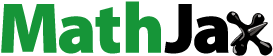
Abstract
This study evaluated the effect of dietary calcium (Ca) and available phosphorus (aP) restriction on growth performance, nutrients retention (ATTR), serum metabolites, and tibia in broiler chickens. A total of 720 one-day-old Ross-308 broilers were used in this study. Broilers were fed with 0 (control), 10 (L1), 20 (L2), and 30% (L3) aP-deficient starting (ST) diets during 1–10 days. In ST period, control included 6 and others included 18 replicates of 12 chicks. In post-starter (PST) period, control was still fed with standard diets, while restricted groups were divided into 3 groups and fed with L1, L2, and L3 diets. Each PST treatment included 6 replicates of 12 chicks. Data were analysed using a completely randomised design in a 1 (control)+3 × 3 factorial arrangement. Factors included aP levels in ST and PST diets. Results showed that L3 diet decreased feed intake and weight gain but increased ATTR of Ca in starter phase in compare with control group (p<.05). Retarded growth of birds was compensated in post-starter phase. The Ca, P and ALP levels in blood serum were not significantly influenced by aP levels on day 10 and day 42. The ATTR of Ca and tP on day 42, were higher in birds fed L3 diets than L2 or L1 groups (p<.05). The main effect of L3 group in both ST and PST, decreased (p<.05) tibia ash, Ca, and P in compare with L1 group. Main effect of post-starter L3 group had lowest femur breaking strength (p=.007). In general, restriction in dietary aP increased nutrients ATTR, impaired bone mineralisation and strength without affecting growth performance.
Phosphorus is one of the most important environmental pollutants that is excreted through broilers manure.
Birds exposed to aP and Ca restriction increased the retention of these minerals.
Dietary aP and Ca restriction impaired bone mineralisation and strength.
HIGHLIGHTS
Introduction
Calcium and P are two main macro-minerals with essential structural and physiological functions in the animal body. Poultry require Ca and P for proper bone development, muscle functionality, enzyme activity, and normal metabolism (Underwood and Suttle, Citation1999). Some of the Ca and P requirements are met by feed ingredients incorporated in the diet; then, inorganic Ca and P supplements are added to the diet to thoroughly fulfil the requirements.
The high inclusion levels of inorganic P in poultry diets contribute to increased feed cost and environmental pollution due to excess P excretion (Delezie et al. Citation2015; Gautier et al. Citation2017). On the other hand, high dietary Ca levels increase digesta pH leading to decreased digestibility of lipids and other nutrients. A mild reduction in dietary Ca level may enhance the P and other nutrients bioavailability (Mutucumarana et al. Citation2014).
Dietary P is not fully digested and utilised by poultry species due to the insufficient production of endogenous phosphatases in their digestive tract. There are evidences that broilers may develop adaptive mechanisms when exposed to available phosphorus (aP) deficiency during early age (Ashwell and Angel Citation2010; Proszkowiec-Weglarz and Angel Citation2013).
Feeding birds with a P-deficient diet for 90 h post-hatch resulted in improved utilisation of dietary P later in life. This improvement in P retention could be partly attributed to a long-lasting increase in gene expression of the intestine-specific Na/P cotransporter during the early P deprivation as well as later in life (Ashwell and Angel Citation2010). Then, starting diets low in P may develop specific adaptive alterations in birds that help them use P more efficiently even during growing and finishing periods. However, there is little information on the levels of early and later P deficiencies by which the compensatory pathways can be induced efficiently in broilers.
Rousseau et al. (Citation2016) reported that broilers are able to adapt to the early life deficiency of dietary P and Ca by improving digestion efficiency in the next periods, and the amount of compensation for bone mineralisation and growth performance depends on the amount of P and Ca in the post-starter diets.
It has been reported that aP levels in diets of modern broiler chickens can be reduced by 20% (Delezie et al. Citation2015). The current study aimed to evaluate broiler chickens’ responses to different dietary Ca and aP restriction regimens, with constant Ca: aP ratio of 2:1, during starter and post-starter periods. The evaluated variables were included growth performance, carcase traits, nutrients apparent total tract retention, serum metabolites, and tibia bone properties.
Materials and methods
This study was approved by the Animal Care and Use Committee of the Ferdowsi University of Mashhad, Mashhad, Iran.
