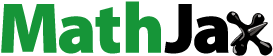
Abstract
This experiment aimed to establish the fatty acid profiles of oils from selected insect species and to evaluate their effects on in vitro rumen fermentation and methanogenesis. Insect samples, namely maggots, krotos, superworms, mealworms and crickets, were subjected to oil extraction using hexane in a Soxhlet apparatus. The fatty acid composition of the oils was subsequently determined. The insect oils were added at 5% dry matter to two kinds of diets, high forage (70% forage + 30% concentrate) and high concentrate (30% forage + 70% concentrate), which represented the diets of dairy cows and beef cattle, respectively. These diets, together with a control for each (without any addition of insect oil), were incubated in an in vitro rumen fermentation system, performed for three runs, with each run represented by two incubation units. Therefore, in total 2 × 6 treatments were incubated: the two types of diet (high forage and high concentrate) and the addition (or not) of insect oils (no addition, maggot, kroto, superworm, mealworm and cricket). The data were analysed by using two-way analysis of variance (ANOVA), followed by Tukey’s test. The results revealed that maggot oil was dominated by C12:0 (43.1% from total fatty acids), whereas the main fatty acids present in kroto oil were C18:1n-9 (38.8%) and C16:0 (20.8%). Superworm oil, mealworm oil and cricket oil were rich in C16:0 (19.5–31.2%), C18:1n-9 (25.8–44.6%) and C18:2n-6 (24.0–27.9%). In no case was the interaction between substrate and the addition of insect oil significant for any of the parameters. The addition of insect oil reduced the methane emission of the incubated substrates (p < .05) without altering total volatile fatty acid concentration, with mealworm oil resulting in the lowest level of methane among the insect oils. The oils had no significant effect on ruminal ammonia concentration. Generally, the addition of insect oil reduced the dry and organic matter digestibility of the substrates (p < .05). In conclusion, insect oils are rich in medium-chain and monounsaturated fatty acids (MUFA), and have the ability to mitigate enteric methane emission.
Oils from various insects, namely maggots, krotos, superworms, mealworms and crickets, were extracted, characterised for their fatty acid profiles, and evaluated for their effects on in vitro rumen fermentation and methanogenesis.
Maggot oil was dominated by C12:0, whereas the main fatty acids present in kroto oil were C18:1n-9 and C16:0. Superworm, mealworm and cricket oils were rich in C16:0, C18:1n-9 and C18:2n-6.
The addition of all the insect oils reduced methane emissions in the high forage and high concentrate substrates, without altering total volatile fatty acid concentration.
Highlights
Introduction
Insects have been considered as a promising future food and feed resource for humans and animals, respectively. The production of insect biomass as food and a feed resource has some comparative advantages over other resources; for example, insects are able to efficiently convert organic waste into their body mass, grow rapidly within a short period, require a low use of water and have low emissions of greenhouse gases (Van Huis Citation2013). They are typically characterised by their high protein content and have been considered as sustainable alternatives to conventional animal proteins in animal nutrition (Gasco et al. Citation2019; Citation2020). The crude protein contents of insects may vary depending on the species and developmental stage (Józefiak et al. Citation2016), and may also vary greatly due to variations in the nutritional quality of the rearing substrates (Makkar et al. Citation2014; Spranghers et al. Citation2017; Meneguz et al. Citation2018). For instance, our previous study indicated that the crude protein content of crickets (Gryllus bimaculatus), mealworms (Tenebrio molitor) and maggots (black soldier fly larvae, Hermetia illucens) ranged from 44.7 to 67.7% dry matter (Jayanegara et al. Citation2017). Part of the nitrogenous compounds present in insects is in the form of chitin, a long-chain polymer of an N-acetylglucosamine. This component is generally hardly digested in the digestive tract of animals (Chaudhari et al. Citation2011), although some animal species possess chitinase activity (Gasco et al. Citation2016; Tabata et al. Citation2018).
