Abstract
An experiment was conducted to investigate the effects of citric acid residue (CAR) and lactic acid bacteria (LAB) on fermentation quality and aerobic stability of alfalfa silage. Alfalfa was treated as follows: (1) control without additive (CON); (2) LAB (L); (3) 12 g/kg CAR (C); (4) 12 g/kg CAR + LAB (CL) and fermented for 3, 6, 9, 15, 30 and 45 days. The residual silages ensiled for 45 days were evaluated for aerobic stability. The results showed that addition of CAR with or without LAB inoculant decreased pH, acetic acid, ammonia nitrogen , neutral detergent fibre, hemicellulose contents and Enterobacteriaceae counts (p < .05) and increased dry matter and lactic acid contents (p < .05) after 45 days of ensiling. Whereas, the lower lactic acid and higher acetic acid contents were observed in L silages (p < .05) after 45 days of ensiling. During aerobic exposure, L and CL silages remained stable in pH, lactic and acetic acid contents, while the control and C silages showed higher pH, yeast and mould counts and lower lactic and acetic acid contents. Compared with the control, L and CL improved aerobic stability, whereas the poorer aerobic stability was shown in C silages (p < .05). In conclusion, applying a combination of CAR and LAB inoculant improved both fermentation quality and aerobic stability of alfalfa silage.
Citric acid residue (CAR) was compared with a lactic acid bacteria (LAB) inoculant in ensiling alfalfa.
The CAR improved fermentation quality compared to control and LAB treated silages.
Aerobic stability improved when CAR was used in combination with LAB but not when used alone.
Highlights
Introduction
Ensiling is a traditional preservation method of forage, and it is based on spontaneous lactic acid fermentation by epiphytic lactic acid bacteria (LAB) under anaerobic conditions (Weinberg and Muck Citation1996; Zhang Q et al. Citation2017). The fermentation quality varies with the chemical compositions and physical structure of forage (McDonald et al. Citation1991). Some forages such as alfalfa, which have low water-soluble carbohydrate (WSC) content and high buffering capacity, are difficult to make quality silage by natural fermentation (McDonald et al. Citation1991; Marshall et al. Citation1993). Thus, chemical additives and microbial inoculants were widely used in silage making to improve fermentation quality.
Organic acid additives based on direct acidification and antimicrobial effects have been shown to be effective in improving silage quality (Koivunen et al. Citation2015; Muck et al. Citation2018). Citric acid is also an important organic acid, and it has been used in food preparation due to its safety (Shen et al. Citation2019). Because of the antimicrobial property of citric acid, it is also used as a silage additive. Previous studies have shown that application of citric acid in alfalfa silage decreased the pH value, limited proteolysis and improved fermentation quality (Ke et al. Citation2017, Citation2018). In addition, Wang et al. (Citation2009) found citric acid increased feed digestion and utilisation in the diet of steer. However, the relatively high costs of citric acid limit its application opportunities in silage making. Citric acid residue (CAR) is the main by-product of citric acid production, and it contains some citric acid, crude protein and other nutrients. In the recent years, large amounts of CAR were generated from citric acid production because of the development of citric acid industry (Zhang et al. Citation2014; Xu et al. Citation2016). However, the disposition of such a large amount of CAR has become a thorny problem, which might pollute the environment and waste resources. Considering the positive effects of citric acid in improvement of silage quality, CAR might become an attractive alternative to other chemical additives. Nevertheless, little information is available on the alfalfa silage treated with CAR, and its true function in silage making is also unclear. It is known that LAB inoculant is a common fermentation stimulant in silage making. A combination of heterofermentative and homofermentative LAB was considered to ensure both anaerobic lactic fermentation and to improve aerobic stability. For all these reasons, both of CAR and LAB addition in silage making might ensure adequate fermentation.
The objective of the experiment was to investigate the effects of adding CAR alone or combined with LAB inoculant on the fermentation quality and aerobic stability of alfalfa silage.
