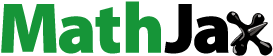
Abstract
The present study aimed to investigate non-genetic sources of variation of milk production and quality traits of dual-purpose Original Brown (OB) cows farmed in Italian alpine region by using milk test-day records (n = 4034). Except for milk yield and somatic cell count, all phenotypes were predicted from milk mid-infrared spectra and included traditional composition traits, coagulation properties, casein fractions, minerals and fatty acids. Data were analysed using a mixed linear model which included calving season, parity, stage of lactation and first-order interactions as fixed effects, and cow and herd-test-date as random effects. Milk yield averaged 18.07 kg/d and means of fat, protein and casein content (%) were 4.03, 3.40 and 2.68, respectively. Primiparous OB cows produced milk with a greater content of unsaturated fatty acids compared with multiparous. Except for Na, minerals content was generally greater in primiparous than multiparous cows. The comparison with the literature demonstrated that OB cows produce milk with fatty acid profile similar to that of Alpine Grey and with more favourable coagulation properties and mineral composition than Pinzgauer cows. Considering that OB milk exhibited good coagulation properties, a prerequisite for cheesemaking, strategies to valorise this endangered breed should mainly focus on the manufacturing of OB mountain cheeses. In general, results for several milk-related performance coupled with the well-known strong adaptability of the breed to extensive mountain farming systems are the starting point towards the valorisation and potential expansion of this local dual-purpose genetic resource, with the ultimate goal of contributing to its conservation.
Primiparous cows showed greater mineral content compared to multiparous cows.
The good milk coagulation properties and high α-CN and κ-CN of Original Brown cows milk are a prerequisite for cheesemaking efficiency.
A greater content of unsaturated fatty acids was observed in primiparous than multiparous OB cows.
Highlights
Introduction
Original Brown (OB) is an autochthonous cattle breed originating from Switzerland and mainly farmed in the alpine regions of Austria, Germany, Italy and Switzerland (Maxa et al. Citation2012). The OB is a middle framed dual-purpose breed of strong bone structure and good muscularity, producing on average 5000 kg of milk per lactation with fat and protein content of 4.00% and 3.30%, respectively, and with carcass characteristics superior to Brown Swiss (BS; Südtiroler Braunviehzuchtverband Citation2020). Due to its phenotypic characteristics, such as robust feet and legs and feed efficiency, OB is highly suitable for alpine low input (dairy and beef) production systems and therefore might be recommended for mountain farming (Braunvieh Schweiz Citation2020).
At the end of the nineteenth century OB cows were exported from Switzerland to the US and underwent several selection programs aimed at improving the dairy attitude, particularly milk yield (MY). This led to the birth of the specialised BS dairy breed (Yoder and Lush Citation1937) that was subsequently used in the 1960s through upgrading programs to improve MY in European BS populations (Maxa et al. Citation2012). As a result, OB has been mainly replaced by high yielding BS genetics, becoming therefore an endangered breed (GEH Citation2020). In South Tyrol (Italian alpine region), OB has a long breeding history and it has been the first cattle breed organised in a breeders’ association. Currently there are financial supports for farmers from EU (200 € per lactating cow; Südtiroler Bauernbund Citation2020) and some valorisation strategies in South Tyrol to support the OB cattle breed.
Further, being an autochthonous cattle breed of alpine area, OB might incorporate potential for diversification when considered for social and economic purposes like marketing and branding of unique agricultural products from specific areas (Marsoner et al. Citation2018). In 2019, 241 lactating cows were farmed in South Tyrol averaging 5539 kg of MY, 3.93% of fat and 3.40% of protein per lactation (Südtiroler Braunviehzuchtverband Citation2020). To our knowledge, a comprehensive characterisation of OB breed population for milk traits and their variation has not been reported yet in the literature. Thus, the aim of the present study was to describe and investigate sources of variation of quality traits of interest in milk of OB cows; in particular, the effects of season of calving, parity, and stage of lactation were studied.
Material and methods
Area of the study
Approximately 40% of all Italian OB cows are reared in the province of Bolzano (South Tyrol, Italy) and the remaining ones can be found in the regions of Piedmont (40%) and Lombardy (20%; Rossoni A., personal communication). The geographical distribution of the 23 farms involved in this study is depicted in Figure . Being an autochthonous dual-purpose breed, OB cows are typically farmed in traditional alpine dairy systems, characterised by traditional feeding (mainly hay and concentrates) and use of summer pasture transhumance for part of the herd (Sturaro et al. Citation2013).
Data and editing
The initial data set comprised 6437 individual milk samples of 330 purebred OB cows collected during routine milk recording testing between January 2014 and December 2019. The list of purebred OB cows was retrieved from the Brown Cattle Breeders Federation of Bolzano Province (Südtiroler Braunviehzuchtverband, Bolzano, Italy). Milk fat (FC), protein (PC), casein (CC) and lactose content (LC), coagulation properties, casein (CN) fractions, minerals and fatty acid (FA) composition of OB cows were predicted from milk mid-infrared spectra in the laboratory of the South Tyrolean Dairy Association (Sennereiverband Südtirol, Bolzano, Italy). The laboratory was equipped with Milkoscan FT6000 (Foss, Hillerød, Denmark) and, from March 2017, with Milkoscan FT7 (Foss, Hillerød, Denmark). Somatic cell count (SCC) was determined by Fossomatic FC (Foss, Hillerød, Denmark). Coagulation properties and mineral composition were determined using the prediction models developed by Visentin et al. (Citation2016). Briefly, a data set composed of 923 milk samples from 60 single-breed herds containing the 4 major cattle breeds reared in the province of Bolzano was used in 2014 to develop prediction equations. In particular, the coefficients of determination in external validation were 0.54, 0.56, 0.52, 0.67, 0.69, 0.65, 0.40, and 0.68 for rennet coagulation time (RCT, min), curd firming time (k20, min), curd firmness (a30, mm), Ca, K, Mg, Na and P, respectively. Further, for the prediction of individual and groups of FA, the models developed by Gottardo et al. (Citation2017) were used. Overall, the prediction accuracies of these traits were moderate (Gottardo et al., Citation2017). Finally, CN fractions were predicted with the models developed by Niero et al. (Citation2016), who reported coefficients of determination in cross validation for α-CN, β-CN, and κ-CN that were 0.88, 0.60, and 0.74, respectively.
