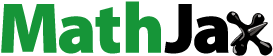
Abstract
The study aimed to assess the effects of dietary extruded linseed (ELS) and Lactobacillus acidophilus (LA) on performance, carcass traits, immune organ weights, plasma lipoprotein response, and caecal bacteria populations in broiler chickens. A total of 648 one-day-old chicks were divided into six groups in a 3 × 2 factorial arrangement consisting of three ELS levels (0, 6 and 12%) without or with probiotic L. acidophilus (0 and 20 g ton−1 feed). Each group had six replicates (18 birds/pen). Results showed no significant effects of ELS level nor LA supplementation on growth performance (body weight gain, feed intake, and feed conversion) of broilers during the overall period (1–42 days). The production efficiency factor increases (p = .045) with the LA addition. There were no effects of ELS level nor probiotic addition on carcase traits and immune organ weights, except for decreases in abdominal fat percentage (p = .027, respectively p = .035). The dietary ELS level significantly correlated with a decrease in the plasma total cholesterol (TC; p = .003), triglycerides, and very-low-density lipoprotein concentrations (p = .020), while the LA addition significantly correlated with a decrease (p = .015) in the plasma TC. The LA addition lowers the caecal pH (p = .007), Staphylococcus spp. and E. coli counts (p < .0001), and increase the caecal Lactobacillus spp. and lactobacilli: E. coli ratio (p < .0001). In conclusion, the use of ELS up to 12% supplemented with L. acidophilus in broiler chicks diet had a positive effect on health status, decreasing the abdominal fat deposition, plasma lipids and the caecal pathogen bacteria E. coli.
Extruded linseed up to 12% in broiler chicks’ diets had no significant effects on growth performance, carcass traits or immune organs size and improve the abdominal fat deposition and plasma lipids response due to the decreased dietary n − 6: n − 3 PUFA ratio.
Extruded linseed up to 12% supplemented with L. acidophilus in broiler chicks diet had a positive effect on health status improving production efficiency factor and decreasing abdominal fat deposition, the plasma lipids and the caeca pathogen bacteria E. coli.
Highlights
Introduction
Taking into consideration the importance of poultry meat and its quality, on the one hand, and the increasing demand of consumer for natural products, on the other hand, the use of microbial feed additives that acts as probiotic associated with n − 3 fatty acids (FA) rich feed ingredients could represent a practical way to meet these requirements.
Different kind of ingredients was evaluated over time to improve meat quality. Most of them have been shown to have high nutritional value, but the factors that limit their use is the content in antinutritional components. Among these, linseed is considered a valuable energy feed ingredient for poultry diets due to its rich content of oil (35–45%), with a favourable n − 3 polyunsaturated fatty acid (PUFA), especially α-linolenic acid (ALA; 45–52%) (Bhatty Citation1995; Singh et al. Citation2011). However, its use has received little attention due to the content in water-soluble polysaccharides (mucilage), cyanogenic glycosides, trypsin inhibitor, phytic acid (Dale and Batal Citation2008). These factors could negatively affect the gastrointestinal health and nutrient utilisation in poultry (Attia Citation2003; Apperson and Cherian Citation2017).
The dietary FA exerts primary physiological functions for fast-growing broilers, and it has been stated that the fat source modulates the FA composition of chicken meat, with health benefits for consumers and birds’ too (Bautista-Ortega et al. Citation2009; Gonzalez et al. Citation2011; Sridhar et al. Citation2017). Several studies have shown that dietary linseed enhanced chicken meat with n − 3 PUFAs ( Rodriguez et al. Citation2001; Attia Citation2003; Betti et al. Citation2009; Mridula et al. Citation2015), but could affect the growth performance (Alzueta et al. Citation2003; Mridula et al. Citation2015). Furthermore, from a nutritional point of view, linseed is also a valuable source of protein (20–30%), essential amino acids, fibre, minerals, and vitamins (Anjum et al. Citation2013).
The extrusion technology is an appropriate method to reduce the antinutritional factors in linseed (Anjum et al. Citation2013; Kostadinović et al. Citation2016), to modify lipid and carbohydrate structures, and to reduce the ileal digesta viscosity (Pirmohammadi et al. Citation2019). Previous studies (Anjum et al. Citation2013; Mridula et al. Citation2015) reported that feeding 5–15% extruded linseed in broilers diets affects growth performance, while other research (Kostadinović et al. Citation2016; Skrivan et al. Citation2020) stated that 6 or 10% extruded linseed improve broilers productive performance. However, the addition of up to 10% of extruded flaxseed in the diet increased the n − 3 FA (ALA, docosahexaenoic and eicosapentaenoic FAs) and decreased the n − 6/n − 3 ratio in broilers meat, with the favourable impact of human health (Anjum et al. Citation2013; Mridula et al. Citation2015; Kostadinović et al. Citation2016; Skrivan et al. Citation2020). Moreover, Kostadinović et al. (Citation2016) showed that 10% of extruded flaxseed had a significant effect on enzymatic and non-enzymatic antioxidative systems in the blood of broilers.