Materials and methods
Silage preparation
Alfalfa (Medicago sativa L.) was cultivated in an experimental field of Nanjing Agricultural University (32.04°N, 118.88°E, altitude 17 m above sea level, Jiangsu, China). Alfalfa was harvested at the early bloom stage leaving a stubble of about 5 cm on 25 November 2018. The fresh alfalfa was taken into the laboratory and chopped into a theoretical length of 2–3 cm with a fodder chopper. The LAB inoculant and CAR were used as additives. The LAB inoculant was a combination of Lactobacillus plantarum MTD-1 (Ecosyl Products Ltd., Stokesley, UK) and Lactobacillus buchneri 40788 (Lallemand Animal Nutrition, Milwaukee, WI) (applied at a ratio of 1:1). The LAB inoculant was applied at a level of 1 × 106 cfu/g of fresh weight (FW). The CAR used in the experiment was an acidic and dark brown liquid with 440 g/kg dry matter (DM) (Jiangsu Guoxin Union Energy Co., Ltd., Yixing, China). The CAR contained 136 g/kg citric acid, 261 g/kg crude protein, 619 g/kg neutral detergent fibre (NDF), 27.3 g/kg ether extract and 18.2 g/kg crude ash on a DM basis. The chopped alfalfa was treated as follows: (1) control without additive (CON); (2) LAB inoculant (L); (3) CAR applied at 12.0 g/kg of FW (C); (4) CAR (12.0 g/kg of FW) + LAB inoculant (CL). All ingredients of each treatment were mixed homogeneously and packed into 5 L laboratory silos (polyethylene bottle with diameter of 17.3 cm and height of 26.5 cm, Lantian Biological Experimental Instrument Co., Ltd., Jiangsu, China). The silos were stored at ambient temperature (16–21 °C) after being sealed with screw tops and plastic tape. Five silo replicates of each treatment were opened after 3, 6, 9, 15, 30 and 45 days of ensiling for subsequent analyses.
Aerobic stability
After ensiling for 45 days, the residual silages of each silo were placed loosely in a 5 L plastic bucket without sealing to test their aerobic stability. Each bucket was covered with a layer of cheesecloth to avoid contamination. Ten bucket replicates were prepared for each treatment, among which 5 buckets were used for temperature measurement, and the left 5 buckets were used for chemical and microbial analysis. Thermocouple wires were placed at the centre of the silage mass and temperatures were recorded every 30 min using a data logger (SMOWO MDL-1048A; Tianhe Automation Instrument Co., Ltd, Shanghai, China). When the temperature of the silage increased by 2 °C above the ambient temperature, the silage was recognised as undergoing aerobic deterioration. Approximately 200 g air-exposed silage was removed from each plastic bucket of each treatment at 2, 4 and 6 days to quantify the changes of chemical and microbial compositions.
Chemical and microbial analysis
The silage from each silo was emptied and placed into a clean plastic container and mixed to uniformity. The dry matter (DM) contents of fresh alfalfa and silages were determined by a forced draft oven to a constant weight at 65 °C for 3 days. The dried samples were ground to pass a 1 mm screen by a laboratory knife mill (FW100, Taisite Instrument Co., Ltd., Tianjin, China). The ground sample was analysed for water-soluble carbohydrates (WSC), total nitrogen (TN), neutral detergent fibre (NDF), acid detergent fibre (ADF) and acid detergent lignin (ADL). The WSC was analysed by colorimetry after reaction with anthrone reagent (Arthur Thomas Citation1977). The TN was determined by a Kjeldahl nitrogen analyser (Kjeltec 8200; FOSS, Sweden), and crude protein (CP) was calculated as TN × 6.25. The NDF, ADF and ADL contents were measured by the method of Van Soest et al. (Citation1991), using the ANKOM filter bag technique with an ANKOM 200i fibre analyser (ANKOM Technologies, Inc., Fairport, NY, USA). Hemicellulose was calculated as NDF minus ADF, and cellulose as ADF minus ADL.
For determination of pH and organic acid, 60 g sample was diluted with 120 mL distilled water and stored in the refrigerator at 4 °C for 24 h, followed by filtration through two layers of cheesecloth and Whatman filter paper (pore size of 11 μm, Xinhua Co, China). The filtrate pH was measured immediately with a pH metre (HANNA pH 211; Hanna Instruments Italia Srl, Villafranca Padovana, Italy). The filtrate stored at −20 °C for subsequent determination of ammonia nitrogen (NH3-N) and organic acid. The filtrate was centrifuged for 10 min at 4 °C, 10 000 × g and filtrated through a microfilter (0.22 μm) for organic acid determination, and then carried out using Agilent 1260 HPLC system (Agilent Technologies, Inc., Waldbronn, Germany) equipped with a refractive index detector (column: Carbomix® H-NP5; Sepax Technologies, Inc., Newark, DE, USA; eluent: 2.5 mmol/L H2SO4, 0.5 mL/min; temperature: 55 °C). The NH3-N was determined by the phenol–hypochlorite reaction (Broderick and Kang Citation1980). The buffering capacity of the fresh alfalfa was determined according to the method described by Playne and McDonald (Citation1966).
