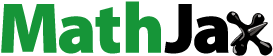
Abstract
The objective of this trial was to determine the amount of alfalfa hay (AH) in low forage diets that could be replaced with dried corn gluten feed (DCGF) without negatively altering eating behaviour, ruminal fermentation, nutrient digestibility, and performance of lactating dairy cows. Eight multiparous Holstein cows were used in a replicated 4 × 4 Latin square design experiment. Treatments included (dry matter [DM] basis): 18.81% AH without DCGF (0DCGF,); 12.53% AH and 6.26% DCGF (6DCGF); 6.26% AH and 12.53% DCGF (12DCGF); and 0% AH and 18.81% DCGF (18 DCGF). Diet particle size and physically effective neutral detergent fibre declined linearly (p < .001) as DCGF replaced AH resulting in a linear decrease (p < .001) in time (min/d) spent eating, ruminating, and chewing. As DCGF replaced AH, ruminal pH decreased linearly (p = .003) and total VFA concentrations increased linearly (p = .02) tending to be highest (p = .062) for 12DCGF. Ruminal acetate and butyrate, and plasma β-hydroxybutyrate concentrations declined linearly (p = .001) whereas ruminal propionate concentration increased linearly (p = .001) as DCGF increased. Dry matter intake (DMI; p = .001) and nutrient digestibility increased linearly (p < .05) and tended to be greatest (p < .10) for 12DCGF. Milk yield (MY; p = .016) and protein yield (p = .001) increased linearly, but milk fat yield declined as DCGF replaced 6 and 12% of AH and decreased more when DCGF replaced all AH resulting in a quadratic response (p = .041). Results of this trial suggest that replacing 12% of the AH with DCGF increases MY because of higher DMI and nutrient digestibility, but replacing all of the AH with DCGF reduces milk fat yield and dairy efficiency.
DCGF can replace AH to improve nutrient digestibility and milk yield.
Improvements in nutrient digestibility when DCGF replaces AH potentially reduce total waste production of dairy cows.
Completely replacing AH with DCGF in low forage diets reduces milk fat yield due to change in ruminal fermentation.
Highlights
Introduction
Corn gluten feed (CGF) is a co-product of wet milling corn and consists of bran and steep liquor (Poore et al. Citation2002). NRC (Citation2001) reported that dried CGF (DCGF) contained an average (dry matter [DM] basis) of 23.8% CP, 35.5% NDF, 3.5% EE, and 1.91 Mcal NEl/kg DM. The DCGF is used as a source of energy, protein, and fibre in diets fed to lactating dairy cows (Bernard et al. Citation1991) to replace concentrate or a combination of forage and concentrate depending on forage availability, feed prices, and other factors. Some researchers have reported no change in DM intake (DMI) or milk yield (MY) (Bernard et al. Citation1991; Schroeder Citation2003; Mullins et al. Citation2010) whereas others (Mullins et al. Citation2010) have reported increased DMI and MY when CGF replaced both forage and concentrate. Few studies have examined replacement of only the forage portion of the diet with CGF. Hao et al. (Citation2017) reported linear increases in DMI and MY with no changes in feed efficiency when alfalfa hay (AH) was replaced by 6.5 or 11% DCGF, but these researchers fed Chinese wild ryegrass to maintain adequate physically effective neutral detergent fibre (peNDF) concentrations.
Different responses to feeding non-forage fibre sources (NFFS) such as DCGF have been reported and are due, in part, to the fraction of the diet replaced (forage only or forage and concentrate) and the effect on the physical and chemical properties of the diet (Bradford and Mullins Citation2012). When NFFS replaces forage, the particle size of the diet is reduced which reduces chewing and saliva secretion increasing the likelihood of acidosis, laminitis, and milk fat depression (Heinrichs and Kononoff Citation2002; Beauchemin Citation2018). Greater sorting may occur when NFFS that have larger particle size replace finely ground grain or protein supplements potentially reducing DMI and MY (Heinrichs and Kononoff Citation2002; Beauchemin Citation2018). Therefore, maintaining adequate dietary peNDF when forage is replaced with NFFS can be a problem as reported by Rezac et al. (Citation2012) who replaced all of the dietary AH and corn silage with DCGF and tallgrass prairie hay so that diets only contained 19.2 or 13.8% forage. These authors concluded the lowest forage diet did not provide adequate peNDF to maintain rumen health, however, the diet containing 19.2% tallgrass prairie hay provided adequate peNDF to maintain ruminal function.
The concept of peNDF refers to the physical properties of the particle size of the dietary fibre (NDF) that affects chewing activity and saliva secretion (Beauchemin and Yang Citation2005; Plaizier et al. Citation2018). Replacing forage with NFFS reduces dietary peNDF that would be expected to reduce chewing activity and saliva secretion to buffer VFA production lowering rumen pH (Bradford and Mullins Citation2012). The effects of replacing forage other than corn silage with DCGF on eating behaviour have not been thoroughly examined as most researchers either maintained ≥29.6% forage (Schroeder Citation2003) or added other effective fibre sources to maintain peNDF (Rezac et al. Citation2012; Hao et al. Citation2017). Previous researchers have reported negative effects on dietary peNDF when forage was replaced with NFFS (Bradford and Mullins Citation2012) that could potentially reduce rumination activity (Mertens Citation1997), fibre digestibilty and perfornance (Allen Citation1997).
