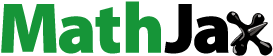
Abstract
Two experiments were conducted to investigate the effects of fermented sesame meal (FSM) replacement by soybean meal (SBM) in broilers. In the first experiment, a completely randomised design with 3 × 4 factorial arrangement was used for microorganism comparison [Lactobacillus acidophilus (LA), Saccharomyces cerevisiae (SC) and LA + SC] and fermentation time courses (0, 2, 7, and 12 days). In the second experiment, a total of 420-day-old boilers (Ross 308®) were randomly allocated to seven treatments and five replicates. The experimental treatments include basal diet based on soybean meal (SBM), SBM substituted with 15 and 25% raw sesame meal (RSM) with and without phytase (PHX); RSM15, RSM25, RSM15 + PHX, and RSM25 + PHX, and SBM substituted with 15 and 25% FSM; FSM15 and FSM25. Results showed that, in the fermentation process, the main effects of microorganism and days of fermentation significantly affected the pH and crude protein (p < .05). Also, the main effect of day of fermentation was found significant for phytic acid, oxalate and crude fibre (p < .05). The crude protein was increased when a mixture of LA and SC was used (p < .05). Broilers fed RSM25 had the lowest and the highest BWG and FCR, respectively, than those fed other diets on day 42 (p < .05). Compared to all diets except for RSM15, RSM25 a reduction was recorded for phosphorus digestibility (p < .05). In conclusion, fermentation process improved the nutrient value of the RSM and subsequently performance of the broilers and could be used as a protein source in broiler diets.
Fermentation process leads to significant decrease in phytic acid content of sesame meal.
Raw sesame meal had adverse effects on the performance of broilers.
Fermented sesame meal may improve the broilers performance similar to soybean meal.
Highlights
Introduction
Raw sesame meal (RSM) is the residue after oil extracting from sesame seed. There are many scientific reports on the nutritional values of sesame meal. The protein content of RSM is up to 40–60% (Pan et al. Citation1992; Mamputu and Buhr Citation1995); therefore, it may be considered as a suitable alternative for soybean meal. The RSM is also a rich source of minerals; though, its mineral availability is lower due to high levels of oxalate and phytate. In addition, Ravindran and Blair (Citation1992) proposed that substitution of RSM in broiler’s starter diets is limited because of its high fibre content and possible availability problems associated with phytate and oxalate. Meanwhile, results from different studies revealed that, substitution of soybean meal with RSM could be safe up to 15%. Various processing methods of RSM, such as heating, soaking, phytase enzyme supplementation, and fermentation have been found with notable effects on its nutrient quality and accessibility; hence, the nutrient composition of RSM varies widely depending on the processing methods. For instance, Lease and Williams (Citation1967) showed that high level of oxalate and phytic acid of RSM could be reduced under heating process. The usefulness of phytase to improve phosphorous and Calcium digestibility in poultry nutrition is generally accepted and substantial number of scientific reports provide clear evidence for these effects (Lei and Stahl Citation2001; Ravindran et al. Citation2006; Selle and Ravindran Citation2007). Also, a significant relationship was reported between the proportion of dietary phytic acid destruction by exogenous phytase and the ileal amino acid digestibility coefficients, where phytase effects ranged from 2–3% to 9–10% as phytic acid destruction increased from <10% to around 50% (Cowieson et al. Citation2017). Another research finding showed that soaking of whole and dehulled black sesame seeds in water decreased oxalate contents by 26.78% and 29.64%, respectively (Makinde and Akinoso Citation2013). Regarding the fermentation, it has been reported that anti-nutritional factors (e.g. tannin, phytic acid and trypsin inhibitor) of fermented sesame oil cake by Bacillus subtilis subsp were lower than that of unfermented sesame oil cake (Das and Ghosh Citation2015). Olude et al. (Citation2016) demonstrated that reduction in phytic acid and tannin of RSM as a result of lactic acid fermentation. Furthermore, it has been showed that the fermented sesame meal (FSM) by Lactobacillus acidophilus (LA) completely removed its phytic acid content (Mukhopadhyay and Ray Citation1999b, Citation1999a). Also, Hassaan et al. (Citation2015) reported that submerged fermentation of soybean meal with Saccharomyces cerevisiae (SC) reduced its phytic acid content lower than detectable levels, which may provide nutritional benefits to monogastric animals, particularly weaned pigs. To the best of our knowledge, there is no study to investigate the effect of FSM on the productivity of broiler chickens. Based on such findings, the assumption is that fermentation of RSM by L. acidophilus and S. cerevisiae could promising increase nutrient utilisation and subsequently cause an improvement in growth performance of broiler chickens. Results from different studies revealed that the L. acidophilus (Onipede et al. Citation2020) and S. cerevisiae (Foster and Nakata Citation2014; Olukomaiya et al. Citation2020) have an enzymatic potential to improve nutrient utilisation and accessibility of nutrients of legume seeds in the livestock diets by decreasing anti-nutritional factors.