Birds, diets, and housing
Seven hundred and twenty 1-day-old straight run Ross-308 broiler chicks with average body weight (BW) of 42.54 ± 0.87 g were randomly distributed into sixty 1 × 1 m2 floor pens with wood shavings as bedding material. Each pen served as a replicate unit, and the 60 pens were allocated into four starting dietary treatments as follows: 1) a standard diet containing recommended levels of Ca and aP (Control); 2) a diet with 10% Ca and aP restriction; 3) a diet with 20% Ca and aP restriction; and 4) a diet with 30% Ca and aP restriction. During the starter period, the control treatment included 6 and other treatments included 18 replicates of 12 chicks each, respectively. At the end of the day 10, total live BW and residual feed weight of each pen were measured. Then each starting Ca and aP deficient group (all starting groups except the control) was divided into three new groups which were reared on one of the 10%, 20% or 30% post-starter Ca and aP restriction regimens until 42 days of age. The control group fed standard growing and finishing diets with no restriction. Accordingly, ten Ca and aP regimens were applied in this study including the control, L1(ST)L1(PST), L1L2, L1L3, L2L1, L2L2, L2L3, L3L1, L3L2, and L3L3 regimens, accordingly. During the post-starter period, each treatment included 6 replicates of 12 chicks each. By the decreasing levels of Ca and aP in the starting diets, we sought to stimulate the adaptation mechanism of broilers and distinguish how much reduction could induce a more adaptive response. With different aP levels in the post-starter period, we aimed to know the possible reduction level of dietary P in response to Ca and aP restriction of starter period. The calcium to aP ratio was kept constant in all experimental diets. The experimental design of the dietary treatments is shown in Table . Diets used in each feeding phase differed only in Ca and aP levels, and all other nutrients were provided following the nutrition specifications of Ross-308 broilers (Aviagen Citation2014b). For each phase, two diets were prepared, one of which contained recommended levels of Ca and aP and the other contained the lowest evaluated level of the Ca and aP (70% of recommendation level). Then limestone and dicalcium phosphate were used instead of sand to obtain different levels of Ca and aP in the diets (Table ).
Table 1. Experimental design of the dietary treatments.
Table 2. Composition of experimental diets, as fed basis.
During the first four days of the rearing period, each pen contained a tray feeder along with a plastic day-old chick drinker, and then a manual type plastic feeder and two nipple drinkers were used per pen. House temperature was set at 32 °C at the time of replacement and then decreased by 3 °C weakly until 21 °C is reached. Relative humidity was kept between 65–75% and 50–60% from 1 to 7 days and 8 to 42 days, respectively. All birds were provided with 24 h light during the first two days, followed by 18 h light: 6 h dark thereafter. Other rearing conditions were managed according to the Ross-308 management handbook (Aviagen Citation2014a).
Growth performance
At the end of the starting (10 days of age), growing (24 days of age), and finishing (42 days of age) phases, collective live weight and residual feed weight of each pen was measured to determine average BW, daily WG and daily FI. Body weight of dead birds and the number of days that the dead birds spent in the pen were recorded to calculate hen-day (HD) and mortality-corrected FCR. Following formulae were used to determine WG, HD, FI, and FCR of a unit during a given period:
where BW1 and BW2 represent BW of birds in the unit at the beginning and end of the period, respectively.
Blood metabolites
Blood samples were collected from the jugular vein at the moment of decapitation. Serum was separated and analysed for Ca and P content (ICP-OES; Spectro Arcos System, Germany, model 76004555) as described by Leske and Coon (Citation2002). The alkaline phosphatase (ALP) activity was assessed using a commercially available kit (Pars Azmon, Tehran, Iran) according to the DGCK Method (The German Societies for Clinical Chemistry).
Tibia and femur characteristics
The left tibias were removed and de-flashed, defatted by soaking in ethyl alcohol for 48 hours, and again in ethyl ether for 48 h (Hamdi et al. Citation2015b). The tibias were then dried to a constant weight using a drying oven at 105 °C for 24 hours. Then they were ashed in a muffle furnace at 550 °C for 12 h (Hamdi et al. Citation2015a). The remaining ash was used to determine tibia Ca and P contents by employing the ICP-OES method. The right femur was removed, de-fleshed, and the breaking strength was measured by the Instron Universal Testing Machine (Model H5KS, Tinius Olsen Company) (Mirakzehi et al. Citation2013).