Insects have been used as a feed ingredient in a number of animal species, such as broiler chickens (Schiavone et al. Citation2017; Benzertiha et al. Citation2019), laying hens (Borrelli et al. Citation2017; Bovera et al. Citation2018), swine (Biasato et al. Citation2019) and aquatic animals (Belforti et al. Citation2015; Gasco et al. Citation2016). However, their utilisation in the feeding of ruminants has been limited. Evaluation of insects as ruminant feed has to date been limited, with only a few reports available (Jayanegara et al. Citation2017; Astuti et al. Citation2019). This may be related to the potential risk of the use of insects for ruminants causing bovine spongiform encephalopathy (BSE), or mad cow disease, although to date there has been no evidence of such a problem. EU regulations prohibit the use of protein from terrestrial animals in feed for food-producing animals, including insect protein (Finke et al. Citation2015). Many other countries, including developing regions, have less clear or even no, specific regulations concerning the use of insects and their derivatives for ruminant feeds (Bessa et al. Citation2020). In Indonesia, there are currently no regulations concerning the use of insects for animal feed, including that of ruminants.
A component which is predominantly high in insects is oil. It is widely known that oil can serve as an energy source for animals. As with protein, the oil content of insects varies widely according to the species, developmental stage and the rearing substrate (Sanchez-Muros et al. Citation2014; Nowak et al. Citation2016). For instance, the ether extract content of black soldier fly larvae, housefly maggots and mealworms have been shown to be within the range of 15.0–34.8, 9.0–26.0 and 31.1–43.1% dry matter, respectively (Makkar et al. Citation2014). The fatty acid composition of oils originating from insects has been explored and reported by a number of authors (for example Belforti et al. Citation2015; Spranghers et al. Citation2017; Meneguz et al. Citation2018). However, to the best of our knowledge, insect oils have yet to be investigated in terms their effects on rumen fermentation and methanogenesis.
This experiment therefore aimed to investigate the fatty acid profiles of oils from selected insect species and to evaluate their effects on rumen fermentation and methanogenesis by employing an in vitro rumen fermentation system.
Materials and methods
Insect samples and extraction procedure
The insect samples, i.e. maggots (Hermetia illucens, order Diptera, family Stratiomyidae), krotos (Oecophylla smaragdina, order Hymenoptera, family Formicidae), superworms (Zophobas morio, order Coleoptera, family Tenebrionidae), mealworms (Tenebrio molitor, order Coleoptera, family Tenebrionidae) and crickets (Gryllus bimaculatus, order Orthoptera, family Gryllidae) were purchased from commercial pet shops in Empang area, Bogor city. In Indonesia, these insect species are commonly fed to pets or companion animals, particularly birds, and are, therefore, widely sold in many areas in the country. However, it is not common to feed insects to ruminants in the country. The maggots and krotos were at larval stages, both at 14 d old. The superworms and mealworms were also at larval stages, at 90 and 50 d old, respectively. The crickets were 25 d old and at the nymph stage. Information regarding the age and developmental stage of the insect samples was obtained directly from the pet shops. A total of 3 kg (fresh matter) of each insect species was dried in an oven at 60 °C for 24 h. The dried samples were then ground using a hammer mill so that they passed through a 1 mm screen sieve. The ground insect samples were subjected to oil extraction by using Soxhlet apparatus, as described by Jayanegara et al. (Citation2018), for which hexane was the solvent used to extract the insect oils. Briefly, a 5 g sample was put into a cellulose thimble and then placed in an extraction chamber. Extraction was performed at boiling point for 6 h, and the remaining solvent was evaporated in an oven at 60 °C to generate the insect oils.
Substrate preparation and chemical analysis
The substrates used for the addition of the insect oils were king grass (KG, Pennisetum purpureum cv. king grass), concentrate for dairy cows (CD) and concentrate for beef cattle (CB). Fresh KG was manually chopped into 3 cm lengths and dried in an oven at 60 °C for 24 h. The dried KG was then milled in order for it to pass through a 1 mm screen. Both the CD and CB were purchased from a commercial supplier (CV Nuansa Baru, Bogor, Indonesia), and the KG was mixed with them to form a ration for dairy cows (RD) and one for beef cattle (RB), respectively. These concentrates composed of similar ingredients but at different proportions, i.e. soybean meal, copra meal, palm kernel meal, rice bran, wheat bran, corn grain, corn cobs, corn gluten feed and cassava pomace. The RD was a mixture of 70% KG + 30% CD (high forage diet), whereas RB was a mixture of 30% KG + 70% CB (high concentrate diet), on a dry matter basis. The proximate composition of the substrates was determined according to AOAC (Citation2005). The analyses included dry matter and ash (AOAC no. 942.05), crude protein (AOAC no. 977.02), and crude fat (AOAC no. 963.15). Neutral detergent fibre (NDF), acid detergent fibre (ADF) and acid detergent lignin (ADL) were determined according to Van Soest et al. (Citation1991), and the values were presented exclusively of residual ash. Non-fibrous carbohydrates (NFC) were obtained by subtracting crude protein, crude fat and NDF from organic matter. The nutrient composition of the KG, CD, CB, RD and RB is presented in Table .
