alpha diversity of microbial communities was measured using the indices of Sobs, Shannon, Simpson, Ace and Chao. The Shannon and Simpson indices represent how much of the differences are among the abundance of different taxa (diversity), whereas the Sobs, Chao and Ace indices reflect the number of the different taxa present in the sample (richness). Our study showed significant differences in the caecal microbiota diversity between the LL and the control groups. Combined with the result of the microbiota composition, the differences of the microbial structure may be due to the increase relative abundance of Bacteroidetes and the concurrent decrease of Firmicutes at the phylum level in the LL group. The results revealed the significantly lower average values of microbial diversity and richness in the LL group compared with those in the control group. These results indicated that dietary supplementation with LL seemed to reduce the bacterial species in the chicken caecal gut. The specific effect mechanism is still unclear.
Consistent with previous studies (Pan and Yu Citation2014; Zheng et al. Citation2019), our results also demonstrated that the caecal microbial communicates were dominated by Firmicutes, Bacteroidetes and Proteobacteria in chickens, which made up > 90% of the total sequences. Meanwhile, the higher relative abundance of Bacteroidetes and the lower Firmicutes than that of the control group were observed in the LL group. Bacteroides could digest complex carbohydrates and maintain an intestinal micro-ecological balance (Hooper Citation2004; Spence et al. Citation2006). Bacteroides could promote the development of the immune system (Sears Citation2005). Bacteroidetes is related to the development of interleukin-17-producing T-helper cells (Mazmanian et al. Citation2005). Firmicutes comprises a lot of genera of outstanding relevance in health care such as Staphylococcus, Listeria, and lactic acid bacteria (Lanza et al. Citation2015) and can obtain more energy from fibrous feed to satisfy the requirement of animal growth (Brulc et al. Citation2009). In the present study, we also observed significant differences in the relative abundance of Verrucomicrobia and Saccharibacteria between the LL and the control group, but the function of these phyla in the hen’s caecum was unclear and needed further investigations.
At the family level, the relative abundance of Rikenellaceae significantly increased in the LL group which may have partially contributed to the increased Bacteroidetes relative abundance in the LL group. Rikenellaceae is a relatively new taxonomic classification that belongs to the order Bacteroidales, with only three genera currently described belonging to this family (Graf Citation2014). Some bacteria in Rikenellaceae can produce acetate (Su et al. Citation2014), thereby suggesting a high level of functional redundancy for this metabolite in the animal gut microbiome. The high abundance of Rikenellaceae in the LL group may be due to the increase in the proportion of the RC9 gut group which belongs to Rikenellaceae in the LL group at the genus level. Ruminococcaceae family is a major population of phylum Firmicutes, the lower relative abundance of Ruminococcaceae may cause a decrease of Firmicutes in the LL group. Ruminococcaceae can produce short-chain fatty acids and degrade diverse polysaccharides and fibres in the human gut (Hooda et al. Citation2012). Except of the unclassified bacteria, the relative abundance of Acidaminococcaceae, Bacteroidales_S24-7_group and ODP1230B8.23 also changed significantly in the LL group compared with that in the control group at family level. However, further studies are needed to investigate the function of these families in the chicken’s gut.
Similarly, as reflected in the phylum and family, our results showed considerable differences in genus composition. The main genera of the LL and the control groups were RC9 gut group and Bacteroides. A large proportion of Firmicutes are composed of the genus Clostridium in chicken’s caecum (Pan and Yu Citation2014). However, in our study, Firmicutes was predominated by sequences belonging to Faecalibacterium and Phascolarctobacterium genus. Many factors can contribute to these differences. These factors include different chicken types, ages, diets and environmental factors. For example, the abundance of Clostridium increases and the proportion of lactobacilli decreases as the chicken aged (Shang et al. Citation2018). Different diet ingredients can also affect the relative abundance of Firmicutes and Bacteroidetes in chickens (Kumar et al. Citation2018). Cage type can also influence the composition of ileal and caecal microbiota of laying hens (Nordentoft et al. Citation2011).Genotype and gender can affect some microbiome species in the chicken gut (Zhao et al. Citation2013). These complexities make comparison with other studies difficult.