Approximately 10 g of fresh alfalfa or silages were tenfold serially diluted with sterilised saline solution (0.85% sodium chloride). The LAB was enumerated on deMan, Rogosa and Sharp agar medium after incubation in an anaerobic incubator at 37 °C for 2 days. Enterobacteriaceae was counted on the Violet Red Bile Glucose Agar medium after 24 h of incubation at 37 °C under aerobic conditions. Yeasts and moulds were enumerated on potato dextrose agar with 0.25% chloramphenicol (Sincere Biotech Co., Ltd., Shanghai, China) after incubation at 30 °C for 3 days.
Statistical analysis
The fermentation characteristics and microbial composition during ensiling were analysed by a two-way analysis of variance to evaluate the effects of treatment, ensiling days and their interactions. Effects of additives on the fibre composition and aerobic stability after 45 days of ensiling were subjected to one-way analysis. The chemical and microbial composition during air exposure was analysed by repeated measures analysis of variance to evaluate the effects of additive treatments, days of air exposure and their interactions. Data were analysed using the General Linear Model (GLM) procedure of SPSS.22 software. The different sample means were compared for significance by Duncan’s multiple range method and significance was declared at p < .05. The microbial data were converted to log10.
Results
Chemical and microbial compositions of fresh alfalfa
The characteristics of fresh alfalfa are shown in Table . Alfalfa had low DM (205.30 g/kg FW) and high CP (230 g/kg DM) contents. The WSC, NDF and ADF contents were 63.7, 402 and 275 g/kg DM, respectively. The buffering capacity was 215 mEq/kg DM. The counts of LAB, Enterobacteriaceae, yeast and mould in fresh alfalfa were all above 1 × 106 cfu/g FW.
Table 1. Chemical and microbial composition of fresh alfalfa.
Fermentation characteristics of alfalfa during ensiling
The changes of pH, DM, NH3-N, WSC contents and LAB counts of alfalfa during ensiling are shown in Table . The pH, NH3-N, WSC contents and LAB and Enterobacteriaceae counts were significantly affected by treatments, ensiling days and their interactions (p < .05), and the DM contents were significantly affected by treatments (p < .05). All additives increased the DM contents and decreased pH value as compared to the control during ensiling. The NH3-N contents increased and the WSC contents decreased in all silages with the progression of ensiling. The NH3-N contents in C and CL silages decreased compared to silage without CAR during ensiling. The WSC content in C silages was higher than that of the control during ensiling. There was no difference in WSC content between L and the control silages. The counts of LAB in all silages increased first and then decreased during ensiling, and addition of CAR had no significant effects on the number of LAB. Compared with the control, all additives decreased Enterobacteriaceae counts during ensiling.
Table 2. Effect of additives on pH value, dry matter, ammonia nitrogen, water soluble carbohydrate contents and microbial compositions of alfalfa during ensiling.
As shown in Table , treatments, ensiling days and their interactions had significant effects on lactic acid/acetic acid (LA/AA), lactic acid, acetic acid, propionic acid and butyric acid contents (p < .05). The lactic and acetic acid contents in all silages increased during ensiling. After 45 days of ensiling, the lactic acid content in C and CL silages were higher than that of the control. The acetic acid content in C and CL silages were lower than that of the control silages during ensiling. At the end of ensiling, L silages were observed the highest acetic acid content among all silages. The LA/AA ratios in CL silage were greater than that of the control. Inoculation with LAB increased propionic acid content as compared to the control. The contents of butyric acid in all additive silages maintained below 2.0 g/kg DM during ensiling.
Table 3. Effect of additives on fermentation acids of alfalfa during ensiling.
The NDF, ADF, ADL, hemicellulose and cellulose contents are shown in Table . The CAR-treated silages showed lower NDF and hemicellulose contents than these of silages without CAR. The NDF, ADF, hemicellulose and cellulose contents in CL silages were lower than these of the control (p < .05). The C silages showed lower NDF and hemicellulose contents than these of the L silages.