Table 1. Nutrient composition of king grass (KG), concentrate for dairy cows (CD), concentrate for beef cattle (CB), ration for dairy cows (RD) and ration for beef cattle (RB) (% dry matter).
Determination of the fatty acid profiles in the insect oils was performed according to the procedure of AOAC (Citation2000). Prior to injection into a gas chromatograph (GC), the fatty acids were methylated into fatty acid methyl esters (FAME). This methylation was performed by adding BF3-MeOH reagent (Medina et al. Citation2007). The GC apparatus (Agilent 7890B, Agilent Technologies, Inc., Santa Clara, CA) was equipped with a Supelco SPTM 2560 capillary column (100 m × 0.25 mm × 0.2 µm) and a flame ionisation detector (FID). The temperature of the injector was set at 225 °C, whereas that of the detector was 240 °C. The carrier gas used was nitrogen, with a flow rate of 0.18 m/s and a split ratio of 1:100. Identification and quantification of the fatty acids were conducted by comparing the chromatograms of the samples with the FAME standard (Supelco 37 Component FAME Mix, Supelco Inc., Bellefonte, PA). Individual fatty acid values were expressed as a percentage of the corresponding total fatty acid value. All the chemical analyses were performed in duplicate and the data were presented descriptively.
In vitro rumen incubation
The substrates and insect oils were incubated in an in vitro rumen fermentation system following the procedure of Theodorou et al. (Citation1994). Rumen microbes were obtained from the rumen samples of two fistulated Ongole crossbred cattle, located in the Research Center for Biotechnology, Indonesian Institute of Sciences, Cibinong. The rumen samples were immediately brought to the laboratory within less than 15 min after being collected. The cattle were cared for according to the animal welfare standards of the Indonesian Institute of Science. Treatments were arranged as a factorial design, in which the first factor was the type of substrate (RD or RB) and the second factor was the type of insect oil added (no addition, maggot oil, kroto oil, superworm oil, mealworm oil or cricket oil). All the insect oils were added at a level of 5% from substrate dry matter, added to the two RD and RB substrates. This level was chosen based on our previous preliminary study regarding the effect of the supplementation level of maggot oil on rumen methanogenesis, in which the levels of 4 and 5% were effective to mitigate the methane emission while 3% and less had no effect (Jayanegara, Anzhany, et al. Citation2020). Prior to the addition, the insect oil was mixed with an emulsifier, specifically Tween 80. Of 500 mg of substrate was put into a serum bottle (150 mL capacity) as an incubation unit, and then 50 mL of rumen fluid-buffer mixture (1:2 v/v) was added. The bottle was gassed with CO2 in order to maintain an anaerobic environment, and closed with a rubber cap and an aluminium crimp seal. Incubation was performed in a water bath at 39 °C for 72 h.
Gas production was measured at 6, 12, 24, 48 and 72 h after the start of the incubation. Methane emission was measured at 24 h after incubation by injecting the gas into an infra-red methane analyser. Other fermentation parameters, such as pH, total volatile fatty acids (VFA) and ammonia concentrations, were also measured after 24 h incubation. The measurements were made according to the protocol described by Jayanegara et al. (Citation2016). The incubation residue was further incubated with pepsin–HCl solution for another 24 h. After the second incubation, all the incubation bottles were filtered with Whatman filter paper no. 41 under vacuum. The dry matter and organic matter content of the final residue were then determined (AOAC Citation2005, AOAC no. 942.05) in order to further calculate the in vitro dry matter digestibility (IVDMD) and in vitro organic matter digestibility (IVOMD), respectively. Incubation was performed in three runs (in consecutive weeks) and each run was represented by two incubation units.