Information on MY (kg/d), days in milk (DIM), parity, herd and birth and calving date of cows was provided by the Breeders Association of Bolzano Province (Bolzano, Italy). Test-day MY, FC, PC, CC, LC, α-CN, β-CN and κ-CN fractions (% of crude protein), RCT, a30, k20, contents of Ca, K, P, Mg and Na (mg/kg), and FA composition (g/100 g total FA) were set to missing if they were outside the range mean ± 3 standard deviations. The FA groups were monounsaturated (MUFA), polyunsaturated (PUFA), saturated (SFA), unsaturated (UFA), trans (transFA), short chain (SCFA), medium chain (MCFA), long chain FA (LCFA), while individual FA were C14:0, C16:0, C18:0 and C18:1. The SCC was considered if comprised between 1000 and 10,000,000 cells/mL and it was log-transformed to somatic cell score (SCS) according to Ali and Shook (Citation1980). Records of cows were retained in the data set if they were within the window from 5 to 420 DIM. Parity ranged from 1 to 11. Furthermore, only cows with at least 3 test-day observations within lactation and herd-test-date with at least 3 cows sampled were kept for the analysis. The final data included 4034 test-day records of 237 cows (520 lactations) farmed in 23 South Tyrolean herds; 30, 23, 18, 16, and 13% of lactations belonged to parity 1, 2, 3, 4, and ≥5, respectively. Farm-specific information regarding feeding practices, use of pasture in summer and feed ration composition were not available in this study.
Statistical analysis
A mixed linear model was implemented in SAS software v. 9.4 (SAS Institute Inc., Cary, NC) to study the sources of variation of milk traits, as:
where yijklmn is the dependent variable (MY, milk composition traits, SCS, coagulation properties, CN fractions, minerals or FA), μ is the overall intercept of the model, seasoni is the fixed effect of the ith calving season of the cow [i = winter (December to February), spring (March to May), summer (June to August), autumn (September to November)], parityj is the fixed effect of the jth class of parity of the cow (j = 1 to 5, with the last class including parities from 5 to 11); stagek is the fixed effect of the kth class of stage of lactation of the cow (1 = 1 to 11, where the first class is 5 to 30 DIM, followed by 9 classes of 30 DIM each, and the last class including DIM from 301 to 420), (season × parity)ij is the fixed interaction effect between calving season and parity, (season × stage)ik is the fixed interaction effect between calving season and stage of lactation, (parity × stage)jk is the fixed interaction effect between parity and stage of lactation, HTDl is the random effect of the lth herd-test-date (l = 1 to 470), cowm is the random effect of the mth cow (m = 1 to 237), and eijklmn is the random residual. Differences between least squares means of the fixed effects were tested using Bonferroni post-hoc test (p < .05). Pearson’s correlations were calculated using the CORR procedure of SAS software v. 9.4 (SAS Institute Inc., Cary, NC). Considering DIM classes, the frequency of test-day records ranged from 5.8% (class 10) to 10.5% (class 2). Finally, the frequency of test-day records was 24.4, 25.9, 18.8, and 30.9% in winter, spring, summer, and autumn, respectively.
Results and discussion
Descriptive statistics
It is well-known that feeding practices (e.g., pasture) and feed composition can affect milk FC and PC in bovine milk. For example, the access to pasture and/or the availability of fresh forage may have an impact on milk FA profile. In fact, FA tend to be significantly different in extensive farming systems compared with intensive systems with diet uniquely based on unifeed, like the total mixed ration (Hanuš et al., Citation2018). For example, milk from cows at pasture tends to show greater content of n-3 FA and lower content of n-6 FA compared to milk from cows fed with total mixed ration (O'Callaghan et al. Citation2016). Cows in pasture-based systems produce milk with significant higher MUFA and PUFA content and lower SFA content compared with cows in indoor feeding systems (Hofstetter et al. Citation2014). Despite this, detailed information regarding access to summer pasture and other feeding practices were not available for the farms involved in the current study; in the light of these considerations, the comparisons of the present results with the literature should be considered with caution.
The highest frequency of calvings in primiparous cows was in autumn, whereas for multiparous cows the seasonal pattern was less evident (Figure ). The high incidence of autumn calvings in primiparous cows may be due to the management practices of alpine region. In fact, in alpine farms heifers usually spend the gestation at summer pastures before becoming lactating cows. This practice exploit the lower quality (energy content) of summer highland pastures that is reported to reduce the risk for high body condition score at calving (fat cows) and thus may limit the subsequent fertility and metabolic disorders caused by negative energy balance (Wathes et al. Citation2007; Roche et al. Citation2013).