It is known that the probiotics beneficially modulate intestinal microbiota of the poultry and develop and stimulates the gut immune system with an impact on health (Mountzouris et al. Citation2007; Attia et al. Citation2017; De Cesare et al. Citation2020).
Lactobacillus strains have been shown to have positive effects on production performance (Smith Citation2014), chicken meat quality (Mountzouris et al. Citation2007), modulation of intestinal microflora and pathogen inhibition (Patterson and Burkholder Citation2003). It has been stated that mechanisms of action included competition for available nutrients, immune stimulation and the alteration of microbial and host metabolism (Patterson and Burkholder Citation2003; Yang et al. Citation2009; Huyghebaert et al. Citation2011; Al-Sagan et al. Citation2020). The major factors that affect probiotics efficacy are the bacterial strain, the dose (colony-forming unit/bird/day), the treatment period and the administration method (via feed or water) (Gallazzi et al. Citation2008). The L. acidophilus D2/CSL probiotic strain was isolated from the gastrointestinal tract of healthy chickens and is known for its ability to balance the intestinal microbiota (Bianchi et al. Citation1985). Several studies have revealed the efficiency of L. acidophilus D2/CSL used as probiotic on laying hen’s productive performance (Gallazzi et al. Citation2008; Cesari et al. Citation2014), on egg production and quality, immune status, or gut microflora and health in organic laying hens (Forte et al. Citation2016a, Citation2016b).
A few feeding trials investigated the effects of this probiotic strain on growth performance and metabolic function in broilers (De Cesare et al. Citation2017), or performances and gut health in rurally reared chickens (Forte et al. Citation2018).
To our knowledge, there are no studies that investigated the combined effect of extruded linseed and probiotic on plasma metabolites, gastrointestinal health, and performance in broilers. Thus, we hypothesised that the probiotic addition to broiler diets including up to 12% extruded linseed could improve the productive performance and lipoprotein utilisation due to complementary effects of antimicrobial properties exert by probiotic and a rich source of bioactive compounds (e.g. ALA, lignans, antioxidants) with potential benefits on broilers gut health.
The present study aimed to assess the effects of dietary extruded linseed (ELS) and L. acidophilus (LA) addition on growth performance, carcass traits, immune organ weights, plasma lipoprotein response, and caecal bacterial populations in broiler chicks.
Materials and methods
The experimental protocol was approved by the Ethics Committee of the National Research Development Institute for Animal Biology and Nutrition, Balotesti, Romania (6283/10/2018).
Broilers and experimental design
A total of 648 one-day-old unsexed broilers (Ross 308) procured from a commercial hatchery were raised for 42 days (d) in floor pens on wood shavings litter. The lighting programme used was 23 L:1D on the first week of life, and from d 7 the light was gradually reduced until 20 L:4D, according to hybrid guide (Ross-Aviagen Citation2018). The floor pens were equipped with manual feeders and nipple drinkers’ line. The feed was offered in mash form, and birds had free access to feed and water. The birds were vaccinated against Marek’s, Newcastle and Gumboro diseases, according to standard protocol.
Broilers were assigned into six groups with six replicates each (18 birds/ pen) in a 3 × 2 factorial arrangement consisting of three extruded linseed (ELS) levels (0, 6 and 12%) without or with probiotic L. acidophilus D2/CSL 1.0 × 109 CFU g−1 (0 and 20 g ton−1 feed). The linseed (Valorex, France) used in this study was heat-treated in five steps as follow: (1) seeds were grinding and mixing with water vapours; (2) maturation (80 °C for 20 min) for the destruction of anti-nutritional factors; (3) preconditioning at 100 °C for starch gelatinisation; (4) extrusion (140 °C and 25–65 bars thermomechanical pressure) to increase the digestibility of protein and fat; (5) drying (12% moisture of final product) and cooling at 15 °C.
The three-phase (starter, grower, and finisher) diets were formulated to be isonitrogenous, isocaloric and to meet the nutrients recommended by Ross 308 manual (Table ).
Table 1. Composition and chemical analyses of the experimental diets.
The use of ELS in broilers diets, as a rich source of PUFA, led to an increase in the ALA content of the experimental diets with 4.0-fold (ELS6), respectively 6.30-fold (ELS12) and decreased the LA: ALA ratio with 4.9-fold (ELS6), respectively 9.40-fold (ELS12), compared with the control diet (Table ).
Growth performance
Body weight (BW) and feed intake (FI) were recorded for each replicate on d 1, 10, 24, and 42. The body weight gain (BWG), and feed conversion ratio (FCR) adjusted for mortality were calculated for each period (1–10 d, 11–24 d, and 25–42 d), as well as for overall period (1–42 d). The mortality rate was registered daily. Production efficiency factor (PEF) was calculated at the end of the trial (42 d), based on the following formula:
Sampling
At 42 days of age, two birds per each replicate were randomly selected, weighed, and blood was collected in heparinised tubes (4 mL/bird) from the wing vein in the morning to reduce the plasma constituent’s variability.
