Abstract
The aim of the present study was to evaluate the effects of dietary total sulphur amino acids (TSAA) levels and guanidinoacetic acid (GAA) supplementation on performance, carcase yield and energetic molecular metabolites in heart and breast muscle of broiler chickens fed wheat-soy diet. A total of 450, male one-day-old Ross-308 broiler chicks were assigned in a 3 × 3 factorial arrangement of nine treatments, five replicates and 10 birds each. Dietary treatments included three graded levels of supplementary TSAA (+0.4 g/kg per level) resulting in a deficient, adequate and plus level of recommended by strain, with three levels of GAA (0, 0.6 and 1.2 g/kg) and were fed for 35 days. Dietary TSAA levels only enhanced performance during the starter period (p < .05). At adequate TSAA level, weight gain (WG) and feed conversion ratio (FCR) in the grower phase were positively affected by supplementing GAA at 1.2 g/kg (p < .05). The FCR during the overall experimental period was significantly lower when feeding GAA at 0.6 and 1.2 g/kg compared to non-supplemented (p < .05). Heart and breast muscle ATP/AMP ratio and breast muscle ADP and PCr concentration and PCr/CrN ratio in the birds fed 1.2 g/kg GAA diet were significantly higher than non-supplemented (p < .05). It is concluded, effects of dietary GAA supplementation were influenced by dietary TSAA level, this indicates to need for proper dietary sulphur amino acid formulation by GAA supplementation. Dietary GAA supplementation could improve broiler chicken’s growth performance via regulating some aspects of energy metabolism.
Guanidinoacetic acid plays a critical role in energy homeostasis.
The effect of dietary Guanidinoacetic acid supplementation may influenced by the dietary sulphur amino acids.
HIGHLIGHTS
Introduction
Guanidinoacetic acid (GAA) is a natural precursor for creatine (Cr) which plays a critical role in cell energy homeostasis (Tabatabaei Yazdi et al. Citation2017). In muscle cell Cr is involved in energy metabolism through the creatine-phosphocreatine shuttle, which is that of a cellular energy transport system (Cr-PCr shuttle). Phosphocreatine (PCr) is a phosphorylated Cr molecule that serves as a rapidly mobilisable reserve of high-energy phosphates in skeletal muscle and myocardium to recycle adenosine triphosphate when required on short notice (Wyss and Kaddurah-Daouk Citation2000). In fast-growing animals such as broiler chickens, the requirement for Cr is proportionally greater because of their high need to supply Cr for muscles in the generation ATP from PCr and to replace Cr losses in the creatinine (CrN) form (Brosnan et al. Citation2009). Supplementation of broiler chickens diets with GAA (as Cr precursor) might restore the Cr load in tissues (Nain et al. Citation2008; Ringel et al. Citation2008; Michiels et al. Citation2012).
The beneficial effects of supplemental GAA to poultry diets have been demonstrated in many experiments (Abudabos et al. Citation2014; Tabatabaei Yazdi et al. Citation2017; DeGroot et al. Citation2018; Majdeddin et al. Citation2019; Michiels et al. Citation2012). It has been assumed that performance enhancements derive from various benefits, including the restoration of Cr levels in tissues (Michiels et al. Citation2012), and arginine sparing effect (Dilger et al. Citation2013; DeGroot et al. Citation2018), stimulation of myogenic differentiation and muscle growth (Wang et al. Citation2018), stimulation of hormonal release and neuromodulation and an adjustment of oxidant-antioxidant status (Ostojic et al. Citation2016). Since GAA is endogenously converted to Cr by methylation, this requires the presence of a methyl group donor, it was hypothesised that the effects of dietary GAA supplementation might determine the methionine provision in vegetable-based diets (Majdeddin et al. Citation2018). On other fracases, methionine deficiency may limit the availability of methyl groups for GAA methylation (Lemme, Gobbi, and Esteve-Garcia Citation2010). The objective of the current study was to evaluate the effects dietary total sulphur amino acids (TSAA) levels and GAA supplementation on growth performance, carcase yield and energetic metabolites in heart and breast muscle of broiler chickens fed wheat-soy diet.