Figure 2. Distribution of calvings of primiparous (dashed bar, n = 154) and multiparous (solid bar, n = 366) cows across calving seasons.
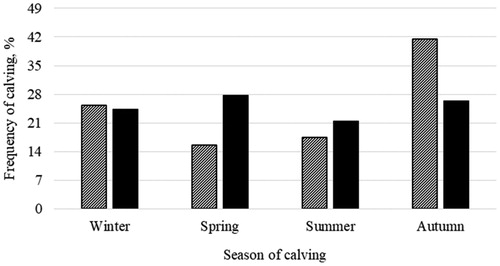
Descriptive statistics for MY, composition, SCS, coagulation properties, CN fractions, minerals and FA composition are summarised in Table . Average MY of OB cows was lower than mean MY of BS cows (24.10 kg/d) reported by Penasa et al. (Citation2014) and than average MY of 1232 dual-purpose Pinzgauer cows (20.87 kg/d) reported by Manuelian et al. (Citation2018c). The average FC was similar to that of Pinzgauer cows (4.01%; Manuelian et al. Citation2018c) but lower than that of BS cows (4.05%; Penasa et al. Citation2014). The coefficient of variation (CV) of traditional milk composition traits and SCS ranged from 4.6% (LC) to 62.5% (SCS) and resembled values observed in the literature for individual milk. In the present study, on average RCT was slightly greater and a30 was lower than values (18.57 min for RCT and 28.07 mm for a30) observed in multi-breed herds of Bolzano province (Visentin et al. Citation2015). Manuelian et al. (Citation2018a) analysed milk coagulation properties and minerals content of 851 Pinzgauer cows, and reported average values of 22.66 min, 5.53 min and 16.79 mm for RCT, k20 and a30, respectively, that is, less favourable than values observed in the current study. Cipolat-Gotet et al. (Citation2012) assessed milk coagulation properties in 913 BS cows and reported RCT (19.95 min) and k20 (5.36 min) that were similar to those of our study. However, in the same study, a30 was firmer (30.09 mm) compared to a30 of OB cows observed in the present study (Table ). This difference may be explained by the different methodology used to determine coagulation properties. In fact, Cipolat-Gotet et al. (Citation2012) conducted a lactodynamographic analysis with Formagraph , whereas in this study mid-infrared spectroscopy predicted data were used. The CV of coagulation properties ranged from 20.7% (RCT) to 57.7% (a30), i.e., higher than CV of RCT and a30 calculated by Manuelian et al. (Citation2018a).
Table 1. Descriptive statistics of milk yield, composition, somatic cell score, coagulation properties, casein fractions, minerals and fatty acid (FA) composition of Original Brown cows.
In the present study, α-CN was greater, and β-CN and κ-CN were lower compared to findings of Franzoi et al. (Citation2019). In purebred Simmental cows, De Marchi et al. (Citation2009) observed lower α-CN and κ-CN (42.02% and 9.24%) and greater β-CN (36.86%) than this study. The increase of CN fractions in milk has been shown to improve cheese-making properties by shortening RCT and increasing a30 (Jõudu et al. Citation2008). Therefore, the high α-CN and κ-CN content of OB milk suggests a good suitability for cheese production.
In terms of minerals, greater Ca and Mg and lower P, Na and K contents were found in OB milk compared to the study of Visentin et al. (Citation2018). In fact, the author reported averages of 1364.56 (Ca), 1668.64 (K), 140.63 (Mg), 1035.57 (P) and 442.83 mg/kg (Na) in 132,380 individual milk samples of Holstein Friesian, BS, Alpine Grey and Simmental cows. Comparing OB performance with those of Pinzgauer (Manuelian et al. Citation2018a), Ca, K and P were higher in OB milk. Overall, high contents of Ca, Mg and P in milk were shown to positively affect the coagulation ability and the stability of the micelles (Malacarne et al. Citation2014).
The overall means for the FA groups varied from 1.88 g/100 g total FA for transFA to 66.56 g/100 g total FA for SFA and CV ranged from 8.0% (SFA) to 38.8% (transFA). Means for individual FA varied from 10.17 g/100 g total FA for C18:0 to 29.76 g/100 g total FA for C16:0 with CV comprised between 12.7% (C16:0) and 22.4% (C18:0). Manuelian et al. (Citation2018c) observed slightly greater SFA content (69.20 g/100 g total FA) and slightly lower MUFA and PUFA (25.43 and 3.19 g/100 g total FA, respectively) in Pinzgauer compared to the present study. Similarly, OB produced milk with greater PUFA and MUFA and lower SFA content than other cattle breeds (Manuelian et al. Citation2019). In fact, Gottardo et al. (Citation2017) reported slightly greater SFA (70.18 g/100 g total FA) and PUFA (2.95 g/100 g total FA), and slightly lower MUFA (24.59 g/100 g total FA) in milk of BS cows compared to this study (Table ). Moreover, progressively greater SFA and lower PUFA and MUFA contents in milk have been observed when moving from cows with low (< 25%) to high (75 to 99%) proportion of BS genetics (Stergiadis et al. Citation2015). It should be considered that, next to genetics, the effect of diet composition has an impact on milk characteristics. In particular, the common practice of summer pasture in farms of alpine area significantly affects milk FA (O’Callaghan et al. 2016; Hanuš et al. Citation2018). Therefore, particular attention should be paid to feeding management and practices, and on the type of forage for producing milk with a desirable FA composition.