After blood collection, the chicks were slaughtered by cervical dislocation. Carcases were immediately plucked, eviscerated, and head, neck, shanks, abdominal fat, organs, and intestinal tract were aseptically removed. The organs were weighed, and the major parts of cut-up carcase (breast, legs, wings with skin and bone) were dissected and weight. The relative weights of cut-up carcase parts and the immune organs were calculated as the percent of live body weight at slaughter.
The caecal contents (from the left and right caeca) were aseptically collected in tubes. After the pH of intestinal content was measure in duplicate (WTW ProfiLine pH 3310, WTW GmbH, Weilheim, Germany), the tubes were stored at −80 °C until analysis.
Chemical and microbiological analyses
The proximate composition of the feeds and diets were assessed in duplicate by standardised methods (OJEU, 2009): dry matter (ISO 6496:2001), crude protein (ISO 5983-2:2009 AOAC 2001.11), crude fat (ISO 6492:2001), crude fibre (ISO 6865:2002), crude ash (ISO 2171:2010), calcium (ISO 6490-2:1983), phosphorus (spectrophotometry method). The metabolisable energy (ME) value of feed ingredients was calculated using the regression equations (National Research Council [NRC] Citation1994; Schiemann et al. Citation1972).
Amino acids profile was determined in duplicate with an HPLC Surveyor Plus Thermo Electron equipment (Massachusetts, United States) by a method described previously by Vărzaru et al. (Citation2013), which implies the acid hydrolysis for the release of amino acids from the protein molecules, preceded by oxidation with performic acid for the sulphur amino acids.
Fatty acid analyses were done in duplicate on a Perkin Elmer-Clarus 500 gas chromatograph (Massachusetts, United States) using the method described by Habeanu et al. (Citation2014).
After blood centrifugation (3000 × g for 15 min) plasma was stored at −20˚C until analysis. A dry chemistry analyser (Spotchem EZ SP-4430, Arkray Inc., Japan) and specific solid-phase reagent strips (Spotchem, Arkray Inc., Japan) were used to determine the blood metabolites (total cholesterol, TC; triglycerides, TG; glucose, Glu; total protein, TPro; albumin, Alb; total bilirubin, TBil; creatinine, Cre; uric acid, UA). The very-low-density lipoprotein–cholesterol (VLDL) were calculated based on Tietz (Citation1996) formula: VLDL-C = Triglycerides divided by 5.
The microbial populations Escherichia Coli, Staphylococcus spp., Lactobacillus spp. and Salmonella spp. in caecal content were determined on specific culture medium. Eosin Methylene Blue agar (EMB; Biokar Diagnostics, Solabia, France) was used for E. Coli enumeration, De Man Rogosa Sharpe agar (MRS; Biokar Diagnostics, Solabia, France) for lactobacilli, Mannitol salt agar (Biokar Diagnostics, Solabia, France) for staphylococci, and Rambach-Salmonella agar (Biokar Diagnostics, Solabia, France) for Salmonella spp. Briefly, one g of caecal content samples was diluted at 1:9 (wt/vol) in sterile saline solution. After 10-fold serial dilutions, one mL of each dilution was inoculated on Petri plates on specific agars. Plates were incubated at 37 °C for 24 to 48 h, and then the bacterial units were counted (Scan 300, Interscience, France). Results were expressed as log10 CFU/g of sample.
Statistical analysis
Data were analysed using the general linear model GLM procedures of SPSS software (v20.0, SPSS Citation2011) as a 3 × 2 factorial arrangement. Two-way analysis of variance (ANOVA), followed by Tukey’s multiple comparisons test, was used to assess the main effects of dietary linseed level, with or without probiotic supplementation and their interactions. The replicate pen was the experimental unit for productive performance and each sample for the other parameters. Pearson’s correlation was used to assess the relationship between indicators. The results are expressed as means and standard error of the mean (SEM). Statistical differences declared at p < .05.
Results
Chemical analyses
The proximate composition, amino acids and fatty acids profiles of extruded linseed used in this study are presented in Tables and .
Table 2. Chemical composition and amino acids profile of extruded linseed.
Table 3. Fatty acids (FA) composition of extruded linseed.
Growth performance
The results of growth performance (Table ) shown that during the overall period (1–42 d of age) the BWG, FI and FCR were not significantly affected by the ELS level nor LA supplementation. The production efficiency factor (PEF) was significantly increased by 5.9% (p = .045) as an effect of the LA addition.
Table 4. Effect of dietary extruded linseed and/or L. acidophilus on growth performanceTable Footnote1 of broilers.
The mortality rate range in normal limits (data not shown) and was not affected by dietary treatments. No interaction between ELS level and LA addition was identified regarding productive performance.
Carcass traits and immune organ weights
There was no effect of dietary extruded linseed or probiotic addition on the carcass or cut-up carcass parts yields (Table ), except the abdominal fat percentage that significantly decreases (11.60%; p = .027), respectively (9.4%; p = .035). The relative weights of immune organs were affected neither by extruded linseed level nor probiotic addition. There was no interaction between treatments.
Table 5. Effect of dietary extruded linseed and/or L. acidophilus on carcass traits and relative immune organs weightTable Footnote1 of broilers.