Material and methods
Birds, housing and care
A total of 450, male one-day-old broiler Ross 308 chicks were obtained from a commercial hatchery and randomly assigned to 45 pens of 10 birds each. Each pen was one square metre space and, floors were covered with wood shaving. The house temperature during initially three days was maintained at 32–34 °C and then was gradually decreased 0.5 °C per day to reach a constant temperature of 20–22 °C on d 24 and was kept constant thereafter. The relative humidity was 50–60% and the lighting programme was 23 L:1 D during the whole experimental period. Animals and housing facilities were inspected three times daily.
Experimental diets
Prior to the trial, samples of the main ingredients (soybean meal: DM, 893; CP, 455; EE, 15; and ash, 75 g/kg; and wheat: DM, 889; CP, 110; EE, 35; and ash, 20 g/kg) were analysed for proximate composition. The amino acid compositions of wheat and soy meal were determined by NIR. These values were used in a least-cost formulation for the experimental diets. Diets were formulated, based on the nutrient requirements of Ross-308 rearing guideline for target live weight 1.7–2.5 kg (Aviagen Citation2018). A completely randomised design experiment with 9 treatments and 5 replicates per treatment was run as a 3 × 3 factorial arrangement comprised of three levels of supplemental Met (MetAMINO, >99%, Evonik Degussa GmbH, Hanau-Wolfgang, Germany) and three levels of supplemental GAA (CreAMINO, >96% GAA, Evonik Degussa GmbH, Hanau-Wolfgang, Germany). As cystine was equal across groups, the three levels of supplemental Met correspond to three levels of TSAA, that is, deficient, 0.4 g/kg less than a requirement; adequate, equal to requirement; and in plus, 0.4 g/kg more than the requirement of recommended by Ross strain (Aviagen Citation2018). The three supplementary levels of GAA were 0, 0.6 and 1.2 g/kg, corresponding to levels most often used in broiler studies and allowed by most feed ingredient legislation across the globe (Majdeddin et al. Citation2019). Ingredient and diet compositions are presented in Table . All birds had free access to mash feed and water throughout the whole experimental period.
Table 1. Feed ingredients and nutrient composition of experimental diets.Table Footnotea.0
Performance traits
Live body weight (LBW) at 1, 10, 24 and 35 d of ages by weighing pen live birds and feed intake during starter (d1–10), grower (d11–24), and finisher (d25–35) periods by weighing the offered and residual feed at the beginning and end of each period for every replicate pen were recorded. The growth performance as mean LBW, weight gain (WG) and feed (FI) intake were calculated during starter, grower, finisher and overall (d1–35) periods. Daily chick mortality was weighed, recorded and used to correct the growth performance. The FCR (adjusted for mortality and calculated as total feed intake divided by total gain including the weight of lost birds) and viability during the overall period (d1–35) were calculated as 100-mortality (%). Similarly, the European Production Efficiency Factor (EPEF) was calculated as: [viability d1–35 (%) × LBW at d35 (kg) × 100]/[age (35) × FCR d1–35].
Slaughter and sampling procedures
At the end of the experimental period, after 6 h of removal of feeders, one chicken/pen (five/treatment), close to the average pen weight was selected. Birds were gently handled in a calm manner prior to decapitation. After bleeding, the carcase was obtained by removing, feathers, feet, skin and visceral organs. Weight of the carcase and part (breast, legs and frame defined carcase without the breast and legs) yield and abdominal fat were expressed as a percentage of live body weight (Zarghi et al. Citation2020). In order to measure molecular concentrations of energetic metabolites in heart and breast muscles, about 1 g of heart and breast (1.5 cm deep in the right pectorals tissue) muscle were collected and wrapped in an aluminium foil and kept in −80 °C freezer for later (determined ATP, ADP, AMP, PCr and CrN concentration) analysis (Tabatabaei Yazdi et al. Citation2017).