Alfalfa hay is commonly fed to lactating dairy cows as a source of effective fibre and digestible nutrients. However, when AH production is limited by drought, water restrictions or other circumstances, NFFS have been fed as a replacement in diets fed to lactating dairy cows. As production cost increase, producers are also looking for economical ingredients that will maintain rumen health and performance of lactating dairy cows. The optimum replacement of AH with DCGF in respect to production efficiency and rumen health of lactating dairy cows has not been identified. In research that has examined replacing AH with DCGF, dietary forage concentrations have been maintained at ≥ 29.6%, with most of the remaining forage provided by corn silage. We hypothesised that as DCFG replaces increasing amounts of AH lowering the amount of effective peNDF in the diet, reductions in chewing time, alter ruminal fermentation and nutrient digestibility would be observed resulting in lower performance. Our objective was to investigate replacing 33.3, 66.6, and 100.0% of the DM provided by AH in low forage diets with DCGF to determine the effect on chewing activity, rumen fermentation, plasma metabolites, nutrient digestibility, and performance of lactating dairy cows.
Materials and methods
The experiment was conducted at the dairy facilities of the Salehi-Saffari Farm, Tehran, Iran. Animal care was according to the guidelines of the Iranian Council of Animal Care (Citation1995) and all protocols were approved by the Institutional Animal Care Committee for Animals Used in Research before beginning the experiment.
Eight multiparous Holstein cows (BW = 623 ± 27.2 kg and DIM = 83 ± 7.2; mean ± SD) were used in a replicated 4 × 4 Latin square experiment with 21 d periods. Each period consisted of 14 d adaptation and 7 d for sample and data collection. Cows were housed in individual (4 × 4 m) stalls with beddings covered with sawdust and woodchips changed on daily basis. The corn silage based experimental diets were formulated so that AH was replaced by DCGF and had similar concentrate mixtures, but contained different proportions of AH and DCGF. Dietary treatments included (DM basis): 18.81% AH without DCGF (0DCGF); 12.53% AH and 6.26% DCGF (6DCGF); 6.26% AH and 12.53% DCGF (12DCGF); and 0% AH and 18.81% DCGF (18 DCGF). The four experimental diets were formulated to meet the requirements for energy, protein, minerals, and vitamins for the animals based on Cornell-Penn-Miner model (Boston et al. Citation2000). Ingredient composition of the experimental diets is outlined in Table . Cows were individually fed three times daily at 0800, 1600 and 2400 h in amounts that allowed 10% refusal. Diets were manually mixed and the amount fed and refused was recorded daily. Intake data were recorded on days 15–18 and used for statistical analysis.
Table 1. Ingredients and chemical composition (% of DM) of the experimental diets on a DM basis.
A sample of AH and DCGF was collected just prior to beginning the trial. Samples of AH, DCGF, and experimental diets were collected from day 15 to 18 and pooled within period. Samples of refusals were collected and pooled by cow within period. Samples were stored at −20 °C for analysis. Once thawed, samples were dried in a forced air oven for 48 h at 60 °C to determine DM and ground to pass through a 1-mm screen using a Wiley mill (Thomas Scientific, Swedesboro, NJ). Samples were analysed for crude protein (Kjeldahl method, AOAC Citation2002, method 955.04), ether extract (AOAC Citation2002, method 920.39), ash (AOAC Citation2002; method 942.05), NDF using heat-stable α-amylase (Van Soest et al. Citation1991), ADF (Robertson and Van Soest Citation1981) and starch (Zhu et al. Citation2016).
On d 18 and 19 of each period, particle size distribution for AH, DCGF, and TMR was determined using Penn State Particle Separator (PSPS) as described by Lammers et al. (Citation1996) for >19.0 mm and 19.0–8.0 mm screens and by Kononoff et al. (Citation2003) using the >19.0 mm, 19.0–8.0 mm, and 8.0–1.18 mm screens in triplicate. All samples were dried in a forced-air oven at 60 °C for 48 h to determine the DM of particles retained on each fraction. The physical effectiveness factor (pef) was calculated as the proportion of DM retained on two sieves (pef > 8; Lammers et al. Citation1996) and on three sieves (pef > 1.18; Kononoff et al. Citation2003) of the PSPS, respectively. The TMR NDF concentration was multiplied by the corresponding pef to determine the peNDF for two (peNDF > 8) and three sieves (peNDF > 1.18).
Cows were milked three times daily at 0730, 1530 and 2330 h. Milk weights were recorded at each milking. Milk samples were collected on days 15–17 of each period, preserved with potassium dichromate, and stored at 4 °C for analysis of milk fat, true protein, lactose, and MUN concentrations using a CombiScope FTIR 600 Dairy analyser (Delta Instruments, Netherlands). Yield of 3.5% fat-corrected (FCM) and energy-corrected (ECM) milk were calculated using the following equations (Tyrrell and Reid Citation1965):
Faecal samples were collected once daily from the rectum on days 15–18 each period (Kanani et al. Citation2015) after the 0800 feeding and stored at −20 °C until analysis. Samples were dried in a forced-air oven at 60 °C for 72 h, ground to pass through a 1-mm screen, and analysed for DM, ash, NDF, ADF, and CP, as described previously. Acid-insoluble ash was used as an inert dietary marker to determine nutrient digestibility (Van Keulen and Young Citation1977).