Materials and methods
Two experiments were designed. In the first experiment, the individual and combinatorial effects of LA and SC on the nutrient availability of RSM in different time (0, 2, 7 and 12 days) were investigated. Based on the findings of this step, the duration and the microbial combination of fermentation were determined. In the second experiment, the effects of sesame meal processed by fermentation and phytase enzyme on the performance, nutrient digestibility, digestive enzyme activities, carcase characteristics, digestive tracts development and lymphoid organs in broiler chickens were investigated.
Fermentation process
LA (PTCC: 1643) was prepared from Persian Type Culture Collection of Iranian Research Organisation for Science and Technology (IROST), and SC (Saf-levure, Lesaffre Group, France) were purchased from local Market. In Fermentation process a completely randomised design with 3 × 4 factorial arrangement was used for microorganism comparison (LA, SC and LA + SC) and fermentation time courses (0, 2, 7 and 12 days). The FSM was prepared according to the method used by Hassaan et al. (Citation2015). Firstly, the raw sesame meal (RSM) was provided by the local company (Arsham Company, Tehran, Iran) and grounded to favourable particle size (0.5 mm >). Then, RSM was diluted in distilled water at 1:1 ratio and autoclaved at 121 °C for 15 min. For assurance, the chemical composition of sesame meal was analysed before and after fermentation and no significant difference were observed (data not shown). After cooling to room temperature, RSM was inoculated with LA and SC individually and combinatorically (1:1) at doses of 1 × 106 CFU/g meal. This provided a microorganism density of 1 × 103 cell/g substrate. Finally, inoculated RSM with different microorganisms (LA, SC and LA + SC) were incubated at 37 °C for different time courses (0, 2, 7 and 12 days). Finally, FSM was dried at 60 °C for 2 days. In day 0 and 2, 7 and 12 days after fermentation, 20 g of FSM were sampled to analyse the content of anti-nutritional factors and chemical composition. The chemical composition (dry matter, crude protein and fibre, ether extract, ash, oxalate, and phytic acid) were determined using the methods of the Association of Official Analytical Chemists (AOAC 1995). The chemical composition and pH of SM before and after fermentation are shown in Table . In addition, the amino acid profile of RSM and FSM was determined according to the method described by Fierabracci et al. (Citation1991) and are shown in Table .
Table 1. The chemical composition of sesame meal before and after fermentation.
Table 2. Amino acid profile of Sesame meal before and after fermentation by combination of Lactobacillus acidophilus and Saccharomyces cerevisiae after 12 days.
Bird and treatments
Farm experimental procedures were conducted in accordance with animal ethics committee guidelines of Guilan university. 420-day-old male broilers (Ross 308) were supplied from the commercial hatchery, individually weighed and allocated to a completely randomised design experiment with 7 treatments and 5 replicates (12 birds per pen). Diets were offered ad libitum and water was freely available. The experimental treatments were designed as follows: basal diet based on soybean meal (SBM), SBM substituted with 15% and 25% raw sesame meal (RSM) with and without phytase enzyme [5000 FTU phyzyme XP/g (PHX)]; RSM15, RSM25, RSM15 + PHX, and RSM25 + PHX, and SBM substituted with 15 and 25% FSM; FSM15 and FSM25. The chemical composition of the starter (1–10 days), grower (11–24 days) and finisher (25–42 days) diets are shown in Table . All diets were fed in mash form and the experiment endured for 42 days.
Table 3. Composition and calculated analysis of the different experimental diets.
Performance parameters
Body weights of the broiler chicken and their feed intakes (FI) were recorded weekly. Mortality was recorded daily, and body weight gain (BWG), FI, and feed conversion ratio (FCR) for d 21 were calculated. Any died bird was weighed and FCR were calculated by dividing total feed intake by weight gain of live plus died birds.
Nutrient digestibility and digestive enzyme activities
On day 42, the birds were fasted for 24 h and then allowed to consume the experimental diets for 3 days before the collection of faecal samples, 10 g celite as a source of acid insoluble ash (AIA) was added per kg of diet and content of the AIA in diet and faeces were measured according to the De Coca-Sinova et al. (Citation2011). Then, faecal samples were pooled, lyophilised, ground to pass through a 0.5-mm sieve, and stored at 4 °C until laboratory analysis. Experimental diets and faecal samples were analysed as described by AOAC (Citation2005); briefly dry matter content using oven-drying (Method no. 930.15), crude protein content using Kjeldahl (Method no. 984.13), and phosphorus concentration using spectrophotometr (Method no. 965.17). All values are expressed on a dry matter basis. The following equation was used to calculate the digestibility coefficient:
Digestive enzyme activities were determined using two randomly selected chicks per pen; euthanized by cervical dislocation, and then the mixed small intestinal (duodenum, jejunum, and ileum) contents were sampled. The intestinal digesta samples were diluted, homogenised, and centrifuged using the protocol described by Jin et al. (Citation2000). Afterwards, the supernatants were divided into small portions and stored at −80 °C till enzyme assays. Amylase activity was measured using soluble starch as a substrate, as described by Bernfeld (Citation1955). Briefly, the mixture of supernatant and substrate was incubated at 40 °C for 1 h, and the reaction was stopped by adding 1 mL dinitrosalicylic acid solution, followed by heating in boiling water for 5 min and cooling to room temperature. Maltose was determined by staining and colour intensity was measured using spectrophotometer (Cecil 7250) at 540 nm. One unit of α-amylase activity was defined as the amount of enzyme that produced one milligram of maltose per minute at 40 °C. Protease activity was analysed using the method reported by Lynn and Clevette-Radford (Citation1984). A 0.2% solution of azocasein (substrate) in 1 mL of 200 mM Tris Hydrochloride buffer, pH 7, was digested by enzyme at 37 °C for 2 h. The reaction was stopped with trichloroacetic acid (1 mL), clarified by centrifugation, and supernatant liquid absorbance was measured at 410 nm by spectrophotometer. The protease activity unit was defined as milligrams of azocasein degraded for two hours incubation at 38 °C per mg of total intestinal digesta protein.