Table 4. Effect of additives on structural carbohydrates composition and aerobic stability of alfalfa ensiled for 45 days.
Aerobic stability of alfalfa silages after 45 days of ensiling
As shown in Table , inoculation with LAB markedly increased aerobic stability as compared to the control and C silages (p < .05), whereas addition of CAR without LAB inoculant observably reduced aerobic stability compared with the control (p < .05). The CL silages showed the longest aerobic stable time among all silages.
The chemical and microbial compositions of alfalfa silage during exposure to air were presented in Figure . Treatments, days of air exposure and their interactions had significant effects on pH, NH3-N, lactic acid, acetic acid contents and the counts of yeast and mould (p < .001). All silages showed an increase in the pH and the counts of yeast and mould, and a decrease in the contents of WSC and lactic acid with the progression of exposure to air. The relatively low pH was observed in CL silages as compared to other silages during exposure to air. The C silages remained higher WSC content than that of other silages during exposure to air. The greatest acetic acid content was observed in L silages during exposure to air. Compared with the control, L silages showed the lower yeast and mould counts, whereas the reverse result was observed in C silages during exposure to air.
Figure 1. The change of (a) pH, (b) water soluble carbohydrate, (c) ammonia nitrogen (NH3-N), (d) lactic acid, (e) acetic acid and (f) yeasts and moulds of alfalfa silages during aerobic exposure. FW: fresh weight; DM: dry matter; NH3-N: ammonia nitrogen; TN: total nitrogen; cfu: colony-forming units; CON: control; L: 1 × 106 log cfu/g lactic acid bacteria inoculant (Lactobacillus plantarum + Lactobacillus buchneri); C: 12 g/kg citric acid residue; CL: C + L. Data are presented as means of five replicates. T: effect of treatments; D: effect of days of air exposure; T × D: interaction between treatments and days of exposure (p<.05).
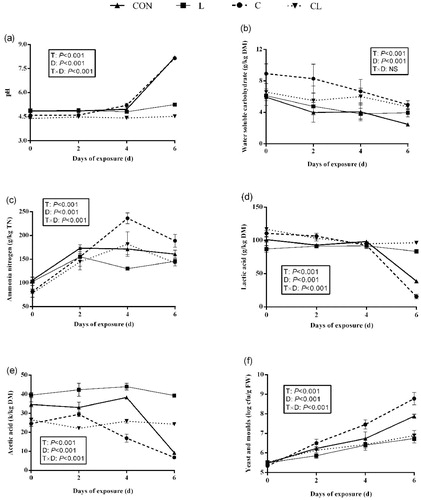
Discussion
Fermentation quality
Citric acid is well known to have antimicrobial activity and widely used as an antimicrobial agent in the food industry (Qiu et al. Citation2014). The antimicrobial activity is due to the undissociated form of citric acid, which can pass into the cell easily and acidify the cytoplasm (Brul and Coote Citation1999; Qiu et al. Citation2014). Thus, addition of CAR in silage might inhibit the activity of undesirable bacteria and hence improved fermentation quality. In the experiment, the higher DM contents were observed in C and CL silages. This is because CAR provided additional substrates, and the antimicrobial activity of citric acid inhibited the activity of undesirable bacteria resulting in a reduction of DM loss.
It is well known that plant proteases and microbial activity play important roles in proteolysis, and high levels of NH3-N indicate a high occurrence of protein degradation during ensiling (Ding et al. Citation2013; Tomaz et al. Citation2018). Addition of CAR decreased the NH3-N content compared with the silages without CAR, which might be attributed to the antimicrobial effect of CAR. A rapid drop in pH in CAR-treated silages can also inhibit proteolysis due to the inactivation of plant enzymes. After 45 days of ensiling, C silages showed the higher WSC content than that of other silages, which might be explained that CAR inhibited the activities of undesirable bacteria and reduced the WSC losses.
The counts of LAB in all silages increased first and then declined during ensiling. In general, the growth of LAB was inhibited by the low pH and fermentation products after it reached the peak numbers of around 10 log cfu/g FM during ensiling (Pahlow et al. Citation2003). The lower number of Enterobacteriaceae in C and CL silages indicated that CAR could inhibit the growth of enterobacteria, which also explained the higher DM contents and lower NH3-N in these silages. The reduction of Enterobacteriaceae counts was also observed in L silages during ensiling. In a similar study, Nascimento Agarussi et al. (Citation2019) reported that inoculation of alfalfa silage with Lactobacillus plantarum decreased the counts of enterobacteria.