Plasma lipoprotein response
The plasma metabolites response (Table ) shows that the dietary ELS level significantly decreases the plasma TC (p = .003), TG, and VLDL concentrations (p = .020) compared to control diet. The dietary LA addition lowers the plasma TC (p = .015) concentration significantly. There was no effect of dietary extruded linseed level nor probiotic addition on plasma protein response. No interaction between dietary treatments was found.
Table 6. Effect of dietary extruded linseed and/or L. acidophilus on plasma lipoprotein metabolitesTable Footnote1 of broilers.
As shown in Table , a strongly positive correlation (p < .01) between dietary LA: ALA ratio and plasma TC, TG, and VLDL concentrations, and also abdominal fat percentage was observed.
Table 7. Pearson correlation between dietary LA: ALA ratio and plasma lipids and abdominal fat percentage.
Caecal bacterial populations
There was no significant effect of dietary ELS level on caecal pH and microflora at 42 days of age (Table ). The dietary probiotic addition lowers the caecal pH (6.81 vs. 6.93), Staphylococcus spp. (8.79 vs. 8.82 log10 CFU/g) and E. coli (9.17 vs. 9.21 log10 CFU/g) counts, and increase the caecal Lactobacillus spp. (12.14 vs. 11.49 log10 CFU/g) and Lactobacillus: E. coli ratio (1.32 vs. 1.25 log10 CFU/g). The samples of caecal content were negative for Salmonella spp. A significant interaction (p = .003) between dietary ELS and LA addition was observed on E. coli count (Table ).
Table 8. Effect of dietary extruded linseed and/ or L. acidophilus on caecal pH and bacterial populations (log10 CFU/g)Table Footnote1.
Discussion
The analysed chemical composition of extruded linseed used in this study have shown that it is a rich ingredient in fat (211.8 g kg−1 DM), with a favourable n − 3 PUFA (50.7%), especially ALA (50.6%). It is also a valuable protein source (182.6 g kg−1 DM) with a balanced essential amino acids profile (7.6 Lys and 10.34 TSAA g kg−1 DM). The results obtained are in line with the previous reports (Kostadinović et al. Citation2016; Ahmad et al. Citation2017; Skrivan et al. Citation2020).
In the current study, the results on the broilers productive performance shown that the extruded linseed level nor LA supplementation has no significant effects on overall BWG, FI and FCR (Table ). Anjum et al. (Citation2013) stated that BWG and FI decrease, and FCR increase in broilers fed with different level of extruded flaxseed (5, 10, 15%). Mridula et al. (Citation2015) reported that fed extruded flaxseed between 5% and 10% impaired growth performances of broiler chicks. The growth impaired was attributed to the presence in flaxseed of antinutritional compounds (e.g. cyanogenic glucosides, mucilage) and vitamin B6 antagonism (Bond et al. Citation1997; Attia Citation2003). Contrary, Kostadinović et al. (Citation2016) indicated that 10% extruded flaxseed improve broilers productive performance vs 2.5, 5% and control diets, and Skrivan et al. (Citation2020) stated that 6% extruded flaxseed increase BW of Ross 308 cockerels at 35 d compared to the control or hemp seed diets. Lactic acid bacteria have been proved to act positively by inhibiting or diminishing other contaminants into the host body. A possible explanation consists of delivering enzyme and other substance when these bacteria are passing by the intestinal tract. The present study showed that L. acidophilus addition slightly increased the BWG of broilers and resulted in significantly increases (by 5.9%) on the PEF. Previous research has shown that Lactobacillus addition as a probiotic in poultry diets positively affects growth performance (Loh et al. Citation2010; Shim et al. Citation2012; Askelson et al. Citation2014; Al-Sagan et al. Citation2020), digestive status (Kim et al. Citation2012), and stimulates an immune response (Brisbin et al. Citation2011). It is stated that factors such as nutrition, environment (hygienic condition), rearing management, probiotic dose, bird’s age, and administration method (via feed or water) could influence the probiotics efficiency (Yang et al. Citation2009).
Our results showed no significant effect of ELS level nor probiotic addition on carcase traits and relative immune organ weights (Table ), except the abdominal fat percentage that significantly decreases with 11.60% (p = .027), respectively 9.4% (p = .035). Similarly, some studies have shown that dietary flaxseed did not significantly affect the carcase traits or the relative organ weights (Pekel et al. Citation2009; Mridula et al. Citation2015). Naseem et al. (Citation2012) found that probiotics did not affect lymphoid organs. Anjum et al. (Citation2005) and Mehr et al. (Citation2007) revealed that probiotic addition decreases abdominal fat weight, while other research reported a concomitant decrease in blood triglyceride concentration (Kalavathy et al. 2003; Mansoub Citation2010). Abdominal fat could be linked by decrease activity of acetyl-CoA carboxylase, the rate-limiting enzyme in fatty acid synthesis, as the effect of probiotic addition that may also explain the blood triglycerides decreased (Santoso et al. Citation1995).