Carcase characteristics, digestive tracts development and lymphoid organs
On day 42, two birds per pen with body weights closest to the mean weight of the same pen were selected, weighed, and euthanized by cervical dislocation. After removal of feathers, feet, and head the carcase yield was determined. Cut-up parts such as thigh, breast, and abdominal fat were weighed. In addition, the empty weight of intestinal, gizzard, different parts of small intestine, and caeca were recorded. Then pancreas, thymus, liver, spleen, and bursa of Fabricius were removed and cleaned from adherent tissues. The weight of the different organs was expressed as relative weights (g/kg live body weight).
Statistical analysis
The data of first experiment were analysed by two-way ANOVA to determine the main effects (microorganism and time) and their interaction using general linear models procedure of the SAS (2009). The data of second experiment were analysed by one-way ANOVA using general linear model procedure of SAS (Citation2009). Differences between means were evaluated using Tukey’s multiple comparison test. The p-values of ≤.05 were considered statistically significant, whereas values of .05 < p < .10 were declared a near-significant trend.
Results
Chemical composition and amino acid profile
The influence of microorganism strain and fermentation period on the chemical composition of RSM is presented in Table . The main effects of microorganism and fermentation times were significant factors affecting pH and crude protein of FSM (p < .05), However, no significant microorganism × day interaction was found for pH and crude protein (p > .05). LA + SC had a higher percentage of crude protein and LA showed a lower pH compared to other treatments (p < .05). In addition, the dry matter of FSM was not influenced by fermentation (p > .05). Considering the percentage of ether extract and ash in fermented treatments, there were no significant differences between treatments (p > .05), though, fermentation time had a significant effect on the two indices mentioned (p < .05). However, no interaction between microorganism strain and period of fermentation was observed for ether extract and ash (p > .05). As the fermentation time increased, the percentage of crude fibre, phytic acid and oxalate of FSM significantly decreased (p < .05). While for microorganism × day interaction, no significant differences were found for the percentage crude fibre, phytic acid and oxalate. In addition, the lowest percentage of phytic acid and oxalate in the FSM was observed after 12 days.
Amino acid profile of RSM before and after 12 days fermentation by combination of LA and SC (LA + SC) is presented in Table . The mean values of methionine, threonine, isoleucine, histidine, tryptophan and serine of FSM were recorded as 1.17, 1.40, 1.96, 1.06, 0.78, and 1.77%, respectively, which indicate a marginal increase over the RSM; whereas, significant increase was observed in mean values of cysteine, lysine, valine, leucine, arginine, phenylalanine, tyrosine, alanine, aspartate, glutamate, glycine, and proline in FSM compared to RSM (p < .05). In addition, aspartic acid (3.55 and 4.21%), glutamate (8.54 and 10.37%), and arginine (4.77 and 5.19%) were found to be the most abundant amino acids in the raw and fermented seed flours, respectively. These findings indicate that, total amino acids content of FSM significantly increased compared to RSM (p < .05).
Performance
Results from feeding trial, showed that BWG and FCR of birds were significantly affected by dietary treatments (Table ), however, no significant differences were observed in FI between treatments for the entire experimental period. From 1 to 10 days of age, inclusion of RSM25 to the diet decreased BWG and increased FCR compared to other diets except for RSM15 containing diet (p < .05).
Table 4. Effect of experimental treatments on the weight gain (g/bird), feed intake (g/bird), feed conversion ratio (g feed/g gain) in broilers.
From 11 to 24 days of age, BWG was reduced (p < .05) as a result of inclusion of RSM25, but other treatments showed no significant differences with diet containing SBM. In addition, FCR of broilers fed RSM25 was higher (p < .05) than other treatments except RSM15 and FSM25.
From 25 to 42 days of age, the chicks fed RSM25 had lower BWG and higher FCR (p < .05) than those fed SBM, while, other treatments had no significant difference in BWG and FCR with SBM treatment. Overall, the inclusion of RSM25 in the diet decreased BWG and subsequently increased FCR in the whole period of the experiment (1–42 days).
Nutrient digestibility and digestive enzyme activities
The effects of dietary treatments on the nutrient digestibility and intestinal digestive enzyme activities in broilers on day 42 are shown in Table . There was no significant difference in the digestibility of dray matter and protein between SBM diet and the other diets (p > .05), however, phosphorus digestibility was decreased (p < .05) by RSM25 compared to other treatments, except for RSM15. Interestingly, there is no significant difference between FSM and SMB containing diets. As shown in Table , compared to SBM diet, all treatments did not have a significant effect on amylase and protease activities on day 42 (p > .05).