The higher accumulation of lactic acid in C and CL silages at the end of ensiling resulted in the low pH in these silages. It is interesting to note that the similar pH and lactic acid content were observed in L silages as compared to the control. The results were similar to Meeske and Basson (Citation1998) who reported that inoculated maize with LAB had no effects on pH reduction and lactic acid production, which might be related to the presence of high numbers of epiphytic LAB on the fresh forage before ensiling. Furthermore, inoculation of silages with Lactobacillus buchneri often will be 0.1 to 0.2 pH units higher than the untreated silages due to the conversion of lactic acid to acetic acid, 1,2-propanediol, and ethanol (Oude Elferink et al. Citation2001; Kleinschmit and Kung Citation2006; Kung et al. Citation2018). Addition of CAR decreased the acetic acid content compared with the control during ensiling. It is probable that the antimicrobial activity of citric acid inhibited the growth of some undesirable bacteria like enterobacteria that could product acetic acid (McDonald et al. Citation1991; Sallam Citation2007). In addition, L silages showed lower acetic acid content than that of the control during the first 15 days of ensiling, while the inverse results were observed at the end of ensiling. The results could be explained that heterofermentative LAB dominated at the end of ensiling in most silages, which encouraged the production of acetic acid (McDonald et al. Citation1991). The LAB inoculant used in this study contained Lactobacillus buchneri, which is a common heterofermentative LAB. It is noted that the contents of lactic and acetic acid in all silages had large fluctuations after 30 days of ensiling, which indicated that the process of ensiling did not reach a plateau. Thus, prolonging the time of ensiling was worthy to be considered. The higher LA/AA ratios were observed in C and CL silages at the end of ensiling. These results indicated that CAR enhanced lactic acid fermentation, which was in agreement with Ke et al. (Citation2018) who found that application of citric acid with or without LAB inoculant in alfalfa silage increased lactic acid content. Inoculation with LAB increased propionic acid content as compared to the silages without inoculation. This might be explained that Lactobacillus buchneri converted lactic acid to 1,2-propanediol, and the latter could be further converted to propionic acid by Lactobacillus diolivorans (Krooneman et al. Citation2002).
Addition of CAR decreased NDF and hemicellulose contents, and the lower contents of NDF, ADF and cellulose were observed in CL silages as compared to the control, which might be related to the result of acid hydrolysis in the CAR-treated silages. These results could also explain the high WSC content was observed in C silages.
Aerobic stability
The aerobic stability of silage is a very important factor determining its subsequent nutritional quality and feeding value (Filya Citation2004). When silage is exposed to air during the feed-out period, aerobic spoilage microorganisms such as yeasts and moulds multiply, resulting in a rise of temperature and a deterioration of the silage (McDonald et al. Citation1991). In the present experiment, L and CL silages showed a longer period of aerobic stability compared with the silages without LAB inoculant. This is because Lactobacillus buchneri could produce acetic acid directly through herterolactic fermentation of carbohydrate, and acetic acid had the ability to inhibit the growth of yeasts thereby improving aerobic stability (McDonald et al. Citation1991). However, the poorer aerobic stability was observed in the C silages, which could be explained that some yeast strains could use citric acid as an available substrate (Carvalho et al. Citation2015), and yeasts are known as initiators of aerobic deterioration of silage (McDonald et al. Citation1991).
During exposure to air, the pH, lactic acid and acetic acid contents remained relatively stable in L and CL silages compared with the control and C silages, which indicated that inoculation with LAB is in favour of aerobic stability. The results also further demonstrated that the longer stable time of air exposure was observed in L and CL silages. The study of Li and Nishino (Citation2011) who reported that inoculation with Lactobacillus buchneri improved the fermentation quality and aerobic stability of wilted Italian ryegrass silage. After 4 days of exposure to air, the control and C silages showed a rapid increase in the pH and the counts of yeast and mould accompanied with a rapid reduction in the contents of lactic and acetic acid. Compared to the L and CL silages, the higher pH, yeast and mould counts and lower lactic acid and acetic acid contents were observed in the control and C silages after exposure for 6 days. However, the aerobic stability in C silages was poorer than that of the control. This result was mainly related to the high yeast and mould counts in C silages during exposure to air, which could be explained that the high residue WSC contents in these silages encouraged the growth of yeasts. Moreover, previous study reported that citric acid could enhance the growth of yeasts in alfalfa silage (Ke et al. Citation2018). However, it is interesting to note that silages treated with CAR plus LAB inoculant showed the low yeast and mould counts during exposure to air. This was probably because the low residue WSC contents in these silages could not supply adequate substrates for the growth of yeasts. Thus, addition of CAR combined with LAB inoculant could improve the aerobic stability of alfalfa silage.