Measurements of eating, rumination, and chewing activities (sum of eating and rumination times) were recorded every 5 min on d 19 of each period. This activity was measured continuously for 24 h once every 5 min. Each activity (i.e. eating, ruminating, and resting) was assumed to last for the entire 5 min. Total eating and ruminating times were summed to determine total chewing time. Total time spent on chewing, eating or ruminating was divided by kg of DM, NDF, peNDF > 8 and peNDF > 1.18 consumed to determine activity per unit (kg/min) of each nutrient.
On d 20 of each period, 3 h after the 0800 feeding, whole blood was collected from the coccygeal vein into 7-mL tubes containing EDTA. Immediately after collection, tubes were placed in ice and transported to the laboratory where they were centrifuged at 3,000 × g for 15 min. Plasma was collected and stored in plastic tubes at −20 °C until analysis. Concentrations of glucose (glucose oxidase-phenol 4-aminoantipyrine peroxidase method, kit number 1500017), cholesterol (cholesterol oxidase-phenol 4-aminoantipyrine peroxidase method, kit number 1500010), triglyceride (glycerol-3-phosphate oxidase-phenol 4-aminoantipyrine peroxidase method, kit number 1500032), total protein (Biuret method, kit number 1500028), albumin (bromocresol green method, kit number 1500001), and plasma urea (Berthelot method, kit number 1400030) were measured using commercial kits (Pars Azmoon Co., Tehran, Iran) according to the manufacturer’s instruction. Concentrations of β-hydroxybutyrate (BHB) were determined using commercial colorimetric kit (kit number RB 1007; Randox Laboratories Ltd., Ardmore, UK). Globulin concentration was determined by calculating the difference between albumin and total protein.
On d 21 of each period, 3 h after the 0800 feeding, ruminal samples were collected using oral stomach tube. The first 100 mL of fluid was discarded and pH of the second sampling was immediately determined using (Sartorius Basic pH Metre PB-11, Sartorius AG, Goettingen, Germany). A 40 mL sample of ruminal fluid was acidified with 0.5% H2SO4 to lower the pH below 2.5 and immediately frozen at −18 °C until analysis. Upon thawing at room temperature, ruminal fluid was prepared for VFA analysis according to Alamouti et al. (Citation2014) using a gas chromatography (Agilent 6890 N, Santa Clara, CA, US) equipped with a capillary column (10.0 m × 0.53 mm × 1.00 μm). The concentration of ammonia N in the ruminal fluid was measured using phenol-hypochlorite technique (Broderick and Kang Citation1980).
In situ rumen degradation of DM and CP in the experimental diets was determined as described by Orskov et al. (Citation1980) using 3 ruminally cannulated Taleshi bulls. The bulls were fed a diet composed of 60% forage and 40% concentrate (DM basis). Samples of each experimental diet was dried using a forced-air oven at 60 °C and then ground to pass through a 3-mm screen using a Wiley Mill. Duplicate samples (5 g) of each experimental diet were weighed and placed in nylon bags (10 × 20 cm, 50-μm pore size) and placed in the rumen and incubated for 0, 4, 8, 16, 24, 48, 72, and 96 h. This resulted in a total of 14 samples per treatment per bull. The bags were removed from the rumen and placed under cold water tap until the water became transparent. Bags were dried at 60 °C for 48 h and then weighed. The DM and CP content of the residual samples were determined as previously described. The NEWAY computer program (Rowett Research Institute, Aberdeen, UK) based on the equations proposed by Orskov et al. (Citation1980) was used to determine degradability parameters. Degradability was calculated by the following equation:
where r = the rate of disappearance at time t (h), a = the rapidly degradable fraction (the soluble fraction), b = the slowly degradable fraction, and degraded at rate c (c > 0). The effective degradability (ED) was determined with the following equation: ED = a + b[c ÷ (c + k)], where k is the rate of passage assumed to be 0.04 and 0.05.
All data were subjected to analysis of variance using PROC MIXED (SAS Institute, 2002). The following model was used for the analysis as a replicated 4 × 4 Latin square design:
where Yijkl is the dependent variable; μ is the overall mean, Si is the fixed effect of square i; C(S)ij is the random effect of cow j within square i; Pk is the fixed effect of period k; Tl is the fixed effect of treatment l; Eijkl is the residual error. Polynomial orthogonal contrast statements were used to evaluate linear, quadratic, and cubic effects of treatments. No cubic differences (p > .10) were observed for any variable, therefore the p value for the cubic effect was not reported. The MIXED model was applied to compute and analyse in situ DM and CP degradation constants (a, b, c, and dg). In this model, each bull was considered a random effect while the treatment was applied as a fixed effect. Statistical significance was declared when p ≤ .05 and tendencies were declared at .05 ≥ p ≤ .10.
Results
As DCGF replaced AH, concentrations of CP, starch, ether extract, and NEL increased whereas ADF were decreased (Table ) compared with 0DCGF. The DCGF used for this trial had less ADF and more CP and EE compared with the AH (Table ). The proportion of particles retained on the >19.0 mm and 19.0–8.0 mm screens decreased linearly (p < .001) as DCGF replaced AH while the proportion of particles retained on the 8.0–1.18 mm screen and the pan (<1.18 mm) increased linearly (p < .001, Table ). The pef and peNDF retained on the 19.0–8.0 mm and 8.0–1.18 mm screens decreased linearly.
Table 2. Chemical composition (% of DM, mean ± SD) of alfalfa hay (AH) and dry corn gluten feed (DCGF) used in the experimental diets (n = 5).