Table 5. Effect of dietary treatments on faecal dry matter, crude protein and Phosphorus digestibility and digestive enzyme activity of intestinal digesta of broilers at 42 days of age.
Carcase characteristics, digestive tracts development and lymphoid organs
The effects of the experimental diets on carcase characteristics, digestive tracts development, and lymphoid organs are shown in Table . Compared to FSM and RSM + PHX diets, only broilers fed diet containing RSM25 showed a significant decrease in carcase yield and breast weight on day 42 (p < .05), though, no differences were recorded between other diets (p > .05). Birds that were received diet containing FSM25 showed a tendency towards a decrease in abdominal fat (p = .07). The relative weights of digestive organs (gizzard, liver, pancreas, intestinal, duodenum, jejunum, ileum and cecum) and lymphoid organs (thymus, bursa and spleen) were not affected by dietary treatments (p > .05).
Table 6. Effect of experimental treatment on the relative weight of digestive and Lymphoid organs and Carcase [(g/kg body weight) × 100] of broilers.
Discussion
Chemical composition and amino acid profile
The present investigation was intended to assess the effectiveness of LA and SC to improve the nutritive value of RSM under different fermentation periods. During the fermentation, the chemical composition of RSM as a result of metabolic activity of LA and SC was changed. As shown in Table , obviously, increasing the day of fermentation process resulted in a decrease in pH, phytic acid, oxalate and crude fibre, while the crude protein increased in FSM and the best results obtained after 12 days of fermentation. Another point to note is that, despite numerically reduction in pH, phytic acid, and oxalate content, it was not possible to estimate that the LA + SC treatment was more effective or that each of them alone had an effect to reduce, pH, phytic acid, oxalate and crude fibre. However, the main effects of LA + SC showed a significant increase in crude protein compared to other treatment. In the previous studies, a remarkable variation was reported in chemical compositions of FSM, partly due to the differences in the processing methods (mechanical press or solvent extraction) during the oil extraction step from the sesame seeds and duration of fermentation process. However, a similar trend has been reported in terms of RSM compounds in the fermentation process. The results of some studies indicate that combination of LA and fungi significantly decreased the pH of the fermented rapeseed meal (Chiang et al. Citation2009; Ashayerizadeh et al. Citation2017). In another study where wheat flour and whole barley were incorporated into the fermentation process, a similar decrease in pH was observed, while lactic acid bacteria population increased (Skrede et al. Citation2003). The use of the mixture of LA and SC in the present study seems to create an environment where the SC consumes the oxygen present in the bag (Chiang et al. Citation2009) and subsequently provides a better environment for LA to produce organic acids resulting in a decrease in the pH of FSM (Van Winsen et al. Citation2001; Canibe et al. Citation2008; Chamberlain et al. Citation2019).
Furthermore, it has been shown that the fermentation of RSM for 15 days using Bacillus licheniformis decreased the crude fibre and phytic acid, while it increased the crude protein content (Roy et al. Citation2014). Olude et al. (Citation2016) noted that fermentation of RSM by Lactobacillus plantarium for 48 h, increased the crude protein from 18.02% to 24.43%. They Also reported that the fermentation process decreased the crude fibre and phytic acid contents of FSM from 37.2 to 22 g/kg and 7.2 to 6 g/kg, respectively. The fermentation of RSM by Lactobacillus acidophilus slightly increased the protein and lipid contents and decreased the phytic acid (Mukhopadhyay and Ray Citation1999a). Results from another study showed that the crude fibre, phytic acid and oxalate contents of fermented sesame seed decreased by 46, 50 and 69% respectively, while the crude protein and fat content increased marginally at the end of 96 h of fermentation (Olagunju and Ifesan Citation2013). Results obtained in this study showed that fermentation of RSM by LA + SC for 12 days leads to an increase in amino acid content. It has been demonstrated that amino acid would increase by increasing the crude protein in the fermented soybean meal (Chen et al. Citation2010). Olagunju and Ifesan (Citation2013) reported that aspartic acid, glutamic acid, and arginine were the most abundant amino acids in unfermented and fermented sesame flour, which agrees with the results of this study. The increase in protein content can be attributed to microbial synthesis of proteins, secretion of enzymes, and other biological products during fermentation by microorganisms (Elyas et al. Citation2002; Zhang et al. Citation2007). On the other hand, observed decrease in fibre content during fermentation by LA + SC treatment could be attributed to the partial solubilisation of cellulose and hemicellulosic type of material by microbial enzymes (Olagunju and Ifesan Citation2013).
The reasons for the low level of phytic acid content of FSM could be related to the enzymatic degradation of phytic acid by Lactobacillus spp. and S. cerevisiae that have been previously reported (Olukomaiya et al. Citation2020; Onipede et al. Citation2020). Furthermore, the enzymatic degradation of phytic acid by phytase enzyme requires an optimum pH which can be provided by natural fermentation (Gupta et al. Citation2015). The findings of the present study indicate the influence of fermentation on oxalate degradation, which is consistent with the results of the previous studies using L. acidophilus (Hatch Citation2017; Chamberlain et al. Citation2019). There is also evidence about an enzyme, known as S. cerevisiae acyl-activating enzyme 3 (ScAAE3), that catalyse the conversion of oxalate to oxalyl-CoA and reducing the inhibitory effects of oxalate on growth (Foster and Nakata Citation2014).