Conclusions
Addition of CAR with or without LAB inoculant improved the fermentation quality of alfalfa silage, as indicated by the relatively high DM and lactic acid contents and low pH, acetic acid and NH3-H contents. Inoculation with LAB showed positive effects on aerobic stability of alfalfa silage, whereas application of CAR alone accelerated aerobic deterioration. In summary, addition of CAR with LAB inoculant improved both fermentation quality and aerobic stability of alfalfa silage.
Acknowledgements
The authors thank Jiangsu Guoxin Union Energy Co., Ltd. for their support in the study.
Disclosure statement
The authors have no conflict of interest to this work.
Additional information
Funding
References
- Arthur Thomas T. 1977. An automated procedure for the determination of soluble carbohydrates in herbage. J Sci Food Agric. 28:639–642.
- Broderick GA, Kang JH. 1980. Automated simultaneous determination of ammonia and total amino acids in ruminal fluid and in vitro media. J Dairy Sci. 63:64–75.
- Brul S, Coote P. 1999. Preservative agents in foods. Mode of action and microbial resistance mechanisms. Int J Food Microbiol. 50:1–17.
- Carvalho BF, Ávila CLS, Miguel M, Pinto JC, Santos MC, Schwan RF. 2015. Aerobic stability of sugarcane silage inoculated with tropical strains of lactic acid bacteria. Grass Forage Sci. 70:308–323.
- Ding WR, Long RJ, Guo XS. 2013. Effects of plant enzyme inactivation or sterilization on lipolysis and proteolysis in alfalfa silage. J Dairy Sci. 96:2536–2543.
- Filya I. 2004. Nutritive value and aerobic stability of whole crop maize silage harvested at four stages of maturity. Anim Feed Sci Technol. 116:141–150.
- Ke WC, Ding WR, Xu DM, Ding LM, Zhang P, Li FD, Guo XS. 2017. Effects of addition of malic or citric acids on fermentation quality and chemical characteristics of alfalfa silage. J Dairy Sci. 100:8958–8966.
- Ke WC, Ding WR, Xu DM, Shah MN, Zhang P, Guo XS. 2018. Influences of addition of malic acid or citric acid, Lactobacillus plantarum and their mixtures on fermentation quality, proteolysis and fatty acid composition of ensiled alfalfa. Arch Anim Nutr. 72:492–502.
- Kleinschmit DH, Kung L. Jr. 2006. A meta-analysis of the effects of Lactobacillus buchneri on the fermentation and aerobic stability of corn and grass and small-grain silages. J Dairy Sci. 89:4005–4013.
- Koivunen E, Jaakkola S, Heikkila T, Lampi AM, Halmemies-Beauchet-Filleau A, Lee MR, Winters AL, Shingfield KJ, Vanhatalo A. 2015. Effects of plant species, stage of maturity, and level of formic acid addition on lipolysis, lipid content, and fatty acid composition during ensiling. J Anim Sci. 93:4408–4423.
- Krooneman J, Faber F, Alderkamp AC, Elferink SJ, Driehuis F, Cleenwerck I, Swings J, Gottschal JC, Vancanneyt M. 2002. Lactobacillus diolivorans sp. nov., a 1,2-propanediol-degrading bacterium isolated from aerobically stable maize silage. Int J Syst Evol Microbiol. 52:639–646.
- Kung L Jr, Shaver RD, Grant RJ, Schmidt RJ. 2018. Silage review: interpretation of chemical, microbial, and organoleptic components of silages. J Dairy Sci. 101:4020–4033.
- Li Y, Nishino N. 2011. Bacterial and fungal communities of wilted Italian ryegrass silage inoculated with and without Lactobacillus rhamnosus or Lactobacillus buchneri. Lett Appl Microbiol. 52:314–321.