Plasma lipid metabolites are considered markers of fat metabolism in poultry organisms, and the age, sex, genetic type, environmental and feeding conditions are the major factors of influence (Krasnodębska-Depta and Koncicki Citation2000; Attia et al. Citation2020). In our study, plasma lipid metabolites were positively influenced by dietary treatments (Table ). The dietary ELS level significantly correlated with a decrease in the plasma TC, TG, and VLDL concentrations, while the LA addition significantly correlated with a decrease in the plasma TC. An explanation is that linseed contains a higher level of n − 3 PUFA, which is known to suppress lipoprotein lipase activity and regulate adipose tissue growth in broilers (Chan and Cho Citation2009). This explanation is strengthened by the significant correlation that we found in our study between dietary LA: ALA ratio and plasma lipid profile (Table ). Our previous research stated that 80 g/kg camelina cake in broiler chicks’ diet, as a rich source of n − 3 PUFA, beneficially affects plasma lipids decreasing the glucose, TC, HDL-C, and LDL-C levels, and alter fatty acids profile of lymphoid tissue (Gheorghe et al. Citation2019). Starčević et al. (Citation2014) observed that 5% of linseed oil in Ross 308 broiler diets lowered the serum cholesterol level, with no significant effect of the other lipids fraction. Present results show a significant decrease in plasma TC concentration and tend to decrease plasma TG and VLDL level, as the effect of LA addition. It has been stated that probiotic supplemented diets in broilers lower blood cholesterol (Anjum et al. Citation2005; Naseem et al. Citation2012). According to Kalavathy et al. (Citation2003), some bacterial probiotic strains can absorb cholesterol into the cells, hydrolyse bile salts or inhibit hydroxyl methylglutaryl-CoA, the rate-limiting enzyme of cholesterogenesis, and lower the cholesterol in the organism.
Modulation of gut microbiota by probiotic addition such as Lactobacillus spp. could improve health and prevent certain diseases (Mai and Draganov Citation2009) by inhibiting the proliferation of pathogenic bacteria (Van Winsen et al. Citation2002). In the present study, the L. acidophilus decreased pH and inhibited the growth of Staphylococcus spp. and E. coli populations in the caeca, concomitant with a significant increase in the Lactobacillus spp. and Lactobacillus: E. coli ratio, which is beneficial for intestinal health (Table ). The results obtained on intestinal microflora are supported by the positive effects noticed in the productive performance. To our knowledge, there are fewer studies regarding the use of L. acidophilus as a monostrain probiotic in broiler chicks. The present results are in line with other studies that noticed that supplementation with L. acidophilus via feed efficiently colonises the intestinal tract and exerts a competitive exclusion effect on pathogenic bacteria because it is part of the chickens’ healthy gut microflora (Lin et al. Citation2008; Li et al. Citation2014; Mookiah et al. Citation2014). De Cesare et al. (Citation2020) studied the effects of L. acidophilus on caecal microbiome and performance in broilers and reported similar L. acidophilus amounts in the caecal contents of broilers fed control or supplemented diet. The authors noticed a positive effect of L. acidophilus supplementation on the metabolic functions, increasing the β-glucosidase and promoting health. Forte et al. (Citation2018) observed that supplementing the Kabir chicks’ diet with L. acidophilus had no significant effect on enterococci, staphylococci, and E. coli populations. The authors reported a Lactobacillus population increased and a tendency to lower coliforms compared with the control diet. Forte et al. (Citation2016b) have shown that Lactobacillus positively modify the gastrointestinal balance of microbiota in laying hens, increasing the presence of beneficial bacteria such as Bifidobacterium spp., and reducing pathogenic bacteria such as E. coli, clostridia, and staphylococci. Contrary, other studies on the L. acidophilus use in broilers reported no significant effect on the lactobacilli population or partial effects on coliforms count (Jin et al. Citation1998; Salarmoini and Fooladi Citation2011).
Conclusions
The results indicated that the use of extruded linseed up to 12% in broiler chicks’ diets had no adverse effects on growth performance, carcass traits or immune organs size and improve the plasma lipids response due to the decreased dietary n-6: n-3 PUFA ratio. In addition, the association between extruded linseed and probiotic (L. acidophilus) positively affects the production efficiency factor, abdominal fat deposition, plasma lipid metabolites, and beneficially alters the caeca bacterial population E. coli.
Disclosure statement
No potential conflict of interest was reported by the author(s).
Additional information
Funding
References
- Ahmad S, Kamran Z, Koutoulis KC. 2017. Chapter 33. Supplemental linseed on egg production. In Hester PY, editor. Egg innovations and strategies for improvements. West Lafayette (IN): Purdue University. p. 349–363.
- Al-Sagan AA, Al-Yemni AH, Al-Abdullatif AA, Attia YA, Hussein EOS. 2020. Effects of different dietary levels of blue lupine (Lupinus angustifolius) seed meal with or without probiotics on the performance, carcass criteria, immune organs, and gut morphology of broiler chickens. Front Vet Sci. 7:124.