Correlations
Overall, correlations between conventional milk composition traits are in line with estimates present in the literature. The negative correlation between milk solid content and MY (Table ) was expected as there is a dilution effect on FC, PC and CC. The positive correlation between SCS and Na is supported by Costa et al. (Citation2019c) and Fernando et al. (Citation1985) . Indeed, Na reacts as an osmotic replacer of lactose during udder infection because permeability of alveolar epithelium changes and blood-milk barrier is disrupted (Wheelock et al. Citation1966; Wegner and Stull Citation1978; Costa et al. Citation2019b, Citation2019c). Summer et al. (Citation2009) reported greater Na and K contents in milk with SCC >400,000 cells/mL compared to milk with SCC <400,000 cells/mL, supporting the positive correlation between Na and SCS observed in this study. Supporting this, SCS and LC were moderately negatively correlated (-0.41) and Na and LC were strongly negatively correlated (−0.83; Table ). This confirmed low LC to be an potential indicator of udder infection and a useful tool for mastitis diagnosis (Costa et al. Citation2019b). The positive correlations of Ca, Mg and P with PC and CC are explained by the fact that these minerals are part of casein micelle structure and are mainly responsible for the casein micelle stability (Holt Citation2004; Bijl et al. Citation2013). Milk Ca, P and Mg were favourably associated with milk coagulation properties. Moreover, a30 and k20 showed favourable correlations with PC and CC (Table ), as described by Cassandro et al. (Citation2008) and Visentin et al. (Citation2018). A strong negative correlation was estimated between RCT and a30 (−0.72), in agreement with the literature (Ikonen et al. Citation2004; Cassandro et al. Citation2008). On the other hand, moderate negative correlations were observed between α-CN and MUFA (−0.40) and PUFA (−0.36), whereas κ-CN was positively associated with MUFA (0.29) and PUFA (0.32; Table ). Positive correlations of β-CN and α-CN fractions with SCFA and moderate negative correlation between κ-CN and SCFA were observed. On the contrary, the correlation between α-CN and LCFA was moderate and negative while the ones between β-CN and κ-CN fractions and LFCA were weak and positive. There is evidence that FA have an effect on size and structure of casein micelle. Panja et al. (Citation2018) have observed interactions of UFA and SFA with casein micelles; for instance, UFA increased more significantly the size and hydrophobicity of casein micelles than SFA. The correlations observed in our study and the results in Panja et al. (Citation2018) might provide useful information for future studies to improve milk technological traits.
Table 2. Pearson correlations between milk yield (kg/d), composition traits, coagulation parameters, casein fractions (% crude protein), and minerals (mg/kg).
Table 3. Pearson correlations between individual and groups of milk fatty acids (g/100 g total FA) with milk yield, composition traits, coagulation parameters, casein fractions (% crude protein), and minerals (mg/kg).
Effect of parity
Least squares means of milk traits for the fixed effect of parity are presented in Table . As in other breeds (Steinwidder and Greimel Citation1999; Miller et al. Citation2006; Siewert et al. Citation2019), daily MY increased from primiparous to multiparous cows, with a plateau from third parity onwards. Milk FC of second-parity cows was the highest but did not differ significantly from that of first-parity cows; PC of second- and third-parity cows were similar and CC was similar in the first 3 parities (Table ). On the contrary, LC was maximum in primiparous cows and decreased in subsequent lactations, in agreement with findings of Costa et al. (Citation2019c) in Holstein Friesian cows. The SCS increased steadily (p < .05) with parity, as the combined result of a decrease of polymorphonuclear leukocytes (Mehrzad et al. Citation2002; Rainard and Riollet Citation2006), age-related physiological alteration of the teat canal resulting in an expansion of the sphincter muscle at the teat tip, and altered epithelial permeability of mammary gland alveolar structures (Guarín and Ruegg Citation2016; Costa et al. Citation2019a, Citation2019b). Variation of CN fractions across parities was generally low, with significance likely related to the sample size. Milk RCT, a30 and k20 were the most favourable in first-parity cows (Table ). These results partly agreed with Penasa et al. (Citation2014), who reported milk coagulation properties to be worse in multiparous than primiparous cows. In the study of Ikonen et al. (Citation2004), a lower a30 was observed in primiparous than multiparous cows. Mineral content was generally greater in primiparous cows except for Na, whose trend was opposite (Table ) and confirmed results of Manuelian et al. (Citation2018b) and Visentin et al. (Citation2018). It is well known that the risk of hypocalcaemia is higher in multiparous cows because of the presence of a previous lactation, unfavourable cations-anions equilibrium in the ration of the dry period and reduced activity of parathyroid hormone which results in a disturbed Ca-homoeostasis with lower Ca mobilisation from bones to blood (Goff et al. Citation1986). The increase of Na across parities might be explained by the decrease of LC and the increase of SCS, as there is flow of extracellular liquid with high concentrations of Na inside alveolar lumen (Fernando et al. Citation1985; Batavani et al. Citation2007; Summer et al. Citation2009; Costa et al., 2019b). Both MUFA and PUFA were maximum in primiparous cows and decreased thereafter (p < .05). On the other hand, least squares means of SFA of multiparous were greater than that of primiparous cows (p < .05; Table ). These findings agreed with results of Manuelian et al. (Citation2018c) in Pinzgauer cattle, where SFA was the lowest in primiparous. Likewise, Gottardo et al. (Citation2017) observed decreasing values for MUFA and PUFA and increasing values for SFA from first- to later-parity Holstein, BS, Simmental and Alpine Grey cows. The MCFA and LCFA were the greatest in second and first parity, respectively, whereas SCFA was maximum in cows of parity ≥5 (Table ). In Gottardo et al. (Citation2017) greatest SCFA and LCFA were observed in primiparous cows and greatest MCFA in second-parity cows and cows in ≥ 5 parity. Similarly, Manuelian et al. (Citation2018c) observed greatest SCFA and LCFA in first- and greatest MCFA in sixed- and later-parity cows. Moreover, transFA were minimum and maximum in parity ≥ 5 and 1, respectively (p < .05; Table ). Finally, C18:0 and C18:1 were greater (p < .05) in first- than later-parity cows, whereas both C16:0 and C14:0 were the lowest in primiparous (Table ). Overall, FA profile tends to be more favourable in primiparous than multiparous OB cows, as there were higher healthy UFA contents in first parity and higher undesired SFA contents in milk of multiparous cows. In general, such findings are in line with the literature (Artegoitia et al. Citation2013; Bilal et al. Citation2014; Hanuš et al. Citation2018). Higher milk SFA in multiparous cows might be explained by greater amounts of de novo synthesised SFA in older animals (Artegoitia et al. Citation2013). In fact, Miller et al. (Citation2006) have observed metabolically less active mammary glands with a lower expression of fatty acid synthase and consequently lower de novo synthesised SFA in primiparous cows.