Sequences related to the genera Butyricicoccus were enriched in the caecum of hens in the LL group. The species of Butyricicoccus genera include Butyricicoccus pullicaecorum which is a main butyrate-producing strain in the intestine (Eeckhaut et al. Citation2008). Butyrate mediates beneficial effects on the growth performance and intestinal integrity of chickens (Mountzouris et al. Citation2010; Ahmed et al. Citation2014). Butyrate can also induce the synthesis of a subset of host defense peptides (cathelicidins) in jejunal and caecal explants from the chicken origin, with a natural broad antimicrobial spectrum that is important as the first line of defense (Sunkara et al. Citation2011). The relative abundance of Phascolarctobacterium, Ruminococcaceae_UCG-014 and Ruminococcaceae_UCG-005 were all significantly decreased in the LL group. The decrease of Ruminococcaceae_UCG-014 and Ruminococcaceae_UCG-005 might explain the decreased average proportion of Ruminococcaceae family. These results might be detrimental for hens because Phascolarctobacterium is an acetate/propionate-producer (Wu et al. Citation2017), and Ruminococcaceae_UCG-014 is positively related to butyrate concentrations (Han et al. Citation2018). However, information about the RC9 gut group, norank_f__Ruminococcaceae and coprostanoligenes group is lacking. Therefore, evaluating the possible effect of the changes in these genera on the chicken’s caecum microbial community is difficult. To the best of our knowledge, the effects of dietary supplementation with LL on the hen’s caecal microbiota have not been reported yet. Therefore, the cause and effect relationships driving these changes in the present study still need to be determined. However, since herbs can influence selectively the microorganisms by an anti-microbial activity or by a favourable stimulation of the aerobiosis of the microflora (Wenk Citation2003), LL may have also acted in this way in the present research. The exact reason for these results needs to be further studied.
Conclusions
The results indicated that dietary supplementation with 1% and 2% LL could improve egg production rate and significantly decrease feed/egg ratio compared with unsupplemented hens, respectively. Dietary supplementation with 2% LL can significantly alter the caecal microbiota composition with the changes in the phylum, family and genus levels. Therefore, 2% LL was the more appropriate level in this experiment. These findings provide an insight into hen’s egg production and gut microbiota modulations in response to different LL dosages and emphasise the requirement for additional research to determine the effects of LL on the chicken gut microbiota to predict the response of the microbiota to these agents.
Ethical approval
This research did not involve the introduction of any intervention on hens. The data collcetion was obtained with humanly handled, which according of animal care and welfare standard of The People's Republic of China. Animal experimentation procedures were approved by the Institutional Animal Care and Use Committee of Henan University of Science and Technology, Luoyang, China.
Supplemental Material
Download MS Word (80.1 KB)Disclosure statement
No potential conflict of interests was reported by the authors
Additional information
Funding
References
- Ahmed ST, Islam MM, Mun H-S, Sim H-J, Kim Y-J, Yang C-J. 2014. Effects of Bacillus amyloliquefaciens as a probiotic strain on growth performance, cecal microflora, and fecal noxious gas emissions of broiler chickens. Poultr Sci. 93(8):1963–1971.
- Ayeleso TB, Matumba MG, Mukwevho E. 2017. Oleanolic acid and its derivatives: Biological activities and therapeutic potential in chronic diseases. Molecules. 22(11):1915.
- Brulc JM, Antonopoulos DA, Miller ME, Wilson MK, Yannarell AC, Dinsdale EA, Edwards RE, Frank ED, Emerson JB, Wacklin P, et al. 2009. Gene-centric metagenomics of the fiber-adherent bovine rumen microbiome reveals forage specific glycoside hydrolases. Proc Natl Acad Sci USA. 106(6):1948–1953.
- Chen P, Wang AQ, Shan AS. 2009. Effects of Ligustrum lucidum fruits on growth performance, antioxidation and meat quality in arbor acres broilers. Asian Australas J Anim Sci. 22(5):700–705. English.
- Eeckhaut V, Van Immerseel F, Teirlynck E, Pasmans F, Fievez V, Snauwaert C, Haesebrouck F, Ducatelle R, Louis P, Vandamme P. 2008. Butyricicoccus pullicaecorum gen. nov., sp. nov., an anaerobic, butyrate-producing bacterium isolated from the caecal content of a broiler chicken. Int J Syst Evol Microbiol. 58(Pt 12):2799–2802.