- Alzueta C, Rodríguez ML, Cutuli MT, Rebolé A, Ortiz LT, Centeno C, Treviño J. 2003. Effect of whole and demucilaged linseed in broiler chicken diets on digesta viscosity, nutrient utilisation and intestinal microflora. Br Poult Sci. 44(1):67–74.
- Anjum FM, Haider MF, Khan MI, Sohaib M, Arshad MS. 2013. Impact of extruded flaxseed meal supplemented diet on growth performance, oxidative stability and quality of broiler meat and meat products. Lipids Health Dis. 12:13.
- Anjum MI, Khan AG, Azim A, Afzal M. 2005. Effect of dietary supplementation of multi-strain probiotic on broiler growth performance. Pak Vet J. 25:25–29.
- Apperson KD, Cherian G. 2017. Effect of whole flax seed and carbohydrase enzymes on gastrointestinal morphology, muscle fatty acids, and production performance in broiler chickens. Poult Sci. 96(5):1228–1234.
- Askelson TE, Campasino A, Lee JT, Duong T. 2014. Evaluation of phytate-degrading Lactobacillus culture administration to broiler chickens. Appl Environ Microbiol. 80(3):943–950.
- Attia YA. 2003. Nutritional values of soaked linseed cake and its inclusion in finishing diets for male broiler chicks a source of protein and n-3 fatty acids. Egypt Poultry Sci J. 23:739–759.
- Attia YA, Al-Harthi MA, Abo El-Maaty HM. 2020. The effects of different oil sources on performance, digestive enzymes, carcass traits, biochemical, immunological, antioxidant, and morphometric responses of broiler chicks. Front Vet Sci. 7:181.
- Attia YA, Al-Harthi MA, El-Shafey AS, Rehab YA, Kim WK. 2017. Enhancing tolerance of broiler chickens to heat stress by addition with vitamin E, vitamin C and/or probiotics. Ann Anim Sci. 17:1–15.
- Bautista-Ortega J, Goeger DE, Cherian G. 2009. Egg yolk n-6 and n-3 fatty acids modify tissue lipid components, antioxidant status, and ex vivo eicosanoid production in chick cardiac tissue. Poult Sci. 88(6):1167–1175.
- Betti M, Perez TI, Zuidhof MJ, Renema RA. 2009. Omega-3-enriched broiler meat: 3. Fatty acid distribution between triacylglycerol and phospholipid classes. Poult Sci. 88(8):1740–1754.
- Bhatty RS. 1995. Nutritional composition of whole flaxseed and flaxseed meal. In Cunnane SC, Thompson LH, editors. Flaxseed in human nutrition. Champaign (IL): AOCS Press. p. 22–45.
- Bianchi SB, Camaschella P, Lavezzari D. 1985. Les lactobacilles specifiques du poulet. Leur influence sur la microflore du tube digestif. Microbiol Aliment Nutr. 3:73–82.
- Blok MC, Dekker RA. 2017. Table ‘Standardized ileal digestibility of amino acids in feedstuffs for poultry’. Wageningen Livestock Research. p. 31–35.
- Bond JM, Julian RJ, Squires EJ. 1997. Effect of dietary flaxseed on broiler growth, erythrocyte deformability, and fatty acid composition of erythrocyte membranes. Can J Anim Sci. 77(2):279–286.
- Brisbin JT, Gong J, Orouji S, Esufali J, Mallick AI, Parvizi P, Shewen PE, Sharif S. 2011. Oral treatment of chickens with lactobacilli influences elicitation of immune responses. Clin Vaccine Immunol. 18(9):1447–1455.
- Cesari V, Mangiagalli MG, Giardini A, Galimberti P, Carteri S, Gallazzi D, Toschi I. 2014. Egg quality and productive performance of laying hens fed different levels of skimmed milk powder added to a diet containing Lactobacillus acidophilus. Poult Sci. 93(5):1197–1201.
- Chan EJ, Cho L. 2009. What can we expect from omega-3 fatty acids? Cleve Clin J Med. 76(4):245–251.
- Dale N, Batal A. 2008. Feedstuffs reference issue and buyers guide. Feedstuffs. 79:17–20.
- De Cesare A, Sala C, Castellani G, Astolfi A, Indio V, Giardini A, Manfreda G. 2020. Effect of Lactobacillus acidophilus D2/CSL (CECT 4529) supplementation in drinking water on chicken crop and caeca microbiome. PLos One. 15(1):e0228338.
- De Cesare A, Sirri F, Manfreda G, Moniaci P, Giardini A, Zampiga M, Meluzzi A. 2017. Effect of dietary supplementation with Lactobacillus acidophilus D2/CSL (CECT 4529) on caecum microbioma and productive performance in broiler chickens. PLoS One. 12(5):e0176309.
- Forte C, Acuti G, Manuali E, Casagrande Proietti P, Pavone S, Trabalza-Marinucci M, Moscati L, Onofri A, Lorenzetti C, Franciosini MP. 2016b. Effects of two different probiotics on microflora, morphology, and morphometry of gut in organic laying hens. Poult Sci. 95(11):2528–2535.