Apparent total tract retention of nutrients
On day 6 and day 36 of the experiment, 15 and 10 male birds from each treatment group were weighed and transferred into 5 metabolic cages, respectively. After 2 days of adaptation, the birds were exposed to a 12 h fasting period. Then the chicks were refed for three days, followed by another 12 h fasting period. Excreted materials were collected quantitatively twice per day and stored immediately in −18 °C until further analysis. Excreta collection was performed from the beginning of the refeeding period to the end of the second fasting period. The amount of consumed feed was recorded for each metabolic cage. Feed samples and faecal materials were dried using a forced air oven at 55 °C for 48 h and then ground through a blade grinder and weighed quantitatively to calculate collective dry matter (DM) intake and DM excretion. All samples were analysed for total nitrogen (TN) using the Kjeldahl method, according to AOAC international (2005; Method 2001.11). Then, the proportion of crude protein (CP) in each sample was obtained by multiplying the associated TN value by 6.25. Inductively coupled plasma optical emission spectroscopy (ICP-OES; Spectro Arcos System, Germany, model 76004555), was used to determine dietary and faecal Ca and total P contents as described by Leske and Coon (Citation2002). Finally, nutrients ATTR were calculated according to the following formula (Mutucumarana et al. Citation2014):
Carcase traits
On day 42 of age, one male bird per pen was selected, weighed, and decapitated for measuring carcase yield. Moreover, breast, thigh, and neck-back-wing parts were weighed separately along with liver, spleen, bursa of Fabricius, and abdominal fat pad. The results were expressed as a percent of live weight.
Statistical analysis
The data were analysed using the GLM procedure of SAS (Citation2012) software in a completely randomised design for variance analysis, the means were compared by Tukey’s test (p < .05). Then the control group was excluded, and the data were analysed as a 3 × 3 factorial with 3 dietary levels of Ca and aP in starter phase and 3 dietary levels of these minerals in the post-starter period. The levels-response effect of Ca and aP was computed using orthogonal polynomial contrasts (linear and quadratic). Orthogonal contrast was used to determine the mean comparison among treatments (control vs. other treatments).
Results
Growth performance
The results of growth performance in broiler chickens during starter (1–10 days), grower (11–24 days), finisher (25–42 days), and post-starter (11–42 days) periods are shown in Tables and . During the starter phase, BW, WG, and FI in the L3 group were significantly lower than that of the control group (p < .05). The BW, WG, and FI were linearly decreased (p < .05) with decreasing levels of dietary aP in the starter phase. Likewise, decreasing dietary aP concentrations linearly decreased BW, WG, and FCR during grower phase (Table ). Whereas, growth performance traits in other phases were not significantly different. The WG, FI, and FCR were not significantly affected by reducing levels of dietary aP during finisher and post-starter periods (Table ). The FCR in L2L2 group on day 11–24 was the lowest, and it was significantly lower than the control group (p = .02). Comparing the main effects of ST and PST regimens revealed that BW, WG, and FI were not influenced by reducing aP levels during finisher, and post-starter periods. But, the FCR was improved in the birds fed with L3 diets compared with the birds fed with L1 or L2 diet during 25–42 days (p < .01). A 2-way interaction between ST and PST for FI was observed (Figure ). Feed consumption in the L3L3 group was significantly lower than other groups during the finisher phase (p = .015). Also, orthogonal contrast between control and aP reduced diets showed that there were no significant differences in regard to BW, WG, FI, and FCR in grower, finisher, and post-starter periods (Table ).
Figure 1. The interaction between starter and post-starter regimens on FI during finisher phase. Dietary Ca and available phosphorus (aP) restriction regimens (left two digits represent restriction rates during the starter phase while right digits represent restriction rates during the post-starter period).
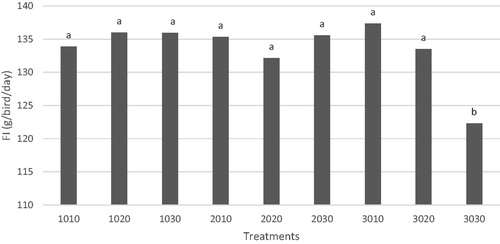
Table 3. Effect of different dietary levels of calcium and available phosphorus on growth performance, nutrients retention, and serum metabolites in broiler chickens (1–10 days).