Measurement of ATP, ADP, AMP, PCr and CrN in muscles
The concentrations of adenosine phosphate (ATP, ADP, and AMP) and PCr and CrN in heart and breast muscles were evaluated by reverse-phase-HPLC according to methods published by Liu et al., (Citation2006) and Tabatabaei Yazdi et al. Citation(2017). In brief, a frozen muscle sample (200 mg) was cut and homogenised for 1 min in 1.0 mL of ice-cold 0.4 M HClO4 and kept in an ice bath for 10 min, then homogenate was centrifuged for 10 min at 3600 g, in the refrigerated centrifuge (4 °C). The supernatant (850 μL) was collected and adjusted to a pH of 6.5 with 850 μL of 0.6 M K2HPO4, and the mixture was mixed (by vortex) for 20 s and centrifugation was repeated for 5 min. The supernatant (1.5 mL) was collected and stored at −20 °C and analysed within 24 h. The separation of muscle ATP, ADP, AMP, PCr and CrN were performed using an Alliance HPLC system Waters-2695 (Waters Corporation, Milford, MA), equipped with an integrated auto-sampler. Chromatographs were recorded with a Waters-2996 PDA UV detector. The HPLC conditions were set as follows: (a) Purospher STAR RP-18e column (250 × 4.6 mm i.d., 5 μm) with a column temperature of 30 °C, (b) Mobile phase, acetonitrile: 50 mM KH2PO4 buffer solution (vol/vol = 10:90, pH adjusted to 6.5 for ATP, ADP, AMP and 6.8 for PCr and CrN using 1 M KOH) at a flow rate of 0.8 mL/min, (c) detection wavelength was 254 nm; and the injection volume was 20 μL, (d) sample and mobile phase water was prepared using a Milli-Q water purification system (Millipore Corp., Bedford, MA). Both of sample and mobile phase solution were filtered with 0.45-μm Durapore membrane polyvinylidene fluoride filters (Millipore Corp), (e) Standard stock solutions (1000 μg/mL) were diluted to 5, 10, 20, 40, 80, 160, and 320 μg/mL in deionised water, (f) a volume of 20 μL of each standard sample was used for HPLC analysis, (g) the standard curve and regression equation was established between the chromatographic peak area (y-axis, mV min) and standard sample concentration (x-axis, μg/mL), and (h) the retention times for ATP, ADP, AMP, PCr and CrN were 3.4, 3.7, 4.4, 3.5 and 4.2 min, respectively (Liu et al. Citation2006; Tabatabaei Yazdi et al. Citation2017). All standards were purchased from Sigma-Aldrich Inc. The ATP, ADP and AMP standard stock solutions of 1000 μg/mL were prepared by dissolving 50 mg of ATP, ADP and AMP standards in 50 mL of per-chloric acid solution (0.4 M HClO4) and stored at 4 °C.
Statistical analysis
The data were tested for main effects of diet TSAA levels and GAA supplementation and for their interaction. Analysis of variance was performed using a completely randomised design experiment with a factorial arrangement. All percentage data were transformed to arc-sin before statistical analysis (Kaps and Lamberson Citation2017). All data were analysed by ANOVA using the GLM procedure of SAS 9.1 software (SAS Citation2003). Means were compared for significant differences using Tukey–Kramer’s multiple range test (p < .05).
Results
Performance traits
Main and interactive effect of dietary TSAA levels and GAA supplementation on average live body weight (LBW), average daily weight gain (WG), average daily feed intake (FI), feed conversion ratio (FCR), viability and European production efficiency factor (EPEF) in male broiler chickens during starter (d1-10), grower (d11–24), finisher (d25–35) and whole (d1–35) experimental periods are shown in Table . Increasing levels of dietary TSAA significantly improved growth performance in the starter period (p < .05). Birds fed the TSAA deficient diet performed less than birds fed diets with TSAA at adequate and plus levels, so that difference between TSAA deficient and TSAA plus levels was significant (p < .05). The GAA main effect and TSAA × GAA interaction effects were significant for WG (p < .05) and FCR (p < .01) in the grower period. Dietary supplementation by 0.6 g/kg GAA significantly increased WG and decreased FCR compared non-supplemented diet (p < .05). Dietary GAA supplementation main and TSAA × GAA interaction effects were not significant on performance during the starter and finisher periods. The FCR during the overall experimental period was significantly lower when feeding GAA at 0.6 and 1.2 g/kg compared to non-supplemented diet (p < .05). Additionally, the EPEF value was significantly higher and FCR was significantly lower in the birds fed the TSAA plus diet as compared to adequate and deficient TSAA diet (p < .05).