Table 3. Effect of replacing alfalfa hay (AH) with dry corn gluten feed (DCGF) on particle distribution, physical effectiveness factor (pef), and physically effective NDF (peNDF) of experimental diets (n = 8)a.
The time spent eating, ruminating, and chewing decreased linearly (p < .001) as DCGF replaced AH (Table ). Similar linear decreases in time spent eating, ruminating, and chewing were also observed when expressed as min per unit of DMI, NDFI and peNDF > 1.8 as DCGF replaced AH. However, the time spent eating, ruminating, and chewing increased linearly as DCGF replaced AH when expressed as min per unit of peNDF > 8.
Table 4. Effect of replacing alfalfa hay with dry corn gluten feed on intake, rumination and chewing activity.
Ruminal pH decreased linearly (p = .003) as DCGF increased (Table ), but ruminal ammonia N exhibited a quadratic response (p = .077) as concentrations decreased with as DCGF replaced AH with the lowest value obtained for 12DCGF before increasing for 18DCGF. Total VFA concentrations increased linearly (p = .02) peaking for 12DCGF, but decreased for 18DCGF resulting in a trend for quadratic response (p = .062). As AH was replaced by DCGF, proportions of acetate (p = .001), butyrate (p = .001), isobutyrate (p = .027) and valerate (p = .022) decreased linearly whereas molar proportions of propionate increased linearly (p = .001). Because acetate decreased and propionate increased, the acetate to propionate ratio decreased linearly (p = .001).
Table 5. Effect of replacing alfalfa hay with dry corn gluten feed on ruminal pH, (volatile fatty acid) VFA, and ammonia-N.
Replacement of AH with DCGF had no impact on plasma concentrations of glucose, cholesterol, triglyceride, total protein, plasma urea N, albumin, and globulin (Table ). However, increasing DCGF in the experimental diets resulted in a linear decrease (p = .001) in plasma BHB concentrations.
Table 6. Effect of replacing alfalfa hay with dry corn gluten feed on blood metabolites.
Replacing AH with DCGF resulted in linear increased intake of DM (p = .001), organic matter (OM, p = .001), CP (p = .001) and NDF (p = .003); however, ADF intake decreased linearly (p = .001, Table ). Digestibility of DM (p = .031) and OM (p = .021) increased linearly; however, digestibility of CP (p = .086), NDF (p = .097) and ADF (p = .068) increased from 0DCGF to 12DCGF, but decreased for 18DCGF resulting in a trend for a quadratic response.
Table 7. Effect of replacing alfalfa hay with dry corn gluten feed on nutrient intake and apparent digestibility of nutrients.
Replacing AH with DCGF linearly increased (p = .016) milk yield which peaked at 12DCGF with no additional response observed for 18DCGF resulting in a trend for a quadratic response (p = .094, Table ). Yield of 3.5% FCM tended to increase (p = .077) linearly; however, no differences were observed among treatments in ECM yield. Milk fat yield declined linearly (p = .003) as DCGF replaced up to 12% of the AH and the decline was greatest for 18DCGF resulting in a quadratic response (p = .041). In contrast, milk protein yield increased linearly (p = .001). Milk lactose yield increased (p = .011) linearly as DCGF replaced up to 12% of the AH, but declined when 18% of the AH was replaced resulting in a quadratic (p =. 03) response. Milk fat percentage and the ratio of fat:protein decreased linearly (p < .001) as DCGF replaced AH.
Table 8. Effect of replacing alfalfa hay with dry corn gluten feed on milk yield, milk composition, and feed efficiency.
Concentrations of MUN exhibited a quadratic response (p = .042) as concentrations declined from 0DCGF to 12DCGF and then increased for 18DCGF. ECM/DMI (p = .005) and FCM/DMI (p = .004) decreased linearly as DCGF replaced AH.
As the proportion of DCGF in the diet increased, a fraction and c rate degradability of DM increased linearly (p < .001) while b fraction decreased linearly (p = .008, Table ). Effective degradability of DM was increased linearly (p < .001), but the extent of the increase was minimal when more than 12% of the AH was replaced with DCGF, similar response was observed for CP.
Table 9. Effect of replacing alfalfa hay with dry corn gluten feed on dry matter and crude protein degradationa.
Discussion
The DCGF fed in our current trial contained less CP and less NDF and ether extract compared with average values reported by NRC (Citation2001). Concentrations of CP in AH was slightly lower compared with average values published by NRC (Citation2001) resulting in a slight increase in dietary CP concentrations as DCGF replaced AH. Dietary NDF concentrations were similar for all diets, but forage NDF decreased from 19.30 to 11.76% as DCGF replaced AH. Ether extract concentrations increased as DCGF replaced AH which increased dietary NEl concentrations.
As expected, the particle distribution of DCGF was different from AH. No particles were retained on either the >19.0 mm or 19.0–8.0 mm screens and the greatest proportions of particles were retained on the 8.0–1.18 mm screen for DCGF. When DCGF replaced AH, particle size of the experimental diets decreased linearly. The particle size distribution is lower than recommended by White et al. (Citation2017b) to maintain rumen health for diets containing 30% NDF and 25% starch. Particles collected on the 19.0–8.0 mm screen contribute to the formation of the rumen mat and stimulate chewing (Heinrichs and Kononoff Citation2013) while particles retained on the 8.0–1.18 mm screen would contain more starch and fine-dense fibre from NFFS. Replacement of forage with NFFS reduces average particle size and dietary peNDF (Bradford and Mullins Citation2012). Insufficient peNDF reduces rumination, thereby reducing chewing time resulting in less saliva secretion and lower ruminal pH (Plaizier et al. Citation2018, NRC Citation2001). However, it should also be noted that the recommended peNDF for traditional rations differ from diets in which NFFS replaces forage because of differences in ingredient digestibility and dietary starch content, especially when corn silage is replaced by NFFS (Bradford and Mullins Citation2012).