- Forte C, Manuali E, Abbate Y, Papa P, Vieceli L, Tentellini M, Trabalza-Marinucci M, Moscati L. 2018. Dietary Lactobacillus acidophilus positively influences growth performance, gut morphology, and gut microbiology in rurally reared chickens. Poult Sci. 97(3):930–936.
- Forte C, Moscati L, Acuti G, Mugnai C, Franciosini MP, Costarelli S, Cobellis G, Trabalza-Marinucci M. 2016a. Effects of dietary Lactobacillus acidophilus and Bacillus subtilis on laying performance, egg quality, blood biochemistry and immune response of organic laying hens. J Anim Physiol Anim Nutr. 100(5):977–987.
- Gallazzi D, Giardini A, Mangiagalli MG, Marelli S, Ferrazzi V, Orsi C, Cavalchini LG. 2008. Effects of Lactobacillus acidophilus D2/CSL on laying hen performance. Ital J Anim Sci. 7(1):27–37.
- Gheorghe A, Hăbeanu M, Lefter NA, Ropotă M. 2019. Performance parameters, plasma lipid status, and lymphoid tissue fatty acid profile of broiler chicks fed camelina cake. Braz J Poultry Sci. 21(4):001–008.
- Gonzalez D, Mustacich DJ, Traber MG, Cherian G. 2011. Early feeding and dietary lipids affect broiler tissue fatty acids, vitamin E status, and cyclooxygenase-2 protein expression upon lipopolysaccharide challenge. Poult Sci. 90(12):2790–2800.
- Habeanu M, Thomas A, Bispo E, Gobert M, Gruffat D, Durand D, Bauchart D. 2014. Extruded linseed and rapeseed both influenced fatty acid composition of total lipids and their polar and neutral fractions in Longissimus thoracis and Semitendinosus muscles of finishing Normand cows. Meat Sci. 96(1):99–106.
- Huyghebaert G, Ducatelle R, Van Immerseel F. 2011. An update on alternatives to antimicrobial growth promoters for broilers. Vet J. 187(2):182–188.
- Jin LZ, Ho YW, Abdullah N, Ali MA, Jalaludin S. 1998. Effects of adherent Lactobacillus cultures on growth, weight of organs and intestinal microflora and volatile fatty acids in broilers. Anim Feed Sci Tech. 70(3):197–209.
- Kalavathy R, Abdullah N, Jalaludin S, Ho YW. 2003. Effects of Lactobacillus cultures on growth performance, abdominal fat deposition, serum lipids and weight of organs of broiler chickens. Br Poult Sci. 44(1):139–144.
- Kim JS, Ingale SL, Kim YW, Kim KH, Sen S, Ryu MH, Lohakare JD, Kwon IK, Chae BJ. 2012. Effect of supplementation of multi-microbe probiotic product on growth performance, apparent digestibility, cecal microbiota and small intestinal morphology of broilers. J Anim Physiol Anim Nutr. 96(4):618–626.
- Kostadinović L, Popović S, Čolović D, Vukmirović Đ, Tasić T, Puvača N, Lević J. 2016. Effect of extruded flaxseed in broiler diets on blood oxidative stability and meat fatty acid composition. Eur Poult Sci. 80: 1–14.
- Krasnodębska-Depta A, Koncicki A. 2000. Physiological values of selected serum biochemical indices in broiler chickens. Med Weter. 56:456–460.
- Li Y, Xu Q, Yang C, Yang X, Lv L, Yin C, Liu X, Yan H. 2014. Effects of probiotics on the growth performance and intestinal microflora of broiler chickens. Pak J Pharm Sci. 27(3 Suppl):713–717.
- Lin CK, Tsai HC, Lin PP, Tsen HY, Tsai CC. 2008. Lactobacillus acidophilus LAP5 able to inhibit the Salmonella choleraesuis invasion to the human Caco-2 epithelial cell. Anaerobe. 14(5):251–255.
- Loh TC, Thanh NT, Foo HL, Hair-Bejo M, Azhar BK. 2010. Feeding of different levels of metabolite combinations produced by Lactobacillus plantarum on growth performance, fecal microflora, volatile fatty acids and villi height in broilers. Anim Sci J. 81(2):205–214.
- Mai V, Draganov PV. 2009. Recent advances and remaining gaps in our knowledge of associations between gut microbiota and human health. World J Gastroenterol. 15(1):81–85.
- Mansoub NH. 2010. Effect of probiotic bacteria utilization on serum cholesterol and triglycerides contents and performance of broiler chickens. Glob Vet. 5:184–186.
- Mehr MA, Shargh MS, Dastar B, Hassani S, Akbari MR. 2007. Effect of different levels of protein and protexin on broiler performance. Int J Poultry Sci. 6(8):573–577.
- Mookiah S, Sieo CC, Ramasamy K, Abdullah N, Ho YW. 2014. Effects of dietary prebiotics, probiotic and synbiotics on performance, caecal bacterial populations and caecal fermentation concentrations of broiler chickens. J Sci Food Agric. 94(2):341–348.