Table 2. Effect of dietary total sulphur amino acid (TSAA) levels and guanidinoacetic acid (GAA) supplementation on live body weight (BW), average daily weight gain (WG), average daily feed intake (FI), feed conversion ratio (FCR), viability and European production efficiency factor (EPEF) in male broiler chickens fed wheat-soy diet.a
Carcase traits and organ weights
The main and interactive effect of dietary TSAA levels and GAA supplementation on carcase traits of birds sampled on d 35 are given in Table . Relative breast weight and legs (thigh + drumstick) weights significantly increased with increasing dietary TSAA levels (p < .05). In contrast, abdominal fat weight was reduced (p < .01). Dietary supplementation by GAA did not affect any of the carcase traits at d 35.
Table 3. Effect of dietary total sulphur amino acid (TSAA) levels and guanidinoacetic acid (GAA) supplementation on carcase traits in male broiler chickens on day 35.Table Footnotea
Energetic molecular metabolites
Main and interactive effect of dietary TSAA levels and GAA supplementation on energetic molecular metabolites in heart and breast muscle sampled at d 35 are given in Table . There was not effect of dietary TSAA levels on any of the heart and breast muscle energy metabolites (ATP, ADP, AMP, PCr and CrN concentration and ATP/AMP, PCr/CrN and PCr/ATP ratio), with the exception of an effect on breast muscle ADP concentration and PCr/CrN ratio at d 35 (p < 0.05). The dietary GAA supplementation main effects on all of the heart muscle energetic molecular metabolites were not significant, with the exception of an effect on ATP/AMP ration (p < .05). By supplementing the diet with 0.6 and 1.2 g/kg GAA, heart muscle ATP/AMP ratio significantly enhanced. The breast muscle ADP and PCr concentration and ATP/AMP and PCr/CrN ratio were significantly affected by dietary GAA supplementation (p < .05). By supplementing the diet with 1.2 g/kg GAA, breast muscle ADP and PCr concentration and ATP/AMP and PCr/CrN ratio significantly enhanced. No treatment interactions were observed for any energetic metabolites.
Table 4. Effect of dietary total sulphur amino acid (TSAA) level and guanidine acetic acid (GAA) supplementation on energy metabolites in broiler chickens heart and breast fresh muscle at day 35.Table Footnotea
Discussion
Performance traits
Increasing dietary TSAA levels did not affect FI. A deficient supply of some AA can result in a reduction in appetite Conde-Aguilera et al. Citation2013, and a deficient Met supply has been reported to reduce feed intake (Bunchasak Citation2009). The main reason to equalise feed intake between treatments in this study was a possible interfering effect of the high level of wheat on FI. In the endosperm cell wall of wheat, there are structural carbohydrates (arabinoxylan), the anti-nutritional effects of arabinoxylan related to its polymeric nature and adhesion properties. Due to this property, the contents of the intestine become a special soluble, preventing the free movement of contains. In our experimental conditions, increasing dietary TSAA levels improved WG and FCR during the starter period and overall FCR. But, the dietary TSAA levels did not affect performance indices during the grower and finisher periods. The sufficient level of TSAA used in this study was calculated to meet the recommended requirement of strain (Aviagen Citation2018). The deficient level corresponded to approximately 95% of the supply of diet TSAA, but this level was only marginally deficient level. This may explain that the performance response to the Met deficiency was moderate during the starter and did not affect during the grower and finisher periods.