Table 4. Least squares means of milk yield, composition, somatic cell score, coagulation properties, casein fractions, minerals and fatty acid (FA) composition across parities.
Effect of lactation stage
The MY decreased almost linearly across DIM, with least squares means ranging from 23.58 (0.72) kg/d in early lactation (5 to 30 DIM) to 11.70 (0.29) kg/d in late lactation (301 to 420 DIM). Overall, the lactation curves of traditional milk quality traits followed the typical shape, with greaterFC, PC and CC at the end of lactation (Figure ). Milk SCS reached its nadir between 31 and 60 DIM, and then progressively increased until the end of lactation (Figure ); on the contrary, LC peaked in the window from 31 to 60 DIM and decreased thereafter (Figure ). These two inverse trends confirmed observations of Costa et al. (Citation2019b) and supported the negative correlation estimated between the two features. Both RCT and a30 slightly increased at the end of lactation (Figure ), as observed by Penasa et al. (Citation2014) and Visentin et al. (Citation2015). However, while a30 decreased rapidly from first to second stage of lactation, RCT had overall an increasing trend for the entire lactation, with the minimum in the first DIM (17.29 ± 0.33 min). As regards k20, the lactation curve was similar to that of LC, with a peak in second stage of lactation and subsequent decrease up to 4.40 ± 0.09 min (301 to 420 DIM); this trend mirrored findings of Visentin et al. (Citation2015). The β-CN fraction increased during lactation, whereas α-CN and κ-CN decreased (Figure ); in particular, the drop was more evident for α-CN, whose minimum and maximum were 0.43 ± 0.002 and 0.46 ± 0.002, respectively. Results resembled the findings reported by Franzoi et al. (Citation2019). Milk Ca, P, Mg, and Na contents increased from 60 DIM onwards, while K slightly decreased across lactation (Figure ); the same trend of milk K has been observed also by Manuelian et al. (Citation2018b) and Visentin et al. (Citation2018) in other dairy cattle breeds. As regards milk FA , both MUFA and PUFA exhibited similar lactation curves and decreased until 61-90 DIM with an increase thereafter (Figure ). In agreement with Manuelian et al. (Citation2018c), SFA increased until 61–90 DIM (peak) and then decreased until the end of lactation. Also, transFA increased at the beginning of lactation and then decreased, but in a more linear manner (Figure 4(b)). Lactation curves of SCFA, MCFA, LCFA and individual FA resembled the patterns observed in other breeds (Gottardo et al. Citation2017; Manuelian et al. Citation2018c). In particular, C14:0 and C16:0 increased up to 61-90 DIM and, after a plateau, they slightly decreased. Instead C18:0 and C18:1 were maximum in early lactation and then decreased till mid-lactation (Figure ).
Figure 3. Least squares means of (a) fat, protein and casein content, and (b) lactose content and somatic cell score (SCS) throughout lactation.
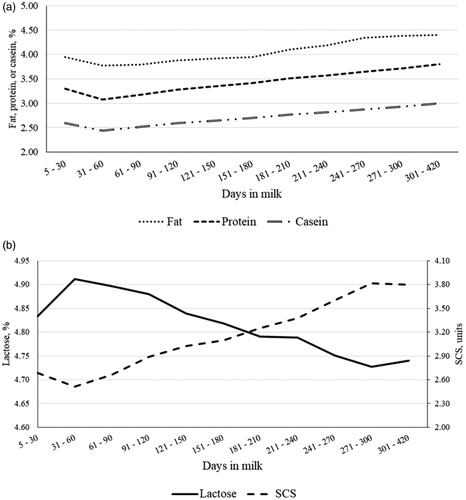
Figure 4. Least squares means of (a) rennet coagulation time (RCT), curd firmness 30 min after enzyme addition to milk (a30) and curd-firming time (k20), and (b) α-casein, β-casein and κ-casein fractions throughout lactation.