Chewing behaviour and rumination time of dairy cows are influenced by different factors including particle size and forage NDF intake (Beauchemin Citation2018). In the present study, eating, rumination, and chewing times deceased linearly as particles retained on the >19.0 mm and 19.0–8.0 mm screens, peNDF (8 and 1.18), and forage NDF decreased as DCGF replaced AH consistent with reports by Biricik et al. (Citation2007). Eating time is influenced by DMI, physical form and chemical composition of the ration. In this study, eating time per unit of DM, NDF, or peNDF > 1.18 consumed declined as DCGF replaced AH whereas eating time per peNDF > 8 increased linearly. Intake of peNDF > 1.18 was relatively constant across treatments (average 6.01 kg/d), but intake of peNDF > 8 decreases from 3.00 to 2.15 kg/d for 0DCGF and 18DCGF, respectively which explains the differences in eating behaviour. High-forage diets require more time for eating per kg of DMI and result in more time for rumination per unit of DMI compared with low-forage diets (Beauchemin Citation2018) which is consistent with our results. White et al. (Citation2017a) reported a correlation of 0.38 between rumination time and the amount of particles retained on the 8-mm screen. In our current study, total chewing activity, which is the sum of eating and rumination times, as well as the adjusted total chewing per unit of DMI, NDFI, and peNDF > 1.18 decreased as DCGF replaced AH which is consistent with Biricik et al. (Citation2007).
Grant (Citation1997) reported that physically effective fibre stimulates chewing activity and secretion of saliva. Any imbalance between acid production and saliva secretion negatively affects ruminal pH (Allen Citation1997; Aschenbach et al. Citation2019). In the present study, ruminal pH decreased linearly as DCGF replaced AH. Previous studies have reported decreased ruminal pH with increasing proportions of CGF (Biricik et al. Citation2007; Mullins et al. Citation2010) unless peNDF was maintained. Hao et al. (Citation2017) did not observe a decrease in ruminal pH as CGF increased, but these researchers fed Chinese wild ryegrass to maintain peNDF. Long-particles from forage are fermented more slowly reducing the potential decline in ruminal pH compared with diets with smaller particles (Mullins et al. Citation2010). In our current trial, ruminal pH remained above 6 until all AH was replaced with DCGF which is consistent with previous research (Allen Citation1997). Decreased ruminal pH has also been linked to increased degradability of DM in DCGF (Lechartier and Peyraud Citation2011). As observed in our in situ analysis, degradability of the a fraction, degradability rate (c), and effective degradability of DM increased linearly as DCGF replaced AH.
Total VFA concentrations increased as DCGF replaced AH and were highest for 12DCGF before declining slightly for 18DCGF. Degradability of the a fraction increased linearly, but degradability of the b fraction decreased linearly which may explain the slight decline in total VFA for 18DCGF; however, total VFA concentrations for 18DCFG were still higher than 0DCGF. Allen (Citation1997) reported that diets with a higher proportion of dietary forage NDF would maintain greater ruminal mobility, potentially increasing mixed rumen contents to support higher ruminal VFA absorption (Allen Citation1997). The slight reduction in total VFA for 18DCGF is consistent with the response observed for total tract digestion of NDF and ADF. This may have been due to a slightly higher passage rate for 18DCGF given the greater proportion of smaller particles and higher DMI that would reduce the extent of ruminal fermentation.
Molar proportions of acetate and butyrate declined whereas propionate increased as DCGF increased which is consistent with previous studies (Biricik et al. Citation2007; Mullins et al. Citation2010). These changes are likely due to changes in particle size, ruminal DM and NDF digestibility DCGF and compared with AH. As DCGF replaced AH, dietary starch concentrations increased from 24.61 to 26.47% of DM and ruminal pH decreased linearly which may also contribute to the differences in individual VFA concentrations observed. These changes decreased the acetate-to-propionate ratio with increasing levels of DCGF in agreement with previous reports (Biricik et al. Citation2007; Sullivan et al. Citation2012). In our current experiment, molar proportions of butyrate declined linearly consistent with the findings reported by Hao et al. (Citation2017). Molar proportions of valerate increased as DCGF replaced AH reflecting the higher dietary CP content and digestibility consistent with previous reports (Mullins et al. Citation2010; Sullivan et al. Citation2012).
No differences were observed in plasma metabolites other than BHB which decreased linearly as DCGF replaced AH which is consistent with previous reports indicating that BHB was higher for cows fed high-forage diets compared with those fed low-forage diets. (Ranathunga et al. Citation2018). Plasma BHB concentrations observed in the current trial were within normal ranges reported for health lactating dairy cows (Voelker and Allen Citation2003; Benedet et al. Citation2019) and reflect butyrate available for oxidation by the liver (Kleinzeller Citation1943).