Our results showed an improvement in overall FCR of birds when GAA was added to the diets (Table ). Similarly, several other studies have shown that dietary GAA supplementation improved FCR in broiler chickens (Ringel et al. Citation2007; Michiels et al. Citation2012; Dilger et al. Citation2013; Mousavi et al. Citation2013). A significant improvement in FCR of broiler chickens by the addition of 0.6 and 1.2 g GAA/kg diet was approved (Lemme et al. Citation2007; Ringel et al. Citation2008). Supplementation of GAA in all-vegetable diets improves performance (Michiels et al. Citation2012). Other studies indicated that GAA supplementation of up to 0.12% to all-vegetable diets improved performance in male and female broiler chickens (Lemme et al. Citation2007). Furthermore, supplementation of GAA to turkey’s diets also improved growth performance (Lemme, Gobbi, Helmbrecht, et al. Citation2010). This positive effect of GAA may be associated with its ability to increase the cells’ Cr level. Because its deficiency may act as a limiting factor for growth, especially in birds that fed solely with plant ingredient because plant feedstuffs lacking in Cr (Michiels et al. Citation2012). In contrast, Some researchers did not find a significant effect of diet GAA supplementation on FCR of broiler chickens (Mousavi et al. Citation2013; Majdeddin et al. Citation2018).
In our experimental condition, interaction effects between dietary TSAA levels and GAA supplementation were significant for WG and FCR in the grower period. Dietary supplementation by 0.6 g/kg GAA significantly increased WG and decreased FCR compared non-supplemented diet. It was clear that dietary supplementation with 1.2 g/kg GAA had hardly lower WG in deficient and slightly lower WG in plus diets compared to the adequate and the dietary supplementation with 0.6 g/kg GAA had higher WG in deficient diet, whereas in adequate diet WG increased by increasing dietary GAA levels (Figure ). Feed conversion ratio significantly decreased by increasing 0.6 and 1.2 g/kg GAA for the adequate group, but in deficient diet, FCR increased by increasing 1.2 g/kg GAA (Figure ). This positive effect of GAA in the birds fed a diet with sufficient and plus TSAA might be related to its ability to increase muscle Cr levels (Wyss and Kaddurah-Daouk Citation2000). Overall, the effects of dietary GAA supplementation were influenced by the dietary TSAA level indicates the need for proper sulphur amino acid formulation in diets when feeding GAA.
Figure 1. Effect of dietary total sulphur amino acid (TSAA) levels and guanidinoacetic acid (GAA) supplementation on average daily gain (ADG) of male broiler chickens for period’s d11–24. Bars with different superscripts are significantly different at p < .05. Deficient, 0.4 g/kg less than requirement for TSAA; Adequate, equal to requirement for TSAA; and Plus, 0.4 g/kg more than requirement for TSAA. GAA supplementary levels were 0, 0.6 and 1.2 g/kg.
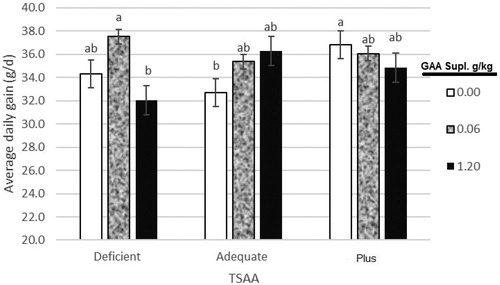
Figure 2. Effect of dietary total sulphur amino acid (TSAA) levels and guanidinoacetic acid (GAA) supplementation on feed conversion ratio (FCR) of male broiler chickens for period’s d11–24. Bars with different superscripts are significantly different at p < .05. Deficient, 0.4 g/kg less than requirement for TSAA; Adequate, equal to requirement for TSAA; and Plus, 0.4 g/kg more than requirement for TSAA. GAA supplementary levels were 0, 0.6 and 1.2 g/kg.