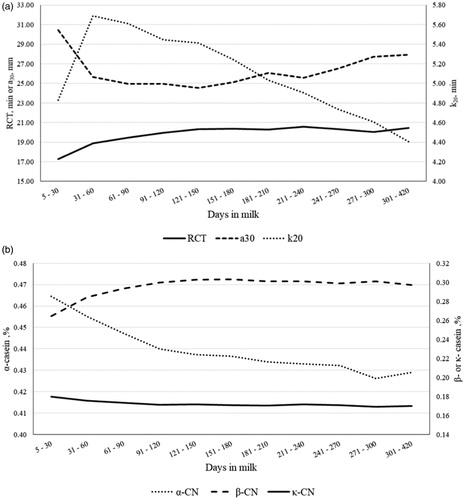
Figure 6. Least squares means of (a) monounsaturated (MUFA) and polyunsaturated fatty acids (PUFA), (b) saturated (SFA) and trans fatty acids (trans FA), (c) short-chain (SCFA), medium-chain (MCFA) and long-chain fatty acids (LCFA), and (d) individual fatty acids throughout lactation.
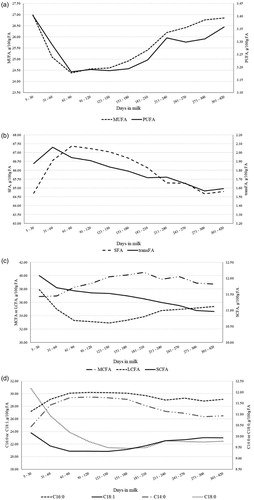
Parity-specific lactation curves were plotted for all milk traits (data not shown). Overall, primiparous clustered differently from multiparous in the case of MUFA, PUFA, SFA, SCS, LC and MY. Both MUFA and PUFA showed greatest content in primiparous cows at the very beginning and at the end of lactation, whereas SFA was the greatest between 61 and 90 DIM in second-parity cows. Regarding udder health-related traits, SCS was the highest throughout the entire lactation in multiparous cows, while, oppositely, LC was overall the highest in primiparous cows in all stages of lactation. Finally, MY was lower in primiparous cows during most of lactation but overlapped with MY of multiparous cows at the end of lactation.
Conclusions
The OB cattle breed is historically related to mountain farming system in alpine region of Italy. The present study provided the first comprehensive phenotypic investigation on milk composition and technological traits of OB population reared in mountain area. Overall, the comparison with the literature demonstrated that OB cows are able to compete with specialised dairy and other local (dual-purpose) cattle breeds in terms of milk quality and coagulation properties. In particular, milk of OB cows showed similar FA profile compared with BS and Alpine Grey cows and more favourable coagulation properties and mineral composition compared with Pinzgauer cows. However, it is worth to underline that OB cows are typically farmed in small farms with often low forage quality, summer pasturing and transhumance, and mostly old-fashioned tie-stall husbandry systems. Therefore, considering these aspects, OB cows may be even superior to other breeds, including BS, in terms of efficiency, resilience, adaptability, pasture use and rusticity. Results showed that multiparous cows were the most productive in terms of MY and primiparous cows were the most recommended for cheese-making in terms of coagulation properties. A more desirable FA composition (greater UFA and lower SFA) was observed in first-parity cows.Conservation strategies for this endangered breed may thus focus on the production of cheeses and dairy foods exclusively made with OB milk from extensive farming systems. Further investigations on cow functional traits are necessary to get better insights in OB in order to address future management choices and conservation plans. In perspective, specific experimental designs based on multi-breed herds and with detailed information on feeding practices would allow a fair comparison between OB and other cattle breeds.
Ethical approval
For this study data routinely recorded on milk samples were used and the approval of the Ethical Committee for the Care and Use of Experimental Animals of the University of Padova was not needed.
Acknowledgments
The authors thank the Brown Cattle Breeders Federation of Bolzano Province (Südtiroler Braunviehzuchtverband, Bolzano, Italy), the Breeders Association of Bolzano Province (Vereinigung der Südtiroler Tierzuchtverbände, Bolzano, Italy) and the South Tyrolean Dairy Association (Sennereiverband Südtirol, Bolzano, Italy) for providing data used in this study.
Disclosure statement
The authors declare that there is no conflict of interest associated with the paper. The authors are fully responsible for the content of the manuscript.
Correction Statement
This article has been republished with minor changes. These changes do not impact the academic content of the article.
Additional information
Funding
References
- Ali AKA, Shook GE. 1980. An optimum transformation for somatic cell concentration in milk. J Dairy Sci. 63(3):487–490.
- Artegoitia V, Meikle A, Olazabal L, Damián JP, Andrien ML, Mattiauda DA, Bermudez J, Torre A, Carriquiry M. 2013. Milk casein and fatty acid fractions in early lactation are affected by nutritional regulation of body condition score at the beginning of the transition period in primiparous and multiparous cows under grazing conditions. J Anim Physiol Anim Nutr. 97:919–932.
- Batavani RA, Asri S, Naebzadeh H. 2007. The effect of subclinical mastitis on milk composition in dairy cows. Iran J Vet Res. 8:205–211.
- Bijl E, van Valenberg HJF, Huppertz T, van Hooijdonk ACM. 2013. Protein, casein, and micellar salts in milk: Current content and historical perspectives. J Dairy Sci. 96(9):5455–5464.
- Bilal G, Cue RI, Mustafa AF, Hayes JF. 2014. Effects of parity, age at calving and stage of lactation on fatty acid composition of milk in Canadian Holsteins. Can J Anim Sci. 94(3):401–410.
- Braunvieh Schweiz. 2020. Phenotypic description of OB and description of breeding goals; [accessed 2020 January 15]. https://homepage.braunvieh.ch/xml_1/internet/de/application/d2/d466/f576.cfm
- Cassandro M, Comin A, Ojala M, Dal Zotto R, De Marchi M, Gallo L, Carnier P, Bittante G. 2008. Genetic parameters of milk coagulation properties and their relationships with milk yield and quality traits in Italian Holstein cows. J Dairy Sci. 91(1):371–376.