The linear increase in DMI as DCGF replaced AH is consistent with previous studies (Kononoff et al. Citation2003; Mullins et al. Citation2010; Sullivan et al. Citation2012; Hao et al. Citation2017). In contrast, Biricik et al. (Citation2007) did not report any change in DMI as DCGF increased in the diet. Replacing forage with NFFS has been reported to increase DMI (Allen and Grant Citation2000) due to reduced particle size of the diet and lower specific gravity of NFFS which increases passage rate and reduces ruminal fill (Giger-Reverdin Citation2000; Allen et al. Citation2019).
Total tract digestibility of CP, NDF, and ADF increased for 6 and 12% DCGF compared with 0DCGF, but no additional increase was observed for 18DCGF suggesting that 12% DCGF was optimal. The lack of improvement in CP, NDF, and ADF digestibility for 18DCGF was most likely due to lowered rumen pH and higher passage rate out of the rumen associated with smaller particle size and increased DMI (Grant Citation1997; de Souza et al. Citation2018). In contrast, Mohammadzadeh et al. (Citation2014) reported linearly increases in digestibility of DM, OM, CP, and NDF when soybean hulls replaced up to 30% of the dietary DM provided by forage and concentrates. Therefore, adequate peNDF from forage is necessary to prevent NFFS from passing out of the rumen too quickly and to allow more complete ruminal digestion (Grant Citation1997).
Milk yield increased from 39.3 to 41.5 kg/day as DCGF replaced AH consistent with previous research (Kononoff et al. Citation2006; Mullins et al. Citation2010; Hao et al. Citation2017). Calculated dietary energy concentrations increased as DCGF replaced AH (Table ) providing more energy to support higher milk and component yield. As DCGF replaced AH, DMI also increased providing additional energy in support of milk and component synthesis as well. Milk fat yield and percentage was lower for 18DCGF due to the lower reduced molar proportions of acetate and butyrate. Decrease milk fat percentage and yield have been reported previously (Boddugari et al. Citation2001; Cyriac Citation2005; Janicek et al. Citation2007), especially when forage was replaced by NFFS (Weiss Citation2012). Allen (Citation1997) reported that changes in milk fat percentage were a good indicator of maintenance of adequate effective fibre when NFFS were fed. Reduced ruminal pH is also positively correlated to reduced milk fat percentage (Zebeli et al. Citation2010). This is especially true for diets containing higher concentrations of linolenic acid that are common in corn byproducts (Janicek et al. Citation2007). Linolenic acid is subject to incomplete biohydrogenation which produces trans-10, cis-12 conjugated linolenic acid, especially at lower ruminal pH, causing depressed milk fat synthesis (Lock and Van Amburgh Citation2012).
Milk protein percentage and yield and milk lactose yield increased linearly reflecting greater energy intake, increased total VFA concentrations, and molar proportions of propionate. Feeding DCGF has been reported to improve microbial CP that would support greater milk protein synthesis (Hao et al. Citation2017). No differences were observed in lactose percentage which is consistent with previous reports (Bernard et al. Citation1991; Hao et al. Citation2017). Concentrations of MUN declined as AH was replaced by up to 12% DCGF, but MUN concentrations increased slightly for 18DCGF. These changes are consistent with the changes observed in ruminal ammonia concentrations. The slight increase in MUN observed for 18DCGF reflects the higher dietary CP observed for 18DCGF compared with 12DCGF. The changes in ruminal ammonia N concentrations area consistent with previous reports (Mullins et al. Citation2010; Sullivan et al. Citation2012). The protein in AH is highly degraded by ruminal microorganisms and without adequate rapidly fermentable carbohydrate to utilise the ruminal ammonia for microbial protein synthesis, the ammonia is absorbed across the rumen wall and converted into urea (Mullins et al. Citation2010). The increase in MUN observed for 18DCGF suggest that ruminal ammonia was not as efficiently utilised for microbial protein synthesis because of the tendency for lower total VFA concentrations compared with 12DCGF.
No differences were observed in yield of ECM, but yield of 3.5% FCM tended to decline linearly due to lower milk fat yield as DCGF replaced AH. Feed efficiency calculated as ECM/DMI and FCM/DMI decreased linearly as DCGF replaced AH. The decrease in efficiency was due to higher DMI and lower milk fat yield as DCGF replaced AH consistent with the results reported by Mullins et al. (Citation2010). Other researchers did not observe any change in feed efficiency when CGF was included diet (Schroeder Citation2003; Kononoff et al. Citation2006).
Conclusions
Under the conditions of this trial replacing all of the AH in the diet with DCGF was less desirable based on changes in ruminal fermentation and the slight reduction in fibre digestibility. Results of this trial indicate DCGF can replace up to 12% of the AH in a low forage diets to optimise ruminal fermentation, nutrient digestibility, and yield of 3.5% FCM. These results suggest some AH should be retained in the diet to provide more peNDF. As DCGF replaces AH, DMI and yield of milk protein increase with improved energy intake and nutrient digestibility; however, feed efficiency measured as FCM/DMI or ECM/DMI decreases. Results of this trial can be used by dairy producers considering a NFFS such as DCGF to replace AH hay due to high prices or limited supplies due to water scarcity.
Ethical approval
Animal care was according to the guidelines of the Iranian Council of Animal Care (1995) and all protocols were approved by the Institutional Animal Care Committee for Animals Used in Research before beginning the experiment.
Acknowledgements
The authors thank the director of Faravari Fructose NAB for supplying the corn gluten feed. The authors are also thankful to the president and staff at the Animal Science Research Institute of IRAN (ASRI) for the assistance they provided in conducting this experiment.