Table 4. Effect of different dietary levels of calcium and available phosphorus (aP) on growth performance in broiler chickens.
Blood metabolites and apparent total tract retention of nutrients
The Ca and P concentrations and the ALP activity in blood serum of broiler chicks were not significantly influenced by reduced dietary aP levels on day 10 (Table ) and day 42 (Table ). Neither aP levels significantly influenced the blood serum metabolites in ST and PST regimens nor their interaction.
Table 5. Effect of different dietary levels of calcium and available phosphorus (aP) on blood metabolites, nutrients retention, and tibia and femur traits in broilers chickens.
Decreasing dietary aP levels did not significantly affect the ATTR of tP, DM, and CP at the starter phase (p > .05). But the ATTR of Ca was significantly increased (p = .0336) in birds fed with L3 diet compared with the birds fed L1 diet and those fed control diet during 1–10 days (Table ). The main effects of ST and PST had no significant effect on ATTR of DM, CP, Ca, and tP on 42 days of age (Table ). But the ATTR of Ca and tP on day 42 of age were significantly influenced by reduced dietary aP of PST diets. Retention of Ca and tP was greater (p = .004 and p = .0002, respectively) for birds fed 30% reduction of aP when compared with 20% group or 10% group at 42 days. Also, ATTR of Ca and P were linearly increased when the aP levels decreased in the diets (Lin, p = .0008 and p < .0001, respectively). The orthogonal contrast between control and aP reduced diets showed that ATTR of Ca and P in low-aP groups were significantly greater (p < .05) than the control group (Table ).
Tibia and femur characteristics
The influence of reduced dietary aP during ST, and PST periods and, their interaction on tibia traits on day 42 of age in broiler chickens are presented in Table . The results showed that ST and PST regimens significantly affected the tibia ash, Ca, and P contents in the finisher phase. The main effect of starter L3 diets (30% reduction in dietary Ca and aP) significantly decreased the tibia ash, Ca, and P contents (p = .001, p = .021 and p = .003, respectively) when compared with L1 group. Likewise, the main effect of post-starter L3 group had lower tibia ash content (p = .036), tibia Ca (p < .001), tibia P (p < .001), and femur breaking strength (p = .007) in compare with the L1 group. Also, tibia ash, Ca, P contents, and the femur breaking strength were linearly decreased by decreasing dietary aP levels.
Additionally, tibia ash, Ca, and P contents and the femur breaking strength in aP-deficient groups were significantly lower than in the control group (control vs. other groups). A 2-way interaction between ST and PST for tibia Ca was observed (Figure ). The groups L2L3, L3L2, and L3L3 had the lowest tibia Ca compared with other groups (p = .0010).
Figure 2. The interaction between starter and post-starter regimens on tibia calcium content on day 42. Dietary Ca and available phosphorus (aP) restriction regimens (left two digits represent restriction rates during the starter phase while right digits represent restriction rate during the post-starter period).
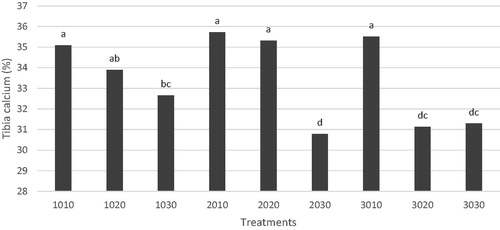
Carcase traits
Based on the results presented in Table , the effect of the model (simple completely randomised design) or the effect of starter and post-starter regimens and their interaction had no significant (p > .05) effect on carcase traits and internal organs in broiler chickens on day 42 of age. By comparing with control groups, reduction of dietary available phosphorus (aP) did not affect (p > .05) carcase parts and internal organs of broiler chickens (control vs. other treatments). Also, with decreasing aP levels, no linear effect was observed on carcase parts and internal organs.
Table 6. Effect of different dietary levels of calcium and available phosphorus (aP) on carcase traits and internal organs in broiler chickens.