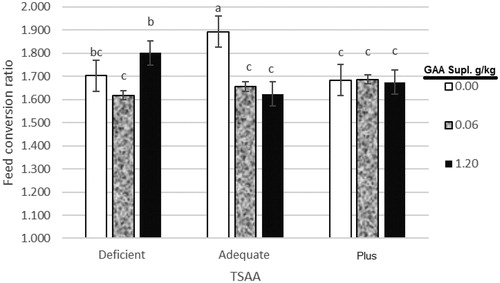
Carcase traits
Increasing dietary TSAA levels improved relative breast and legs (thigh + drumstick) weight and decreased abdominal fat deposition, in agreement with our result in this experiment, other researcher reported in broiler chickens muscle growth significantly will improvement in by supplementation SAA (Conde-Aguilera et al. Citation2013; Drazbo et al. Citation2015; Wen et al. Citation2017; Majdeddin et al. Citation2019). The sulphur amino acids (Met + Cys) are typically the first limiting AA for protein deposition in poultry diets. Met is an important methyl donor and precursor for the biosynthesis of compounds such as Cr, CrN, and polyamines (Baker et al. Citation1996; Conde-Aguilera et al. Citation2013). It is well known that dietary Met supplementation promotes growth by increasing protein synthesis (Majdeddin et al. Citation2019). Indeed, the addition of TSAA has been correlated with the tendency to less total body fat (Rostagno et al. Citation1995). In our experimental conditions, the dietary GAA supplementation not effect on carcase traits, in accordance with our result some researcher did not find a significant effect of diet GAA supplementation on carcase and part yield of broiler chickens (Mousavi et al. Citation2013; Majdeddin et al. Citation2018). However, some experiments reported supplementation of GAA in all-vegetable diets improves carcase characteristics in terms of breast meat yield (Michiels et al. Citation2012).
Energetic molecular metabolites
In agreement with our result in this experiment, many studies have confirmed the Cr loading effect of GAA (Lemme et al. Citation2007; Ringel et al. Citation2007; Ringel et al. Citation2008; Michiels et al. Citation2012; Tabatabaei Yazdi et al. Citation2017; Majdeddin et al. Citation2018; Majdeddin et al. Citation2019). Addition GAA to fast-growing broiler chickens diet can be useful to meet the needs of chicks to Cr (Brosnan et al. Citation2009). Ringel et al. (Citation2007) reported 66 to 75% of Cr requirements are met endogenously, and the remainder must be supplied by the diet. It is well known, GAA is the single immediate precursor of Cr in animal tissue (Michiels et al. Citation2012; Tabatabaei Yazdi et al. Citation2017). Cr act as a key energy reserve in muscle cells devoid from ATP, it helps to avoid the depletion of cellular ATP through the immediate provision of high energy phosphates to generate the ATP (Wyss and Kaddurah-Daouk Citation2000). Our results in this experiment showed by dietary GAA supplementation broiler chickens heart muscle ATP/AMP ratio, breast muscle ATP/AMP ratio and breast muscle ADP concentration increased 60, 56 and 14%, respectively than chickens fed non-supplemented diet. Also, breast muscle PCr concentration and PCr/CrN ratio increased, via the addition of GAA to diet. The increases in breast muscle ADP and PCr concentration, and ATP/AMP and PCr/CrN ratio may indicate that oxidative phosphorylation are elevated after GAA supplementation. Our result in this study indicates that PCr increased buffering capacity for ATP hydrolysis and supports the idea that Cr-loaded muscles have the capacity for increased muscle work, which might be beneficial for skeletal muscle growth. This indicated that GAA can serve as an efficient Cr source that has the potential to rapidly mobilise reserve of high-energy phosphate for ATP formation. It may be speculated that the cellular energy requirement is augmented during GAA supplementation due to an anabolic effect of Cr (Kreider Citation1999).