Performance
Beneficial effects of the phytase supplementation on the performance of broilers are well known and proven that phytase can increase the availability of several nutrients in diets (Simons et al. Citation1990; Gordon and Roland Citation1998; Kaneko et al. Citation2002; Shirley and Edwards Jr Citation2003; Rahimian et al. Citation2013). Over the entire experimental period (days 1–42), the experimental treatments that were received RSM25 showed lower BWG and FCR than SBM diet, while those fed with FSM did not have a significant difference with SBM diet. There was no significant difference in FI of diets incorporating RSM and FSM relative to SBM diet. However, feeding RSM25 to broilers typically reduced BWG and impaired the growth compared with feeding SBM and FSM. These findings are in line with Farran et al. (Citation2000) who reported weight gain of broiler chick in diet containing 12% sesame hulls was reduced compared to control diet. Furthermore, it has been reported that BWG and FCR of broiler were impaired by increasing the level of RSM in broilers (Mamputu and Buhr Citation1995; Rahimian et al. Citation2013). However, other studies reported that RSM could be safely used up to 15% in the broiler and Japanese quail diets without a negative effect on performance (Ravindran and Blair Citation1992; Yamauchi et al. Citation2006; Sina et al. Citation2014; Ghazvinian et al. Citation2016). In consistent to the result of the present study, previous studies indicate that inclusion of the RSM more than 15% had adverse effects on the performance of the broiler chicken. There is no study comparing the impacts of the FSM and RSM on the performance of the broiler chickens more than 15%. However, this study showed that the fermentation of RSM by LA + SC significantly decreased the antinutritional factors (phytic acid and oxalate) and increased the availability of some nutrients (phosphorus and protein). Also, the low pH and high lactic acid bacteria population of FSM made it the best trigger to modulate microbial balance towards benefit bacterial and consequently improving intestinal morphology of broilers (presented data in another manuscript). As a result, broilers that were received FSM15 and FSM25 showed better performance (similar to SBM containing diet) compared to those fed RSM25.
Nutrient digestibility and digestive enzyme activities
Phosphorus digestibility in chicks receiving different levels of RSM + PHX and FSM significantly improved, and it was similar with those fed SBM diet (Table ). The capacity of phytase to increase the total phosphorus digestibility in broilers has been frequently demonstrated. It has been reported that phytase increased ileal phosphorus digestibility by 14.7% in broiler diets containing 4.5 g/kg non-phytate phosphorus (Ravindran et al. Citation2000). In addition, Ravindran et al. (Citation2006) reported that in a corn-soybean meal-based diet the ileal digestibility of phosphorus was decreased by increasing the phytate concentration and increased with increasing phytase content. The results of another study showed that adding phytase to the piglet diet containing RSM, did not affect apparent ileal digestibility of dry matter, crude protein, and energy digestibility but significantly improved the apparent ileal digestibility of calcium and phosphorus (de Souza et al. Citation2017). The phytase enzymes function to hydrolyse phytate and consequently release of phosphorus and other nutrients are well proved in many studies (Jendza et al. Citation2006; Selle and Ravindran Citation2007; Adeola Citation2010; Kong and Adeola Citation2011). To the best of our knowledge, no studies have reported concerning the effects of FSM on the digestibility of broilers chickens. However, available literatures have provided some reports concerning the effect of Lactobacillus spp. and Saccharomyces spp. to reduce phytate content of oilseeds and their meal during the fermentation process. For example, during starter phase, fermented rapeseed meal by a mixed liquid culture containing Lactobacillus fermentum, Enterococcus faecium, Saccharomyces cerevisae, and Bacillus subtilis increased the apparent digestibility of dry matter and phosphorus compared to unfermented rapeseed meal in broiler chickens (Chiang et al. Citation2009). A possible explanation for the significant increase in phosphorus digestibility observed in FSM-fed broilers in the present study could be related to phytase activities of Lactobacillus spp. (Taheri et al. Citation2009; García-Mantrana et al. Citation2016; Priyodip et al. Citation2017) and SC (Kłosowski et al. Citation2018) which have been previously reported. It is widely acknowledged that decomposition of phytic acid, increases the availability of many cations and followed by nutritional value (West Citation2014; Gupta et al. Citation2015).