- Gao D, Li Q, Li Y, Liu Z, Fan Y, Liu Z, Zhao H, Li J, Han Z. 2009. Antidiabetic and antioxidant effects of oleanolic acid from Ligustrum lucidum Ait in alloxan-induced diabetic rats. Phytother Res. 23(9):1257–1262.
- Graf J. 2014. The family rikenellaceae. In: Eugene R, Stephen DEFL, Erko S, Fabiano T, editors. The prokaryotes: Other major lineages of bacteria and the archaea. Berlin Heidelberg: Springer-Verlag; p. 857–859.
- Han K, Jin W, Mao Z, Dong S, Zhang Q, Yang Y, Chen B, Wu H, Zeng M. 2018. Microbiome and butyrate production are altered in the gut of rats fed a glycated fish protein diet. J Funct Foods. 47:423–433.
- Hegde SN, Rolls BA, Coates ME. 1982. THe effects of the gut microflora and dietary fibre on energy utilization by the chick. Br J Nutr. 48(1):73–80.
- Hooda S, Boler BM, Serao MC, Brulc JM, Staeger MA, Boileau TW, Dowd SE, Fahey GC, Jr., Swanson KS. 2012. 454 pyrosequencing reveals a shift in fecal microbiota of healthy adult men consuming polydextrose or soluble corn fiber. J Nutr. 142(7):1259–1265.
- Hooper LV. 2004. Bacterial contributions to mammalian gut development. Trends Microbiol. 12(3):129–134.
- Hu B, Du Q, Deng S, An HM, Pan CF, Shen KP, Xu L, Wei MM, Wang SS. 2014. Ligustrum lucidum Ait. fruit extract induces apoptosis and cell senescence in human hepatocellular carcinoma cells through upregulation of p21. Oncol Rep. 32(3):1037–1042.
- Huang X, Wang W. 2011. Chemical constituents of Ligustrum lucidum fruit: Research advances. J Int Pharm Res. 38(4751):6.
- Huang XJ, Ying W, Yin ZQ, Ye WC. 2010. Two new dimeric secoiridoid glycosides from the fruits of Ligustrum lucidum. J Asian Nat Prod Res. 12(8):685–690.
- Józefiak D, Rutkowski A, Martin SA. 2004. Carbohydrate fermentation in the avian ceca: a review. Animal Feed Sci Technol. 113(1-4):1–15.
- Kumar S, Chen C, Indugu N, Werlang GO, Singh M, Kim WK, Thippareddi H. 2018. Effect of antibiotic withdrawal in feed on chicken gut microbial dynamics, immunity, growth performance and prevalence of foodborne pathogens. PLoS One. 13(2):e0192450.
- Lanza VF, Tedim AP, Martinez JL, Baquero F, Coque TM. 2015. The plasmidome of firmicutes: impact on the emergence and the spread of resistance to antimicrobials. Microbiol Spectr. 3(2):PLAS-0039-2014.
- Li Q, Fan YS, Gao ZQ, Fan K, Liu ZJ. 2015. Effect of Fructus Ligustri Lucidi on osteoblastic like cell-line MC3T3-E1. J Ethnopharmacol. 170:88–95.
- Li XL, He WL, Yang ML, Yan YM, Xue YH, Zhao ST. 2017. Effect of dietary supplementation of Ligustrum lucidum on performance, egg quality and blood biochemical parameters of Hy-Line Brown hens during the late laying period. Animal. 11(11):1899–1904.
- Liu J. 2005. Oleanolic acid and ursolic acid: research perspectives. J Ethnopharmacol. 100(1-2):92–94.
- Liu Q, Kim SH, Kim SB, Jo YH, Kim ES, Hwang BY, Oh K, Lee MK. 2014. Anti-obesity effect of (8-E)-niizhenide, a secoiridoid from Ligustrum lucidum, in high-fat diet-induced obese mice. Nat Prod Commun. 9(10):1399–1401.
- Ma D, Liu Y, Liu S, Liu Q, Shan A. 2007. Influence of Ligustrum lucidum and Schisandra chinensis fruits on antioxidative metabolism and immunological parameters of layer chicks. Asian Australas J Anim Sci. 20(9):1438–1443.