- Cipolat-Gotet C, Cecchinato A, De Marchi M, Penasa M, Bittante G. 2012. Comparison between mechanical and near-infrared methods for assessing coagulation properties of bovine milk. J Dairy Sci. 95(11):6806–6819.
- Costa A, Egger-Danner C, Mészáros G, Fuerst C, Penasa M, Sölkner J, Fuerst-Waltl B. 2019a. Genetic associations of lactose and its ratios to other milk solids with health traits in Austrian Fleckvieh cows. J Dairy Sci. 102(5):4238–4248.
- Costa A, Lopez-Villalobos N, Sneddon NW, Shalloo L, Franzoi M, De Marchi M, Penasa M. 2019b. Invited review: Milk lactose-Current status and future challenges in dairy cattle. J Dairy Sci. 102(7):5883–5898.
- Costa A, Visentin G, De Marchi M, Cassandro M, Penasa M. 2019c. Genetic relationships of lactose and freezing point with minerals and coagulation traits predicted from milk mid-infrared spectra in Holstein cows. J Dairy Sci. 102(8):7217–7225.
- De Marchi M, Bonfatti V, Cecchinato A, Di Martino G, Carnier P. 2009. Prediction of protein composition of individual cow milk using mid-infrared spectroscopy. Ital J Anim Sci. 8(sup2):399–401.
- Fernando RS, Spahr SL, Jaster EH. 1985. Comparison of electrical conductivity of milk with other indirect methods for detection of subclinical mastitis. J Dairy Sci. 68(2):449–456.
- Franzoi M, Niero G, Visentin G, Penasa M, Cassandro M, De Marchi M. 2019. Variation of detailed protein composition of cow milk predicted from a large database of mid-infrared spectra. Animals. 9(4):176.
- Gesellschaft zur Erhaltung alter und gefährdeter Haustierrassen (GEH). 2020. List of endangered livestock breeds; [accessed 2020 February 10]. http://www.g-e-h.de/index.php/die-geh1/rote-liste.
- Goff JP, Littledike ET, Horst RL. 1986. Effect of synthetic bovine parathyroid hormone in dairy cows: Prevention of hypocalcemic parturient paresis. J Dairy Sci. 69(9):2278–2289.
- Gottardo P, Penasa M, Righi F, Lopez-Villalobos N, Cassandro M, De Marchi M. 2017. Fatty acid composition of milk from Holstein-Friesian, Brown Swiss, Simmental and Alpine Grey cows predicted by mid-infrared spectroscopy. Ital J Anim Sci. 16(3):380–389.
- Guarín JF, Ruegg PL. 2016. Short communication: Pre- and postmilking anatomical characteristics of teats and their associations with risk of clinical mastitis in dairy cows. J Dairy Sci. 99(10):8323–8329.
- Hanuš O, Samková E, Křižová L, Hasoňova L, Kala R. 2018. Role of fatty acids in milk fat and the influence of selected factors on their variability-A review. Molecules. 23(7):1636.
- Hofstetter P, Frey HJ, Gazzarin C, Wyss U, Kunz P. 2014. Dairy farming: indoor v. pasture-based feeding. J Agric Sci. 152(6):994–1011.
- Holt C. 2004. An equilibrium thermodynamic model of the sequestration of calcium phosphate by casein micelles and its application to the calculation of the partition of salts in milk. Eur Biophys J. 33(5):421–434.
- Ikonen T, Morri S, Tyrisevä AM, Ruottinen O, Ojala M. 2004. Genetic and phenotypic correlations between milk coagulation properties, milk production traits, somatic cell count, casein content, and pH of milk. J Dairy Sci. 87(2):458–467.
- Jõudu I, Henno M, Kaart T, Püssa T, Kärt O. 2008. The effect of milk protein contents on the rennet coagulation properties of milk from individual dairy cows. Int Dairy J. 18(9):964–967.
- Malacarne M, Franceschi P, Formaggioni P, Sandri S, Mariani P, Summer A. 2014. Influence of micellar calcium and phosphorus on rennet coagulation properties of cows milk. J Dairy Res. 81(2):129–136.
- Manuelian CL, Penasa M, Visentin G, Benedet A, Cassandro M, De Marchi M. 2019. Multi-breed herd approach to detect breed differences in composition and fatty acid profile of cow milk. Czech J Anim Sci. 64(No. 1):11–16.
- Manuelian CL, Penasa M, Visentin G, Cassandro M, De Marchi M. 2018a. Phenotypic analysis of milk coagulation properties and mineral content of Pinzgauer cattle breed. Arch Anim Breed. 61(2):215–220.
- Manuelian CL, Penasa M, Visentin G, Zidi A, Cassandro M, De Marchi M. 2018b. Mineral composition of cow milk from multibreed herds. Anim Sci J. 89(11):1622–1627.
- Manuelian CL, Visentin G, Penasa M, Cassandro M, De Marchi M. 2018c. Phenotypic variation of milk fatty acid composition of Pinzgauer cattle breed. Ital J Anim Sci. 17(3):574–577.
- Marsoner T, Egarter Vigl L, Manck F, Jaritz G, Tappeiner U, Tasser E. 2018. Indigenous livestock breeds as indicators for cultural ecosystem services: A spatial analysis within the Alpine Space. Ecol Ind. 94:55–63.