Conclusions
Under the conditions of fed broiler chickens with wheat-soy diet, the effect of dietary GAA supplementation on performance traits was influenced by the dietary TSAA level. The primary finding of this study was that GAA is an efficient precursor to in terms of Cr loading to improve energy homeostasis in heart and breast muscle cells. Overall, in the broiler chickens fed wheat-soy based diet positive outcomes of supplementing GAA might depend on the proper formulation of other factors in the diet, not least to sulphur amino acid provision.
Ethical Approval
The experiment was conducted with the approval of theAnimal Care Committee of the Ferdowsi University ofMashhad (Mashhad, Iran).
Acknowledgement
The authors would like to thank the Evonik Co. in Iran for supplying CreAMINO and feedstuffs amino acid analysis. This work was supported by the vice president in research at the Ferdowsi University of Mashhad, Iran (Research Code: 3/30431).
Disclosure statement
No potential conflict of interest was reported by the author(s).
References
- Abudabos AM, Saleh F, Lemme A, Zakaria HAH. 2014. The relationship between guanidino acetic acid and metabolisable energy level of diets on performance of broiler chickens. Ital J Anim Sci. 13(3):548–556.
- Aviagen. 2018. Ross 308: broiler nutrition specification. Huntsville (AL): Aviagen.
- Baker DH, Fernandez SR, Webel DM, Parsons CM. 1996. Sulfur amino acid requirement and cystine replacement value of broiler chicks during the period three to six weeks posthatching. Poult Sci. 75(6):737–742.
- Brosnan JT, Wijekoon EP, Warford-Woolgar L, Trottier NL, Brosnan ME, Brunton JA, Bertolo RF. 2009. Creatine synthesis is a major metabolic process in neonatal piglets and has important implications for amino acid metabolism and methyl balance. J Nutr. 139(7):1292–1297.
- Bunchasak C. 2009. Role of dietary methionine in poultry production. Jpn Poult Sci. 46(3):169–179.
- Conde-Aguilera JA, Cobo-Ortega C, Tesseraud S, Lessire M, Mercier Y, Van Milgen J. 2013. Changes in body composition in broilers by a sulfur amino acid deficiency during growth. Poult Sci. 92(5):1266–1275.
- DeGroot AA, Braun U, Dilger RN. 2018. Efficacy of guanidinoacetic acid on growth and muscle energy metabolism in broiler chicks receiving arginine-deficient diets. Poult Sci. 97(3):890–900.
- Dilger RN, Bryant-Angeloni K, Payne RL, Lemme A, Parsons CM. 2013. Dietary guanidino acetic acid is an efficacious replacement for arginine for young chicks. Poult Sci. 92(1):171–177.
- Drazbo A, Kozlowski K, Chwastowska-Siwiecka I, Sobczak A, Kwiatkowski P, Lemme A. 2015. Effect of different dietary levels of DL-methionine and the calcium salt of DL-2-hydroxy-4-[methyl] butanoic acid on the growth performance, carcass yield and meat quality of broiler chickens. Eur Poultry Sci. 79(7):1–14.
- Kaps M, Lamberson WR. 2017. Biostatistics for animal science. Wallingford (UK): CABI.
- Kreider RB. 1999. Dietary supplements and the promotion of muscle growth with resistance exercise. Sports Med. 27(2):97–110.
- Lemme A, Gobbi R, Esteve-Garcia E. 2010. Effectiveness of creatine sources on performance of broilers at deficient or adequate methionine supply. In Proceedings of the XIII European Poultry Conference, 23-27 August 2010, Tours, France, poster113.
- Lemme A, Gobbi R, Helmbrecht A, Van Der Klis JD, Firman J, Jankowski J, Kozlowsk K. 2010. Use of guanidine acetic acid in all-vegetable diets for turkeys. In Proceedings of the 4th Turkey Science Production Conference, Macclesfield, UK. Tarporley, Cheshire, UK: Turkeytimes, pp. 57–61.
- Lemme A, Ringel J, Rostagno HS, Redshaw MS. 2007. Supplemental guanidine acetic acid improved feed conversion, weight gain, and breast meat yield in male and female broilers. Paper presented at the 16th European Symposium on Poultry Nutrition; August 26–30; Strasbourg, France.