Discussion
Growth performance
The adverse effects of dietary aP deficiency was very clear on growth performance during the starter phase, but these effects were disappeared in the later phases. In the present study, growth performance was not influenced by decreased dietary aP during the finisher and post-starter periods. These results are in agreement with the findings of Yan et al. (Citation2005) who reported that the birds fed the low Ca and P diet (0.30% nPP and 0.6% Ca) had weighed less (p < .05) than those fed the control diet (0.45% nPP and 0.9% Ca) at 18 days of age; however at 28 and 32 days no significant differences were observed regarding to BW among the treatments. Birds fed with diet containing 0.6% Ca and 0.30% nPP had similar growth performance in compare to those fed 1.0% Ca and 0.45% nPP diet (Rousseau et al. Citation2016). In another study, reducing dietary aP levels did not significantly affect BW, WG, FI, and FCR from 1 to 42 days of age (Farhadi et al. Citation2017).
The disappearance the negative effects of aP deficiency on growth in the later phases, may be due to the birds’ ability to adapt to this deficiency. Modern broilers showed a high adaptive capacity when fed with diets containing low Ca or P in the early age (Yan et al. Citation2005). Increasing nutrient digestibility may be one of the adaptive responses. Mitchell and Edwards Jr (1996) reported that the reduced mineral content of diets resulted in higher apparent retention of Ca and tP. The growth performance and nutrient retention were better in birds fed with diet containing (0.6% Ca) compared with birds receiving more than 0.6% Ca with constant dietary nPP level at 0.30% (Gautier et al. Citation2017). Additionally, the true ileal P digestibility and true P retention were highest (p < .05) in birds that fed with diets containing 0.13% Ca compared with birds fed diets formulated with 0.95% Ca (Perryman et al. Citation2016). Also, Abudabos (Citation2012) reported that the intestinal phytase activity was increased with decreased inorganic P in the diets.
Blood metabolites and apparent total tract retention of nutrients
The Ca and P concentrations and activity of the ALP in blood serum of broiler chicks were not significantly influenced by reduced dietary aP on day 10 and day 42 (Tables and ). These results agree with the findings of Kheiri and Rahmani (Citation2006), who reported that serum Ca and P concentrations were not influenced by reduced dietary aP level. In contrast, Bar et al. (Citation2003) reported that reduced dietary Ca from 10.3 g/kg to 1.9 g/kg diet during 1–11 days of age led to hypocalcaemia. The different responses of serum Ca and P concentrations may be related to the severity of the deficiency.
In the current study, reducing dietary aP levels led to an increase in the ATTR of Ca and P in both day 10 and day 42 of age. With this adaptation mechanism, the birds tried to compensate for the dietary deficiency of P and Ca. These results were supported by findings of Mitchell and Edwards Jr (1996), who showed that the reduced mineral content of diets resulted in higher apparent retention of Ca and P. Reduced levels of inorganic Ca in the diet improved phytate-P availability (Selle et al. Citation2009). Similarly, the apparent digestibility of Ca, and P was linearly increased when the dietary levels of Ca decreased from 1.16 to 0.47% with constant dietary P level at 0.34% (Plumstead et al. Citation2008). Increased Ca and P retention in the current study may be due to the development of the adaptive mechanisms which include, synthesis of 1,25-dihydroxycholecalciferol, plasma membrane Ca-transporting, calbindin expression, sodium-calcium exchanger, plasma membrane Ca2+ ATPase (PMCA), calcium pumps, sodium-dependent phosphate transport protein 2B that increase the availability of the minerals. Adedokun and Adeola (Citation2013) reported that the increase in the synthesis of 1,25-dihydroxycholecalciferol enhances intestinal Ca absorption. Additionally, previous studies have shown that the adaptation of chickens to a P-deficient diet resulted in an increase in the net synthesis of the duodenal plasma membrane Ca-transporting, plasma membrane Ca2+ ATPase and its mRNA (Cai et al. Citation1993). Centeno et al. (Citation2004) demonstrated that a low Ca diet increases sodium-calcium exchanger activity in chick enterocytes.
Tibia and femur characteristics
In the current study, reduced dietary aP levels led to a decrease femur breaking strength and ash, Ca, and P contents in the tibia. This decrease was more pronounced in birds fed with post-starter diets that had a greater decrease in aP and Ca levels. The birds fed diets with 10% aP deficiency in the post-starter period, regardless of the aP restriction level in the starter phase, had no significant difference with the control treatment. In other words, birds fed with diets containing 20 and 30% aP and Ca restriction in the post-starter period, could not compensate the deficiency of these minerals. This indicates that the dietary aP and Ca concentrations can be reduced by 30% in the starter period, but the reduction of these minerals in the post-starter period diets by more than 10% is not appropriate.