- Ma D, Shan A, Chen Z, Du J, Song K, Li J, Xu Q. 2005. Effect of Ligustrum lucidum and Schisandra chinensis on the egg production, antioxidant status and immunity of laying hens during heat stress. Arch Anim Nutr. 59(6):439–447.
- Mazmanian SK, Liu CH, Tzianabos AO, Kasper DL. 2005. An immunomodulatory molecule of symbiotic bacteria directs maturation of the host immune system. Cell. 122(1):107–118.
- Mohammadi Gheisar M, Kim IH. 2018. Phytobiotics in poultry and swine nutrition–a review. Ital J Anim Sci. 17(1):92–99.
- Mountzouris K, Tsitrsikos P, Palamidi I, Arvaniti A, Mohnl M, Schatzmayr G, Fegeros K. 2010. Effects of probiotic inclusion levels in broiler nutrition on growth performance, nutrient digestibility, plasma immunoglobulins, and cecal microflora composition. Poultr Sci. 89(1):58–67.
- Nordentoft S, Molbak L, Bjerrum L, De Vylder J, Van Immerseel F, Pedersen K. 2011. The influence of the cage system and colonisation of Salmonella Enteritidis on the microbial gut flora of laying hens studied by T-RFLP and 454 pyrosequencing. BMC Microbiol. 11:187.
- Pan D, Yu Z. 2014. Intestinal microbiome of poultry and its interaction with host and diet. Gut Microbes. 5(1):108–119.
- Sears CL. 2005. A dynamic partnership: celebrating our gut flora. Anaerobe. 11(5):247–251.
- Shang Y, Kumar S, Oakley B, Kim WK. 2018. Chicken gut microbiota: importance and detection technology. Front Vet Sci. 5:254
- Spence C, Wells WG, Smith CJ. 2006. Characterization of the primary starch utilization operon in the obligate anaerobe Bacteroides fragilis: Regulation by carbon source and oxygen. J Bacteriol. 188(13):4663–4672.
- Su XL, Tian Q, Zhang J, Yuan XZ, Shi XS, Guo RB, Qiu YL. 2014. Acetobacteroides hydrogenigenes gen. nov., sp. nov., an anaerobic hydrogen-producing bacterium in the family Rikenellaceae isolated from a reed swamp. Int J Syst Evol Microbiol. 64(Pt 9):2986–2991.
- Sunkara LT, Achanta M, Schreiber NB, Bommineni YR, Dai G, Jiang W, Lamont S, Lillehoj HS, Beker A, Teeter RG, et al. 2011. Butyrate enhances disease resistance of chickens by inducing antimicrobial host defense peptide gene expression. PLoS One. 6(11):e27225.
- Wenk C. 2003. Herbs and botanicals as feed additives in monogastric animals. Asian Australas J Anim Sci. 16(2):282–289.
- Wu F, Guo X, Zhang J, Zhang M, Ou Z, Peng Y. 2017. Phascolarctobacterium faecium abundant colonization in human gastrointestinal tract. Exp Therapeutic Med. 14(4):3122–3126.
- Zhang C, Wang JL, Liu TY, Shan AS. 2012. The effects of Ligustrum lucidum extract on growth performance and meat quality in growing-finishing pigs. J Anim Veterinary Adv. 11(18):3342–3345.
- Zhang CH, Zhang MH, Wang SY, Han RJ, Cao YF, Hua WY, Mao YJ, Zhang XJ, Pang XY, Wei CC, et al. 2010. Interactions between gut microbiota, host genetics and diet relevant to development of metabolic syndromes in mice. Isme J. 4(2):232–241.
- Zhao L, Wang G, Siegel P, He C, Wang H, Zhao W, Zhai Z, Tian F, Zhao J, Zhang H, et al. 2013. Quantitative genetic background of the host influences gut microbiomes in chickens. Sci Rep. 3:1163.
- Zheng M, Mao P, Tian X, Meng L. 2019. Growth performance, carcass characteristics, meat and egg quality, and intestinal microbiota in Beijing-you chicken on diets with inclusion of fresh chicory forage. Ital J Anim Sci. 18(1):1310–1320.