Disclosure statement
None of the authors had a personal or financial conflict of interest.
References
- Alamouti A, Alikhani M, Ghorbani G, Teimouri-Yansari A, Bagheri M. 2014. Response of early lactation Holstein cows to partial replacement of neutral detergent soluble fibre for starch in diets varying in forage particle size. Livest Sci. 160:60–68.
- Allen DM, Grant R. 2000. Interactions between forage and wet corn gluten feed as sources of fiber in diets for lactating dairy cows. J Dairy Sci. 83:322–331.
- Allen M, Sousa D, VandeHaar M. 2019. Equation to predict feed intake response by lactating cows to factors related to the filling effect of rations. J Dairy Sci. 102:7961–7969.
- Allen MS. 1997. Relationship between fermentation acid production in the rumen and the requirement for physically effective fiber. J Dairy Sci. 80:1447–1462.
- AOAC. 2002. Official methods of analysis. 17th ed. Arlington (VA): Association of Official Analytical Chemists.
- Aschenbach JR, Zebeli Q, Patra AK, Greco G, Amasheh S, Penner GB. 2019. Symposium review: the importance of the ruminal epithelial barrier for a healthy and productive cow. J Dairy Sci. 102:1866–1882.
- Beauchemin K, Yang W. 2005. Effects of physically effective fiber on intake, chewing activity, and ruminal acidosis for dairy cows fed diets based on corn silage. J Dairy Sci. 88:2117–2129.
- Beauchemin K. 2018. Invited review: current perspectives on eating and rumination activity in dairy cows. J Dairy Sci. 101:4762–4784.
- Benedet A, Manuelian C, Zidi A, Penasa M, De Marchi M. 2019. Invited review: β-hydroxybutyrate concentration in blood and milk and its associations with cow performance. Animal. 13:1676–1689.
- Bernard J, Delost R, Mueller F, Miller J, Miller W. 1991. Effect of wet or dry corn gluten feed on nutrient digestibility and milk yield and composition. J Dairy Sci. 74:3913–3919.
- Biricik H, Gencoglu H, Bozan B, Gulmez BH, Turkmen II. 2007. The effect of dry corn gluten feed on chewing activities and rumen parameters in lactating dairy cows. Ital J Anim Sci. 6:61–70.
- Boddugari K, Grant R, Stock R, Lewis M. 2001. Maximal replacement of forage and concentrate with a new wet corn milling product for lactating dairy cows. J Dairy Sci. 84:873–884.
- Boston R, Fox D, Sniffen C, Janczewski E, Munson R, Chalupa W. 2000. The conversion of a scientific model describing dairy cow nutrition and production to an industry tool: the CPM Dairy project. In: McNamara JP, France J, Beever DE, editors. Modelling nutrient utilization in farm animals. OXON (UK): CABI Publishing; p. 87–102.
- Bradford B, Mullins C. 2012. Invited review: strategies for promoting productivity and health of dairy cattle by feeding nonforage fiber sources. J Dairy Sci. 95:4735–4746.
- Broderick G, Kang J. 1980. Automated simultaneous determination of ammonia and total amino acids in ruminal fluid and in vitro media. J Dairy Sci. 63:64–75.
- Cyriac J. 2005. Effects of replacing forage fibre with non-forage fibre in lactating dairy cow diets. South Dakota State University.
- de Souza R, Tempelman R, Allen M, Weiss W, Bernard J, VandeHaar M. 2018. Predicting nutrient digestibility in high-producing dairy cows. J Dairy Sci. 101:1123–1135.
- Giger-Reverdin S. 2000. Characterization of feedstuffs for ruminants using some physical parameters. Anim Feed Sci Technol. 86:53–69.
- Grant R. 1997. Interactions among forages and nonforage fiber sources. J Dairy Sci. 80:1438–1446.
- Hao X, Gao H, Wang X, Zhang G, Zhang Y. 2017. Replacing alfalfa hay with dry corn gluten feed and Chinese wild rye grass: effects on rumen fermentation, rumen microbial protein synthesis, and lactation performance in lactating dairy cows. J Dairy Sci. 100:2672–2681.
- Heinrichs J, Kononoff P. 2002. Evaluating particle size of forages and TMRs using the new Penn State forage particle separator. Pennsylvania State University, College of Agricultural Sciences, Cooperative Extension DAS; p. 02–42.
- Heinrichs J, Kononoff P. 2013. The Penn state particle separator. University Park, PA: Penn State Extension DSE. 186:p. 1–8.
- Iranian Council of Animal Care. 1995. Guide to the care and use of experimental animals. Vol. 1. Isfahan (Iran): Isfahan University of Technology.
- Janicek B, Kononoff PJ, Gehman A, Karges K, Gibson M. 2007. Short communication: effect of increasing levels of corn bran on milk yield and composition. J Dairy Sci. 90:4313–4316.
- Kanani J, Philipp D, Coffey KP, Kegley EB, West CP, Gadberry S, Jennings J, Young AN, Rhein RT. 2015. Diurnal variation in fecal concentrations of acid-detergent insoluble ash and alkaline-peroxide lignin from cattle fed bermudagrass hays of varying nutrient content. J Anim Sci Biotech. 6:24.
- Kleinzeller A. 1943. The metabolism of butyric and related acids in animal tissue. Biochem J. 37:678–682.