- Marshall SA, Campbell CP, Buchanan-Smith JG. 1993. Proteolysis and rumen degradability of alfalfa silages preserved with a microbial inoculant, spent sulfite liquor, formic acid or formaldehyde. Can J Anim Sci. 73:559–570.
- McDonald P, Henderson AR, Heron S. 1991. The biochemisty of silage. 2nd ed. Marlow (UK): Chalcombe Publications.
- Meeske R, Basson HM. 1998. The effect of a lactic acid bacterial inoculant on maize silage. Anim Feed Sci Technol. 70:239–247.
- Muck RE, Nadeau EMG, McAllister TA, Contreras-Govea FE, Santos MC, Kung L Jr. 2018. Silage review: recent advances and future uses of silage additives. J Dairy Sci. 101:3980–4000.
- Nascimento Agarussi MC, Gomes Pereira O, Paula RA, Silva VPD, Santos Roseira JP, Fonseca E. 2019. Novel lactic acid bacteria strains as inoculants on alfalfa silage fermentation. Sci Rep. 9:8007.
- Oude Elferink SJ, Krooneman J, Gottschal JC, Spoelstra SF, Faber F, Driehuis F. 2001. Anaerobic conversion of lactic acid to acetic acid and 1, 2-propanediol by Lactobacillus buchneri. Appl Environ Microbiol. 67:125–132.
- Pahlow G, Muck RE, Driehuis F, Elferink S. Spoelstra SF. 2003. Microbiology of ensiling. In: Buxton DR, Muck RE, Harrison JH, editors. Silage science and technology. Madison (WI): American Society of Agronomy, Crop Science Society of America, Soil Science Society of America; p. 31–93.
- Playne MJ, McDonald P. 1966. The buffering constituents of herbage and of silage. J Sci Food Agric. 17:264–268.
- Qiu X, Chen S, Liu G, Yang Q. 2014. Quality enhancement in the Japanese sea bass (Lateolabrax japonicas) fillets stored at 4 °C by chitosan coating incorporated with citric acid or licorice extract. Food Chem. 162:156–160.
- Sallam KI. 2007. Antimicrobial and antioxidant effects of sodium acetate, sodium lactate, and sodium citrate in refrigerated sliced salmon. Food Control. 18:566–575.
- Shen YZ, Ding LY, Chen LM, Xu JH, Zhao R, Yang WZ, Wang HR, Wang MZ. 2019. Feeding corn grain steeped in citric acid modulates rumen fermentation and inflammatory responses in dairy goats. Animal. 13:301–308.
- Tomaz PK, de Araujo LC, Sanches LA, dos Santos-Araujo SN, de Lima TO, Lino A. d A, Ferreira EM. 2018. Effect of sward height on the fermentability coefficient and chemical composition of Guinea grass silage. Grass Forage Sci. 73:588–598.
- Van Soest PJ, Robertson JB, Lewis BA. 1991. Methods for dietary fiber, neutral detergent fiber, and nonstarch polysaccharides in relation to animal nutrition. J Dairy Sci. 74:3583–3597.
- Wang C, Liu Q, Meng J, Yang WZ, Yang XM, He DC, Dong KH, Huang YX. 2009. Effects of citric acid supplementation on rumen fermentation, urinary excretion of purine derivatives and feed digestibility in steers. J Sci Food Agric. 89:2302–2307.
- Weinberg ZG, Muck RE. 1996. New trends and opportunities in the development and use of inoculants for silage. FEMS Microbiol Rev. 19:53–68.
- Xu J, Su XF, Bao JW, Zhang HJ, Zeng X, Tang L, Wang K, Zhang JH, Chen XS, Mao ZG. 2016. A novel cleaner production process of citric acid by recycling its treated wastewater. Bioresour Technol. 211:645–653.
- Zhang Q, Yu Z, Wang X, Na R. 2017. Effects of chlorpyrifos and chlorantraniliprole on fermentation quality of alfalfa (Medicago sativa L.) silage inoculated with or without Lactobacillus plantarum LP. Anim Sci J. 88:456–462.
- Zhang HJ, Zhang JH, Xu J, Tang L, Mao ZG. 2014. A novel recycling process using the treated citric acid wastewater as ingredients water for citric acid production. Biochem Engin J. 90:206–213.