These results are in agreement with the findings of Onyango et al. (Citation2003), who found that bone mineral content and ash percentage were linearly decreased as the level of dietary Ca decreased from 0.91% to 0.45%. Mondal et al. (Citation2007) indicated that the tibia ash, Ca, and P were decreased in the birds fed low-P diets (0.30% nPP) compared with the control diet (0.46% nPP). Similarly, the tibia ash was decreased by reduced dietary P level from 3.5 g/kg to 2.5 g/kg in the diet (Wilkinson et al. Citation2014). The decrease in tibia ash, Ca, and P, probably due to increase in parathyroid (PTH) and 1,25(OH)2D3 hormones level, promotes the transfer of Ca and P from the bones to the blood. Dittmer and Thompson (Citation2011) showed that the 1,25(OH)2D3, together with PTH, promotes mobilisation of Ca from bones to maintain constant blood Ca concentration. The 1,25(OH)2D3 hormone stimulates osteoclastogenesis, resorption of bone, and mobilisation of Ca (Kitazawa et al. Citation2003). Similarly, PTH increases the Ca resorption from the bones by enhancing the 1,25-(OH)2D3 production (de Matos Citation2008).
Carcase traits
Lowering dietary aP did not show a significant effect on carcase parts and internal organs in broilers chickens. The present study findings are in agreement with published reports in this field. Han et al. (Citation2015) reported that nPP levels did not significantly influence the carcase, breast, and legs relative weight. Likewise, Akter et al. (Citation2016) pointed out that the relative weight of birds’ internal organs were not impacted by reduced Ca and nPP from 10 g/kg Ca and 4 g/kg nPP to 6 g/kg Ca and 3 g/kg nPP. Similar results have been reported by Abdulla et al. (Citation2017), who observed that Ca levels did not affect yield, leg, and breast percentages. In another study, the carcase, breast, drumstick, and thigh yields were not influenced by the reduction of Ca up to 30% of the broilers’ requirements (Tizziani et al. Citation2019). In the current study, meat quality was not studied and can be considered in future investigations.
In the current study, we hypothesised that, by reducing the dietary aP level by 10, 20, and 30% of the standard in the starter phase, adaptive responses would be seen in the post-starter period. Each of these starter-period treatments was divided into three groups in the post-starter period (10, 20, and 30% of the standard) with the aim of reducing the amount of dietary aP in response to probable induced adaptation in the starter period. The results showed that the birds in the post-starter period did not show remarkable response to the decrease of the starter phase aP diet. Although, their retarded growth due to starter aP restriction was well compensated and the Ca and P retention in response to a decrease in dietary P, were linearly increased at 42 days of age. Even the body weight of 42-day-old restricted chicks, except for the treatment with the most severe restriction (L3 L3), was numerically higher than the control treatment. In this experiment, aP and Ca restrictions were applied in the starter phase, but the recommended levels of these minerals were not used in the diet in the post-starter period. It is recommended that the control diet be used after the starter period restriction in future researches. If we consider the growth performance of broilers as a response criterion, it is possible to reduce dietary aP levels by 30% and 10% in the starter and post-starter periods, respectively.
On the other hand, the results of this experiment showed that, by reducing the level of aP in the diet, bone mineralisation is impaired. Even in treatments with a 10% reduction in dietary aP, the tibia ash, Ca, and P contents, as well as the femur’s breaking strength, were numerically decreased. Therefore, if we consider the tibia mineralisation as a response criterion, we should not reduce the dietary Ca and aP levels during the post-starter period. Since the bone mineralisation is a critical parameter in the fast-growing broilers, Ca and aP reduction in the diets will be risky. Therefore, we conclude that the recommended dietary levels of aP and Ca by Aviagen (Citation2014b) is appropriate and should not be reduced.
Conclusions
It could be concluded that dietary Ca and aP restriction up to 30% early in life (1–10 days of age) could make broilers utilise the two minerals more efficiently during the post-starter phase without negative effect on the birds’ growth performance. Dietary aP and Ca restriction impaired bone mineralisation and strength. The ATTR of Ca and P was improved by decreasing their dietary concentrations. Blood serum metabolites and carcase traits were not significantly affected by decreasing aP and Ca up to 30% in the diet.