- Mountzouris KC, Tsirtsikos P, Kalamara E, Nitsch S, Schatzmayr G, Fegeros K. 2007. Evaluation of the efficacy of a probiotic containing Lactobacillus, Bifidobacterium, Enterococcus, and Pediococcus strains in promoting broiler performance and modulating cecal microflora composition and metabolic activities. Poult Sci. 86(2):309–317.
- Mridula D, Kaur D, Nagra SS, Barnwal P, Gurumayum S, Singh KK. 2015. Growth performance and quality characteristics of flaxseed-fed broiler chicks. J Appl Anim Res. 43(3):345–351.
- Naseem S, Rahman SU, Shafee M, Sheikh AA, Khan A. 2012. Immunomodulatory and growth-promoting effect of a probiotic supplemented in the feed of broiler chicks vaccinated against infectious bursal disease. Rev Bras Cienc Avic. 14(2):109–113.
- National Research Council (NRC). 1994. Nutrient requirements of poultry. 9th rev ed. Washington (DC): The National Academies Press.
- Patterson JA, Burkholder KM. 2003. Application of prebiotics and probiotics in poultry production. Poult Sci. 82(4):627–631.
- Pekel AY, Patterson PH, Hulet RM, Acar N, Cravener TL, Dowler DB, Hunter JM. 2009. Dietary camelina meal versus flaxseed with and without supplemental copper for broiler chickens: live performance and processing yield. Poult Sci. 88(11):2392–2398.
- Pirmohammadi A, Khalaji S, Yari M. 2019. Effects of linseed expansion on molecular structures of diet, digestive enzymes activity, serum metabolites and ileal morphology. J Appl Poult Res. 28(4):997–1012.
- Rodriguez ML, Alzueta C, Rebole A, Ortiz LT, Centeno C, Trevino J. 2001. Effect of inclusion level of linseed on the nutrient utilisation of diets for growing broiler chickens. Br Poult Sci. 42(3):368–375.
- Ross-Aviagen. 2018. Ross Broiler management handbook. viagen US, 1118-AVNR-032. www.aviagen.co
- Salarmoini M, Fooladi MH. 2011. Efficacy of Lactobacillus acidophilus as probiotic to improve broiler chick’s performance. J Agric Sci Tech. 13:165–172.
- Santoso U, Tanaka K, Ohtani S. 1995. Effect of dried Bacillus subtilis culture on growth, body composition and hepatic lipogenic enzyme activity in female broiler chicks. Br J Nutr. 74(4):523–529.
- Schiemann R, Nehring K, Hoffmann L, Jentsch W, Chudy A. 1972. Energetische Futterbevertung und Energienormen. Berlin: VEB Deutscher Landwirtschatsverlag. p. 344.
- Shim YH, Ingale SL, Kim JS, Kim KH, Seo DK, Lee SC, Chae BJ, Kwon IK. 2012. A multi-microbe probiotic formulation processed at low and high drying temperatures: effects on growth performance, nutrient retention and caecal microbiology of broilers. Br Poult Sci. 53(4):482–490.
- Singh KK, Mridula D, Rehal J, Barnwal P. 2011. Flaxseed: a potential source of food, feed and fiber. Crit Rev Food Sci Nutr. 51(3):210–222.
- Skrivan M, Englmaierová M, Taubner T, Skrivanová E. 2020. Effects of dietary hemp seed and flaxseed on growth performance, meat fatty acid compositions, liver tocopherol concentration and bone strength of cockerels. Animals. 10(3):458.
- Smith JM. 2014. A review of avian probiotics. J Avian Med Surg. 28(2):87–94.
- SPSS. 2011. Statistics version 20.0. Armonk (NY): IBM SPSS Inc.
- Sridhar K, Nagireddy NK, Panda AK, Jatoth N, Thirunahari R, Vangoor RR. 2017. Effect of dietary incorporation of n-3 polyunsaturated fatty acids rich oil sources on fatty acid profile, keeping quality and sensory attributes of broiler chicken meat. Anim Nutr. 3:386–391.
- Starčević K, Mašek T, Brozić D, Filipović N, Stojević Z. 2014. Growth performance, serum lipids and fatty acid profile of different tissues in chicken broilers fed a diet supplemented with linseed oil during a prolonged fattening period. Vet Arhiv. 84(1):75–84.
- Tietz NW. 1996. Fundamentals of clinical chemistry. 4th ed. Philadelphia (PA): W.B. Saunders Company.
- Van Winsen RL, Keuzenkamp D, Urlings BA, Lipman LJ, Snijders JA, Verheijden JH, van Knapen F. 2002. Effect of fermented feed on shedding of Enterobacteriaceae by fattening pigs. Vet Microbiol. 87(3):267–276.
- Vărzaru I, Untea AE, Martura T, Olteanu M, Panaite TD, Schitea M, Van I. 2013. Development and validation of an RP-HPLC method for methionine, cysteine and lysine separation and determination in corn samples. Rev Chim (Bucharest). 64(7):673–679.
- Yang Y, Iji PA, Choct M. 2009. Dietary modulation of gut microflora in broiler chickens: a review of the role of six kinds of alternatives to in-feed antibiotics. World’s Poult Sci J. 65(1):97–114.