- Liu H, Jiang YM, Luo YB, Jiang WB. 2006. A simple and rapid determination of ATP, ADP and AMP concentrations in pericarp tissue of litchi fruit by high performance liquid chromatography. Food Technol Biotechnol. 44:531–534.
- Majdeddin M, Golian A, Kermanshahi H, De Smet S, Michiels J. 2018. Guanidinoacetic acid supplementation in broiler chickens fed on corn-soybean diets affects performance in the finisher period and energy metabolites in breast muscle independent of diet nutrient density. Br Poult Sci. 59(4):443–451.
- Majdeddin M, Golian A, Kermanshahi H, Michiels J, De Smet S. 2019. Effects of methionine and guanidinoacetic acid supplementation on performance and energy metabolites in breast muscle of male broiler chickens fed corn-soybean diets. Br Poult Sci. 60(5):554–563.
- Michiels J, Maertens L, Buyse J, Lemme A, Rademacher M, Dierick NA, De Smet S. 2012. Supplementation of guanidinoacetic acid to broiler diets: effects on performance, carcass characteristics, meat quality, and energy metabolism. Poult Sci. 91(2):402–412.
- Mousavi SN, Afsar A, Lotfollahian H. 2013. Effects of guanidinoacetic acid supplementation to broiler diets with varying energy contents. J Appl Poult Res. 22(1):47–54.
- Nain S, Ling B, Alcorn J, Wojnarowicz CM, Laarveld B, Olkowski AA. 2008. Biochemical factors limiting myocardial energy in a chicken genotype selected for rapid growth. Comp Biochem Physiol Part A, Mol Integrative Physiol. 149(1):36–43.
- Ostojic SM, Drid P, Ostojic J. 2016. Guanidinoacetic acid increases skeletal muscle creatine stores in healthy men. Nutrition. 32(6):723–724.
- Ringel J, Lemme A, Knox A, McNab J, Redshaw MS. 2007. Effects of graded levels of creatine and guanidino acetic acid in vegetable-based diets on performance and biochemical parameters in muscle tissue. Paper presented at the 16th European Symposium on Poultry Nutrition; August 26–30; Strasbourg, France.
- Ringel J, Lemme A, Redshaw MS, Damme K. 2008. The effects of supplemental guanidino acetic acid as a precursor of creatine in vegetable broiler diets on performance and carcass parameters. Poult Sci. 87(1):72.
- Rostagno HS, Pupa JMR, Pack M. 1995. Diet formulation for broilers based on total versus digestible amino acids. J Appl Poult Res. 4(3):293–299.
- SAS. 2003. User’s guide: statistics, version 9.1. Vol. 2. Cary (NC): S.A.S Institute.
- Tabatabaei Yazdi F, Golian A, Zarghi H, Varidi M. 2017. Effect of wheat-soy diet nutrient density and guanidine acetic acid supplementation on performance and energy metabolism in broiler chickens. Ital J Anim Sci. 16(4):593–600.
- Wang Y, Ma J, Qiu W, Zhang J, Feng S, Zhou X, Wang X, Jin L, Long K, Liu L, et al. 2018. Guanidinoacetic acid regulates myogenic differentiation and muscle growth through miR-133a-3p and miR-1a-3p co-mediated Akt/mTOR/S6K signaling pathway. IJMS. 19(9):2837–2856.
- Wen C, Jiang X, Ding L, Wang T, Zhou Y. 2017. Effects of dietary methionine on breast muscle growth, myogenic gene expression and IGF-I signaling in fast- and slow-growing broilers . Sci Rep. 7(1):1924–1931.
- Wyss M, Kaddurah-Daouk R. 2000. Creatine and creatinine metabolism. Physiol Rev. 80(3):1107–1213.
- Zarghi H, Golian A, Nikbakhtzade M. 2020. Effect of dietary digestible lysine level on growth performance, blood metabolites and meat quality of broilers 23–38 days of age. J Anim Physiol Anim Nutr. 104(1):156–165.