- Maxa J, Neuditschko M, Russ I, Förster M, Medugorac I. 2012. Genome-wide association mapping of milk production traits in Braunvieh cattle. J Dairy Sci. 95(9):5357–5364.
- Mehrzad J, Duchateau L, Pyörälä S, Burvenich C. 2002. Blood and milk neutrophil chemiluminescence and viability in primiparous and pluriparous dairy cows during late pregnancy, around parturition and early lactation. J Dairy Sci. 85(12):3268–3276.
- Miller N, Delbecchi L, Petitclerc D, Wagner GF, Talbot BG, Lacasse P. 2006. Effect of stage of lactation and parity on mammary gland cell renewal. J Dairy Sci. 89(12):4669–4677.
- Niero G, Penasa M, Gottardo P, Cassandro M, De Marchi M. 2016. Short communication: Selecting the most informative mid-infrared spectra wavenumbers to improve the accuracy of prediction models for detailed milk protein content. J Dairy Sci. 99(3):1853–1858.
- O'Callaghan TF, Hennessy D, McAuliffe S, Kilcawley KN, O'Donovan M, Dillon P, Ross RP, Stanton C. 2016. Effect of pasture versus indoor feeding systems on raw milk composition and quality over an entire lactation. J Dairy Sci. 99(12):9424–9440.
- Panja S, Khatua DK, 2018. Investigations on the effect of fatty acid additives on casein micelles: role of ethylenic unsaturation on the interaction and structural diversity. ACS Omega. 3(1):821–830., Halder M.
- Penasa M, Tiezzi F, Sturaro A, Cassandro M, De Marchi M. 2014. A comparison of the predicted coagulation characteristics and composition of milk from multi-breed herds of Holstein-Friesian, Brown Swiss and Simmental cows. Int Dairy J. 35(1):6–10.
- Rainard P, Riollet C. 2006. Innate immunity of the bovine mammary gland. Vet Res. 37(3):369–400.
- Roche JR, Kay JK, Friggens NC, Loor JJ, Berry DP. 2013. Assessing and managing body condition score for the prevention of metabolic disease in dairy cows. Vet Clin North Am Food Anim Pract. 29(2):323–336.
- Siewert JM, Salfer JA, Endres MI. 2019. Milk yield and milking station visits of primiparous versus multiparous cows on automatic milking system farms in the Upper Midwest United States. J Dairy Sci. 102(4):3523–3530.
- Steinwidder A, Greimel M. 1999. Economic valuation of longevity of dairy cows. Die Bodenkultur. 50:235–249.
- Stergiadis S, Bieber A, Franceschin E, Isensee A, Eyre MD, Maurer V, Chatzidimitriou E, Cozzi G, Bapst B, Stewart G, et al. 2015. Impact of US Brown Swiss genetics on milk quality from low-input herds in Switzerland: interactions with grazing intake and pasture type . Food Chem. 175:609–618.
- Sturaro E, Marchiori E, Cocca G, Penasa M, Ramanzin M, Bittante G. 2013. Dairy systems in mountainous areas: Farm animal biodiversity, milk production and destination, and land use. Livest Sci. 158(1-3):157–168.
- Südtiroler Bauernbund (SBB) 2020. Description of financial support of agricultural and rural development; [accessed 2020 March 11]. https://www.sbb.it/service/foerderungen/umweltpr%C3%A4mie.
- Südtiroler Braunviehzuchtverband 2020. Description of the Original Brown breed; [accessed 2020 February 18]. https://www.braunvieh.it/it/razza-bruna-originale/descrizione-della-razza.html.
- Summer A, Franceschi P, Malacarne M, Formaggioni P, Tosi F, Tedeschi G, Mariani P. 2009. Influence of somatic cell count on mineral content and salt equilibria of milk. Ital J Anim Sci. 8(sup2):435–437.
- Visentin G, Penasa M, Gottardo P, Niero G, Isaia M, Cassandro M, De Marchi M.2015. Milk coagulation properties of cattle breeds reared in Alpine area. Poljoprivreda. 21(1 sup):237–240.
- Visentin G, Penasa M, Niero G, Cassandro M, De Marchi M. 2018. Phenotypic characterization of major mineral composition predicted by mid-infrared spectroscopy in cow milk. Ital J Anim Sci. 17(3):549–556.
- Visentin G, Penasa M, Gottardo P, Cassandro M, and De Marchi M. 2016. Predictive ability of mid-infrared spectroscopy for major mineral composition and coagulation traits of bovine milk by using the uninformative variable selection algorithm. J. Dairy Sci. 99:8137–8145. http://dx.doi.org/10.3168/jds.2016-11053
- Wathes DC, Fenwick M, Cheng Z, Bourne N, Llewellyn S, Morris DG, Kenny D, Murphy J, Fitzpatrick R. 2007. Influence of negative energy balance on cyclicity and fertility in the high producing dairy cow. Theriogenology. 68:S232–S241.
- Wegner TN, Stull JW. 1978. Relation between Mastitis Test Score, mineral composition of milk, and blood electrolyte profiles in Holstein cows. J Dairy Sci. 61(12):1755–1759.
- Wheelock J, Rook JAF, Neave FK, Dodd FH. 1966. The effect of bacterial infections of the udder on the yield and composition of cow’s milk. J Dairy Res. 33(2):199–215.
- Yoder DM, Lush JL. 1937. A genetic history of the Brown Swiss cattle in the United States. J Hered. 28(4):154–160.