- Kononoff P, Heinrichs AJ, Buckmaster D. 2003. Modification of the Penn State forage and total mixed ration particle separator and the effects of moisture content on its measurements. J Dairy Sci. 86:1858–1863.
- Kononoff PJ, Ivan S, Matzke W, Grant R, Stock R, Klopfenstein TJ. 2006. Milk production of dairy cows fed wet corn gluten feed during the dry period and lactation. J Dairy Sci. 89:2608–2617.
- Lammers B, Buckmaster D, Heinrichs AJ. 1996. A simple method for the analysis of particle sizes of forage and total mixed rations. J Dairy Sci. 79:922–928.
- Lechartier C, Peyraud JL. 2011. The effects of starch and rapidly degradable dry matter from concentrate on ruminal digestion in dairy cows fed corn silage-based diets with fixed forage proportion. J Dairy Sci. 94:2440–2454.
- Lock AL, Van Amburgh ME. 2012. Feeding for milk components. WCDS Adv. Dairy Technol. 24:265–277.
- Mertens D. 1997. Creating a system for meeting the fiber requirements of dairy cows. J Dairy Sci. 80:1463–1481.
- Mohammadzadeh H, Rezayazdi K, Nikkhah A. 2014. Effects of inclusion of graded amounts of soya bean hulls on feed intake, chewing activity and nutrient digestibility in dairy cows. J Anim Physiol Anim Nutr. 98:476–482.
- Mullins C, Grigsby K, Anderson D, Titgemeyer E, Bradford B. 2010. Effects of feeding increasing levels of wet corn gluten feed on production and ruminal fermentation in lactating dairy cows. J Dairy Sci. 93:5329–5337.
- NRC. 2001. Nutrient requirements of dairy cattle. 7th rev. ed. Washington (DC): National Academy of Sciences.
- Orskov E, Hovell F, Mould F. 1980. The use of the nylon bag technique for the evaluation of feedstuffs. Tropical Animal Production. 5:195–213.
- Plaizier J, Mesgaran MD, Derakhshani H, Golder H, Khafipour E, Kleen J, Lean I, Loor J, Penner G, Zebeli Q. 2018. Review: enhancing gastrointestinal health in dairy cows. Animal. 12:s399–s418.
- Poore MH, Johns JT, Burris WR. 2002. Soybean hulls, wheat middlings, and corn gluten feed as supplements for cattle on forage-based diets. Vet Clin North Am Food Anim Pract. 18:213–231.
- Ranathunga SD, Kalscheur KF, Anderson JL, Herrick KJ. 2018. Production of dairy cows fed distillers dried grains with solubles in low- and high-forage diets . J Dairy Sci. 101:10886–10898.
- Rezac DJ, Grigsby KN, Bello NM, Bradford BJ. 2012. Effects of varying rates of tallgrass prairie hay and wet corn gluten feed on productivity of lactating dairy cows. J Dairy Sci. 95:842–849.
- Robertson JB, Van Soest PJ. 1981. The detergent system of analysis and its application to human food. James WPT, Theander O, editors. The analysis of dietary fiber and food. New York (NY): Marcel Dekker; p. 123–158.
- SAS Institute. 2002. SAS user’s guide: statistics. Version 9.1. Cary (NC): SAS Institute Inc.
- Schroeder J. 2003. Optimizing the level of wet corn gluten feed in the diet of lactating dairy cows. J Dairy Sci. 86:844–851.
- Sullivan M, Grigsby K, Bradford B. 2012. Effects of wet corn gluten feed on ruminal pH and productivity of lactating dairy cattle fed diets with sufficient physically effective fiber. J Dairy Sci. 95:5213–5220.
- Tyrrell H, Reid J. 1965. Prediction of the energy value of cow’s milk. J. Dairy Sci. 48:1215–1223.
- Van Keulen J, Young B. 1977. Evaluation of acid-insoluble ash as a natural marker in ruminant digestibility studies. J Anim Sci. 44:282–287.
- Van Soest P, Robertson J, Lewis B. 1991. Methods for dietary fiber, neutral detergent fibre, and nonstarch polysaccharides in relation to animal nutrition. J Dairy Sci. 74:3583–3597.
- Voelker J, Allen M. 2003. Pelleted beet pulp substituted for high-moisture corn: 1. Effects on feed intake, chewing behavior, and milk production of lactating dairy cows. J Dairy Sci. 86:3542–3552.
- Weiss WP. 2012. Use of a corn milling product in diets for dairy cows to alleviate milk fat depression. J Dairy Sci. 95:2081–2090.
- White RR, Hall MB, Firkins JL, Kononoff PJ. 2017a. Physically adjusted neutral detergent fiber system for lactating dairy cow rations. I: deriving equations that identify factors that influence effectiveness of fiber. J Dairy Sci. 100:9551–9568.
- White RR, Hall MB, Firkins JL, Kononoff PJ. 2017b. Physically adjusted neutral detergent fiber system for lactating dairy cow rations. II: development of feeding recommendations. J Dairy Sci. 100:9569–9584.
- Zebeli Q, Mansmann D, Steingass H, Ametaj B. 2010. Balancing diets for physically effective fibre and ruminally degradable starch: a key to lower the risk of sub-acute rumen acidosis and improve productivity of dairy cattle. Livest Sci. 127:1–10.
- Zhu L, Jones C, Guo Q, Lewis L, Stark CR, Alavi S. 2016. An evaluation of total starch and starch gelatinization methodologies in pelleted animal feed. J Anim Sci. 94:1501–1507.