Ethical approval
All procedures were approved by the Animal Care and Use Committee of the Ferdowsi University of Mashhad, Mashhad, Iran.
Disclosure statement
No potential conflict of interest was reported by the author(s).
Additional information
Funding
References
- Abdulla NR, Loh TC, Akit H, Sazili AQ, Foo HL, Kareem KY, Mohamad R, Abdul Rahim R. 2017. Effects of dietary oil sources, calcium and phosphorus levels on growth performance, carcass characteristics and bone quality of broiler chickens. J Appl Anim Res. 45:423–429.
- Abudabos AM. 2012. Intestinal phytase activity in chickens (Gallus domesticus). Afr J Microbiol Res. 6:4932–4938.
- Adedokun SA, Adeola O. 2013. Calcium and phosphorus digestibility: Metabolic limits. J Appl Poult Res. 22:600–608.
- Akter M, Graham H, Iji P. 2016. Response of broiler chickens to different levels of calcium, non-phytate phosphorus and phytase. Br Poult Sci. 57:799–809.
- Ashwell CM, Angel R. 2010. Nutritional genomics: a practical approach by early life conditioning with dietary phosphorus. R Bras Zootec. 39:268–278.
- Association of Official Analytical Chemists. 2005. Official Methods of Analysis of the AOAC. 18th ed. Gaithersburg (MD): Association of Official Analytical Chemists.
- Aviagen. 2014a. Ross broiler: management handbook. Huntsville (AL): Aviagen group.
- Aviagen. 2014b. Ross broiler: nutrition specifications. Huntsville (AL): Aviagen group.
- Bar A, Shinder D, Yosefi S, Vax E, Plavnik I. 2003. Metabolism and requirements for calcium and phosphorus in the fast-growing chicken as affected by age. Br J Nutr. 89:51–61.
- Cai Q, Chandler JS, Wasserman RH, Kumar R, Penniston JT. 1993. Vitamin D and adaptation to dietary calcium and phosphate deficiencies increase intestinal plasma membrane calcium pump gene expression. Proc Natl Acad Sci U S A. 90:1345–1349.
- Centeno VA, Díaz de Barboza GE, Marchionatti AM, Alisio AE, Dallorso ME, Nasif R, de Talamoni NGT. 2004. Dietary calcium deficiency increases Ca2+ uptake and Ca2+ extrusion mechanisms in chick enterocytes. Comp Biochem Physiol, Part A Mol Integr Physiol. 139:133–141.
- de Matos R. 2008. Calcium metabolism in birds. Vet Clin North Am Exot Anim Pract. 11:59–82.
- Delezie E, Bierman K, Nollet L, Maertens L. 2015. Impacts of calcium and phosphorus concentration, their ratio, and phytase supplementation level on growth performance, foot pad lesions, and hock burn of broiler chickens. J Appl Poult Res. 24:115–126.
- Dittmer KE, Thompson KG. 2011. Vitamin D metabolism and rickets in domestic animals: a review. Vet Pathol. 48:389–407.
- Farhadi D, Karimi A, Sadeghi G, Rostamzadeh J, Bedford MR. 2017. Effects of a high dose of microbial phytase and myo-inositol supplementation on growth performance, tibia mineralization, nutrient digestibility, litter moisture content, and foot problems in broiler chickens fed phosphorus-deficient diets. Poult Sci. 96:3664–3675.
- Gautier AE, Walk CL, Dilger RN. 2017. Influence of dietary calcium concentrations and the calcium-to-non-phytate phosphorus ratio on growth performance, bone characteristics, and digestibility in broilers. Poult Sci. 96:2795–2803.
- Hamdi M, López-Vergé S, Manzanilla EG, Barroeta AC, Pérez JF. 2015a. Effect of different levels of calcium and phosphorus and their interaction on the performance of young broilers. Poult Sci. 94:2144–2151.
- Hamdi M, Solà-Oriol D, Davin R, Perez JF. 2015b. Calcium sources and their interaction with the different levels of non-phytate phosphorus affect performance and bone mineralization in broiler chickens. Poult Sci. 94:2136–2143.