Carcase characteristics, digestive tracts development and lymphoid organs
Results recorded for live weight and carcase weight indicated that the addition of RSM leads to decrease of these indices which is in agreement with findings of Rahimian et al. (Citation2013) and David and John (Citation2015). Another report showed that dressing percentage and slaughter weight were not affected by 9 and 18% RSM, while inclusion of 36% RSM resulted in a decrease (Shanti et al. Citation2012). They also showed that RSM and phytase interaction resulted in heavier giblets in birds fed with 36% RSM supplemented with 600 FTU/kg phytase. In addition, it has been showed that as the dietary RSM level increased, the dressing proportion was decreased (Kaneko et al. Citation2002). Al Harthi and El Deek (Citation2009) demonstrated that lower dosage inclusion of RSM (5, 10 and 15%) with and without phytase in diet of broilers did not have significant effects on the carcase, breast, liver, heart, thigh, and gizzard. Similarly, it has been reported that there are no significant differences on carcase dressing percentage and meat in broilers fed with 5 and 10% RSM compared with control diet (Nikolakakis et al. Citation2014). Regarding the abdominal fat, it has been shown that the abdominal fat percentage was decreased by feeding RSM and phytase enzyme (Rahimian et al. Citation2013). Also, relative weights of abdominal fat were not affected by inclusion of RSM up to 0.67 of SBM, but it decreased significantly with further increase in RSM up to 1.00 of SBM (Rama Rao et al. Citation2008). However, in this research, there was a tendency towards decreasing abdominal fat by FSM25. Also, the percentage of the abdominal fat was numerically decreased by increasing the level of RSM. Reduced feed efficiency and poor availability of protein and phosphorus (Tables and ) in broilers receiving RSM as a substitute for SBM might be responsible for low carcase yield. Based on findings in this study, adverse effects of RSM could be eliminated by fermentation process. As shown in Table , not only the carcase yield of the birds fed with two levels of FSM did not significant differences with control and phytase containing diets but also there was a tendency to decrease the abdominal fat.
Conclusions
The in vitro results indicated that, increasing the time course of fermentation, the availability of nutrients in FSM increased, and the best results obtained after 12 days. However, in concern to availability of nutrients in FSM among treatments, clear differences have not obtained between the individual and combinatorial effects of the LA and SC. Yet, the main effects of the microorganism to increase crude protein was significant for LA + SC treatment. The in vivo results showed that the FSM and RSM + PHX diets improved phosphorus digestibility and subsequently performance of broilers as same as SBM diets. Based on the findings of this study, diets containing different levels of RSM25 reduced BWG and impaired the growth compared to other diets. All in all, more detailed studies are needed to completely remove of oxalate and phytic acid in RSM.
Disclosure statement
No potential conflict of interest was reported by the author(s).
Data availability statement
The data that support the findings of this study are available from the corresponding author, Majid Mottaghitalab, upon reasonable request.
References
- Adeola O. 2010. Phosphorus equivalency value of an Escherichia coli phytase in the diets of White Pekin ducks. Poult Sci. 89:1199–1206.
- Al Harthi M, El Deek A. 2009. Evaluation of sesame meal replacement in broiler diets with phytase and probiotic supplementation. Egypt Poult Sci J. 29:99–125.
- AOAC. 2005. Official Methods of Analysis of the Association Official Analytical Chemists. 18th ed. Gaithersburg (MA): AOAC International.
- Ashayerizadeh A, Dastar B, Shargh MS, Mahoonak AS, Zerehdaran S. 2017. Fermented rapeseed meal is effective in controlling Salmonella enterica serovar Typhimurium infection and improving growth performance in broiler chicks. Vet Microbiol. 201:93–102.
- Bernfeld P. 1955. Amylase α and β. Methods Enzymol. 1:149–155.
- Canibe N, Miettinen H, Jensen B. 2008. Effect of adding Lactobacillus plantarum or a formic acid containing-product to fermented liquid feed on gastrointestinal ecology and growth performance of piglets. Livest Sci. 114:251–262.
- Chamberlain CA, Hatch M, Garrett TJ. 2019. Metabolomic profiling of oxalate-degrading probiotic Lactobacillus acidophilus and Lactobacillus gasseri. PLoS One. 14:e0222393.
- Chen C, Shih Y, Chiou P, Yu B. 2010. Evaluating nutritional quality of single stage-and two stage-fermented soybean meal. Asian Australas J Anim Sci. 23:598–606.
- Chiang G, Lu W, Piao X, Hu J, Gong L, Thacker P. 2009. Effects of feeding solid-state fermented rapeseed meal on performance, nutrient digestibility, intestinal ecology and intestinal morphology of broiler chickens. Asian Australas J Anim Sci. 23:263–271.
- Cowieson A, Ruckebusch JP, Sorbara J, Wilson J, Guggenbuhl P, Roos F. 2017. A systematic view on the effect of phytase on ileal amino acid digestibility in broilers. Anim Feed Sci Technol. 225:182–194.
- Das P, Ghosh K. 2015. Improvement of nutritive value of sesame oil cake in formulated diets for rohu, Labeo rohita (Hamilton) after bio-processing through solid state fermentation by a phytase-producing fish gut bacterium. Int J Aquat Biol. 3:89–101.
- David O, John O. 2015. Response of broiler chickens fed diets containing differently processed Sesame (Sesame indicum L.) seed meal. ARJASR. 3:13–20.
- De Coca-Sinova A, Mateos GG, González-Alvarado J, Centeno C, Lázaro R, Jiménez-Moreno E. 2011. Comparative study of two analytical procedures for the determination of acid insoluble ash for evaluation of nutrient retention in broilers. Span J Agric Res. 9:761–768.
- de Souza TR, Escobar K, Aguilera G, Ramírez B, Mariscal-Landín R. 2017. Sesame meal as the first protein source in piglet starter diets and advantages of a phytase: a digestive study. SA J an Sci. 47:606–615.