- Han J, Ma K, Wang J, Chen G, Zhang J, Qu H, Yan Y, Cheng Y. 2015. Effects of Non-phytate phosphorus and 1a-hydroxycholecalciferol on growth performance, bone mineralization, and carcass traits of broiler chickens. Rev Bras Cienc Avic. 17:503–510.
- Kheiri F, Rahmani H. 2006. The effect of reducing calcium and phosphorous on broiler performance. International J of Poultry Science. 5:22–25.
- Kitazawa S, Kajimoto K, Kondo T, Kitazawa R. 2003. Vitamin D3 supports osteoclastogenesis via functional vitamin D response element of human RANKL gene promoter. J Cell Biochem. 89:771–777.
- Leske K, Coon C. 2002. The development of feedstuff retainable phosphorus values for broilers. Poult Sci. 81:1681–1693.
- Mirakzehi MT, Kermanshahi H, Golian A, Raji AR. 2013. The effects of dietary 1, 25-dihydroxycholecalciferol and hydroalcoholic extract of Withania somnifera root on bone mineralisation, strength and histological characteristics in broiler chickens. Br Poult Sci. 54:789–800.
- Mitchell RD, Edwards HM Jr. 1996. Additive effects of 1,25-dihydroxycholecalciferol and phytase on phytate phosphorus utilization and related parameters in broiler chickens. Poult Sci. 75:111–119.
- Mondal MK, Panda S, Biswas P. 2007. Effect of microbial phytase in soybean meal based broiler diets containing low phosphorous. Int J Poult Sci. 6:201–206.
- Mutucumarana RK, Ravindran V, Ravindran G, Cowieson AJ. 2014. Influence of dietary calcium concentration on the digestion of nutrients along the intestinal tract of broiler chickens. Jpn Poult Sci. 51:392–401.
- Onyango EM, Hester PY, Stroshine R, Adeola O. 2003. Bone densitometry as an indicator of percentage tibia ash in broiler chicks fed varying dietary calcium and phosphorus levels. Poult Sci. 82:1787–1791.
- Perryman KR, Masey O'Neill HV, Bedford MR, Dozier WA. 2016. Effects of calcium feeding strategy on true ileal phosphorus digestibility and true phosphorus retention determined with growing broilers. Poult Sci. 95:1077–1087.
- Plumstead PW, Leytem AB, Maguire RO, Spears JW, Kwanyuen P, Brake J. 2008. Interaction of calcium and phytate in broiler diets. 1. Effects on apparent prececal digestibility and retention of phosphorus. Poult Sci. 87:449–458.
- Proszkowiec-Weglarz M, Angel R. 2013. Calcium and phosphorus metabolism in broilers: effect of homeostatic mechanism on calcium and phosphorus digestibility. J Appl Poult Res. 22:609–627.
- Rousseau X, Valable AS, Létourneau-Montminy MP, Même N, Godet E, Magnin M, Nys Y, Duclos MJ, Narcy A. 2016. Adaptive response of broilers to dietary phosphorus and calcium restrictions. Poult Sci. 95:2849–2860.
- SAS (Statistical Analyses System). 2012. SAS/STAT Software, Version 9.4. Cary (NC): SAS Institute Inc.
- Selle PH, Cowieson AJ, Ravindran V. 2009. Consequences of calcium interactions with phytate and phytase for poultry and pigs. Livest Sci. 124:126–141.
- Tizziani T, de Oliveira Donzele RFM, Donzele JL, Silva AD, Muniz JCL, de Freitas Jacob R, Brumano G, Albino LFT. 2019. Reduction of calcium levels in rations supplemented with vitamin D3 or 25-OH-D3 for broilers. R Bras Zootec. 48:1–14.
- Underwood EJ, Suttle NF. 1999. The mineral nutrition of livestock. 3rd ed. Wallingford (UK): CABI Publishing.
- Wilkinson SJ, Bradbury EJ, Bedford MR, Cowieson AJ. 2014. Effect of dietary nonphytate phosphorus and calcium concentration on calcium appetite of broiler chicks. Poult Sci. 93:1695–1703.
- Yan F, Angel R, Ashwell C, Mitchell A, Christman M. 2005. Evaluation of the broiler’s ability to adapt to an early moderate deficiency of phosphorus and calcium. Poult Sci. 84:1232–1241.