- Elyas SH, El Tinay AH, Yousif NE, Elsheikh EA. 2002. Effect of natural fermentation on nutritive value and in vitro protein digestibility of pearl millet. Food Chem. 78:75–79.
- Farran M, Uwayjan M, Miski A, Akhdar N, Ashkarian V. 2000. Performance of broilers and layers fed graded levels of sesame hull. J Appl Poult Res. 9:453–459.
- Fierabracci V, Masiello P, Novelli M, Bergamini E. 1991. Application of amino acid analysis by high-performance liquid chromatography with phenyl isothiocyanate derivatization to the rapid determination of free amino acids in biological samples. J Chromatogr B, Biomed Sci Appl. 570:285–291.
- Foster J, Nakata PA. 2014. An oxalyl-CoA synthetase is important for oxalate metabolism in Saccharomyces cerevisiae. FEBS Lett. 588:160–166.
- García-Mantrana I, Yebra MJ, Haros M, Monedero V. 2016. Expression of bifidobacterial phytases in Lactobacillus casei and their application in a food model of whole-grain sourdough bread. Int J Food Microbiol. 216:18–24.
- Ghazvinian K, Pour HA, Alanghi AR. 2016. Effect of sesame meal supplementation to the feed on performance, blood parameters and physiology characteristics in Japanese quail. Entomol Appl Sci Lett. 3:71–75.
- Gordon RW, Roland DA. 1998. Influence of supplemental phytase on calcium and phosphorus utilization in laying hens. Poult Sci. 77:290–294.
- Gupta RK, Gangoliya SS, Singh NK. 2015. Reduction of phytic acid and enhancement of bioavailable micronutrients in food grains. J Food Sci Technol. 52:676–684.
- Hassaan MS, Soltan MA, Abdel-Moez AM. 2015. Nutritive value of soybean meal after solid state fermentation with Saccharomyces cerevisiae for Nile tilapia, Oreochromis niloticus. Anim Feed Sci Technol. 201:89–98.
- Hatch M. 2017. Gut microbiota and oxalate homeostasis. Ann Transl Med. 5:36–36.
- Jendza J, Dilger R, Sands J, Adeola O. 2006. Efficacy and equivalency of an Escherichia coli-derived phytase for replacing inorganic phosphorus in the diets of broiler chickens and young pigs. J Anim Sci. 84:3364–3374.
- Jin L, Ho Y, Abdullah N, Jalaludin S. 2000. Digestive and bacterial enzyme activities in broilers fed diets supplemented with Lactobacillus cultures. Poult Sci. 79:886–891.
- Kaneko K, Yamasaki K, Tagawa Y, Tokunaga M, Tobisa M, Furuse M. 2002. Effects of dietary sesame meal on growth, meat ingredient and lipid accumulation in broilers. J Poult Sci (Japan), 39:56–62.
- Kłosowski G, Mikulski D, Jankowiak O. 2018. Extracellular phytase production by the wine yeast S. cerevisiae (Finarome Strain) during submerged fermentation. Molecules. 23:848.
- Kong C, Adeola O. 2011. Protein utilization and amino acid digestibility of canola meal in response to phytase in broiler chickens. Poult Sci. 90:1508–1515.
- Lease JG, Williams WP. 1967. Availability of Zinc and comparison of in vitro and in vivo zinc uptake of certain oil seed meals. Poult Sci. 46:233–242.
- Lei X, Stahl C. 2001. Biotechnological development of effective phytases for mineral nutrition and environmental protection. Appl Microbiol Biotechnol. 57:474–481.
- Lynn K, Clevette-Radford N. 1984. Purification and characterization of hevain, a serine protease from Hevea brasiliensis. Phytochemistry. 23:963–964.
- Makinde F, Akinoso R. 2013. Nutrient composition and effect of processing treatments on anti nutritional factors of Nigerian sesame (Sesamum indicum Linn) cultivars. Int Food Res J. 20:2293.
- Makinde F, Akinoso R, Adepoju A. 2013. Effect of fermentation containers on the chemical composition of fermented sesame (Sesamum indicum L) seeds. AJFAND. 13:7122–7137.
- Mamputu M, Buhr R. 1995. Effect of substituting sesame meal for soybean meal on layer and broiler performance. Poult Sci. 74:672–684.
- Mukhopadhyay N, Ray A. 1999a. Effect of fermentation on the nutritive value of sesame seed meal in the diets for rohu, Labeo rohita (Hamilton), fingerlings. Aquac Nutr. 5:229–236.
- Mukhopadhyay N, Ray A. 1999b. Improvement of quality of sesame Seasamum indicum seed meal protein with supplemental amino acids in feeds for rohu Labeo rohita (Hamilton) fingerlings. Aquac Res. 30:549–557.
- Nikolakakis I, Bonos E, Kasapidou E, Kargopoulos A, Mitlianga P. 2014. Effect of dietary sesame seed hulls on broiler performance, carcass traits and lipid oxidation of the meat. Eur Poult Sci. 78: 28.
- Olagunju AI, Ifesan BO. 2013. Changes in nutrient and antinutritional contents of sesame seeds during fermentation. J Microbiol Biotechnol Food Sci. 2:2407.
- Olude O, George F, Alegbeleye W. 2016. Utilization of autoclaved and fermented sesame (Sesamum indicum L.) seed meal in diets for Til-aqua natural male tilapia. Anim Nutr. 2:339–344.
- Olukomaiya O, Adiamo OQ, Fernando WC, Mereddy R, Li X, Sultanbawa Y. 2020. Effect of solid-state fermentation on proximate composition, anti-nutritional factor, microbiological and functional properties of lupin flour. Food Chem. 315:126238.
- Onipede G, Aremu B, Sanni A, Babalola O. 2020. Molecular study of the phytase gene in lactic acid bacteria isolated from Ogi and Kunun-Zaki. African Fermented Cereal Gruel and Beverage. Applied Food Biotechnology. 7:49–60.
- Pan Y, Hy L, Gao Q. 1992. The optimum allowance of sesame cake meal for broilers. Chin J Anim Sci. 28:26–27.
- Priyodip P, Prakash PY, Balaji S. 2017. Phytases of probiotic bacteria: characteristics and beneficial aspects. Indian J Microbiol. 57:148–154.
- Rahimian Y, Valiollahi S, Tabatabaie S, Toghiani M, Kheiri F, Rafiee A, Khajeali Y. 2013. Effect of use cumulative levels of sesame (Sesamum indicum L.) meal with phytase enzyme on performance of broiler chickens. World Appl Sci J. 26:793–800.
- Rama Rao S, Raju M, Panda A, Poonam N, Sunder GS, Sharma R. 2008. Utilisation of sesame (Sesamum indicum) seed meal in broiler chicken diets. Br Poult Sci. 49:81–85.
- Ravindran V, Blair R. 1992. Feed resources for poultry production in Asia and the Pacific. II. Plant protein sources. Worlds Poult Sci J. 48:205–231.
- Ravindran V, Cabahug S, Ravindran G, Selle P, Bryden W. 2000. Response of broiler chickens to microbial phytase supplementation as influenced by dietary phytic acid and non-phytate phosphorous levels. II. Effects on apparent metabolisable energy, nutrient digestibility and nutrient retention. Br Poult Sci. 41:193–200.
- Ravindran V, Morel P, Partridge G, Hruby M, Sands J. 2006. Influence of an Escherichia coli-derived phytase on nutrient utilization in broiler starters fed diets containing varying concentrations of phytic acid. Poult Sci. 85:82–89.
- Roy T, Banerjee G, Dan SK, Ghosh P, Ray AK. 2014. Improvement of nutritive value of sesame oilseed meal in formulated diets for rohu, Labeo rohita (Hamilton), fingerlings after fermentation with two phytase-producing bacterial strains isolated from fish gut. Aquacult Int. 22:633–652.
- SAS I. 2009. Sas/Graph 9.2: Graph Template Language User’s Guide. SAS Institute.
- Selle PH, Ravindran V. 2007. Microbial phytase in poultry nutrition. Anim Feed Sci Technol. 135:1–41.
- Shanti H, Abo Omar J, Al-Shakhrit K, Ghany AA. 2012. Performance and some blood constituents of broilers fed sesame meal supplemented with microbial phytase. Asian Pac J Trop Biomed. 1:1–8.
- Shirley R, Edwards H Jr. 2003. Graded levels of phytase past industry standards improves broiler performance. Poult Sci. 82:671–680.
- Simons P, Versteegh HA, Jongbloed AW, Kemme P, Slump P, Bos K, Wolters M, Beudeker R, Verschoor G. 1990. Improvement of phosphorus availability by microbial phytase in broilers and pigs. Br J Nutr. 64:525–540.
- Sina G, Jafari M, Khojasteh S. 2014. The use of sesame meal in diets of Japanese Quail. Iran J Appl Anim Sci. 4:877–881.
- Skrede G, Herstad O, Sahlstrøm S, Holck A, Slinde E, Skrede A. 2003. Effects of lactic acid fermentation on wheat and barley carbohydrate composition and production performance in the chicken. Anim Feed Sci Technol. 105:135–148.
- Taheri H, Moravej H, Tabandeh F, Zaghari M, Shivazad M. 2009. Screening of lactic acid bacteria toward their selection as a source of chicken probiotic. Poult Sci. 88:1586–1593.
- Van Winsen RL, Urlings BA, Lipman LJ, Snijders JM, Keuzenkamp D, Verheijden JH, van Knapen F. 2001. Effect of fermented feed on the microbial population of the gastrointestinal tracts of pigs. Appl Environ Microbiol. 67:3071–3076.
- West TP. 2014. Effect of phytase treatment on phosphate availability in the potential food supplement corn distillers’ grains with solubles. J Food Process. 2014:1–5.
- Yamauchi K, Samanya M, Seki K, Ijiri N, Thongwittaya N. 2006. Influence of dietary sesame meal level on histological alterations of the intestinal mucosa and growth performance of chickens. J Appl Poult Res. 15:266–273.
- Zhang WJ, Xu ZR, Zhao SH, Sun JY, Yang X. 2007. Development of a microbial fermentation process for detoxification of gossypol in cottonseed meal. Anim Feed Sci Technol. 135:118–176.