Data analysis
The data were analysed using analysis of variance (ANOVA) with a factorial arrangement, in which the first factor was the substrate and the second the insect oil added. The following statistical model was employed:
where Yijkl is the observed value; µ is the grand mean; βi is the block effect (different incubation runs); αj is the effect of the substrate; γk is the effect of the insect oil addition; (αγ)jk is the interaction effect between the substrate and insect oil addition; and εijkl is the random error. Since the interactions for all the parameters were insignificant, only data from the main factor effects were presented. When a parameter was statistically significant at p < .05, it was followed by a post-hoc test, namely Tukey’s test. The statistical analysis was conducted employing SPSS software version 16.0 (SPSS Inc., Chicago, IL).
Results and discussion
Fatty acid profiles of insect oils
The oil contents of the maggots, krotos, superworms, mealworms and crickets were 29.1, 17.0, 37.2, 20.3 and 14.5% on a dry matter basis, respectively. Different insect oils had distinct fatty acid profiles (Table ). The maggot oil was dominated by C12:0 by more than 40% from total fatty acids. Other fatty acids that were high in maggot oil were C14:0, C16:0 and C18:1n-9. The main fatty acids present in kroto oil were C18:1n-9 and C16:0. Superworm oil, mealworm oil and cricket oil were similar with regard to their main fatty acid profiles, i.e. C16:0, C18:1n-9 and C18:2n-6, respectively. Omega three fatty acids were generally observed to be low in all the insect oils. The proportions of polyunsaturated fatty acids (PUFA) were low in the maggot oil and kroto oil, whereas they were high in the superworm, mealworm and cricket oil.
Table 2. Fatty acid profiles of insect oils from maggot, kroto, superworm, mealworm and cricket (% total fatty acids).
The high proportion of C12:0 observed in the maggot oil was in line with the findings of Spranghers et al. (Citation2017). Their study found that the C12:0 proportion of maggot (from total fatty acids) during the prepupae stage was 57.4, 43.7, 60.9 and 57.6% when grown on chicken feed, digestate, vegetable waste and restaurant waste, respectively. In another study, concentrations of C12:0 in maggot fed with organic wastes and agro-industrial by-products were 52.1–57.4% and 32.4–34.7%, respectively (Meneguz et al. Citation2018). The main fatty acids present in kroto were C18:1n-9 and C16:0, which constituted 50.0 and 22.3% of the total fatty acids respectively (Chakravorty et al. Citation2016), levels which were in agreement with this study. High proportions of C16:0, C18:1n-9 and C18:2n-6 in superworm oil, mealworm oil and cricket oil have also been reported by other authors (Jabir et al. Citation2012; Paul et al. Citation2017; Starčević et al. Citation2017). All these findings suggest that oils originating from these insects are particularly rich in medium-chain fatty acids (MCFA) and monounsaturated fatty acids (MUFA).
Effects of substrates on rumen fermentation and methanogenesis
Interaction between the substrate and added insect oil was not significant for all the in vitro parameters. During the early incubation period of samples of up to 48 h in the in vitro rumen fermentation system, gas production was higher for the RB substrate than for the RD (p < .05, Table ). Different substrates did not influence the pH value nor the total VFA concentration of the rumen microbial mixed culture medium (Table ). The incubation of the RB substrate resulted in a higher ammonia concentration than that of RD (p < .01). The RB substrate had higher DMD and OMD than the RD (p < .001, Table ). With regard to greenhouse gas methane emissions, the RB diet produced lower methane compared to the RD diet (p < .05).
Table 3. Influence of different substrates and insect oils on gas production (mL) at various incubation periods (h).
Table 4. Influence of different substrates and insect oils on fermentation of in vitro rumen microbial mixed culture.
Table 5. Influence of different substrates and insect oils on digestibility and methane emission of in vitro rumen microbial mixed culture.
The higher gas production, DMD and OMD of the RB substrate than the RD were apparently due to its high concentrate proportion, leading to a higher NFC and a lower NDF content. Gas in the in vitro rumen fermentation originated from two main sources: microbial degradation and fermentation on the substrate, and the buffering of VFA production (Getachew et al. Citation1998). The NFC fraction is more rapidly degraded and fermented than structural carbohydrate in the rumen, and therefore produces more gas, particularly during the initial incubation period, as well as higher DMD and OMD values. The higher ammonia concentration of the RB substrate is related to the slightly higher CP content, with CP in the rumen degraded to amino acids and subsequently fermented to generate ammonia (Kondo et al. Citation2014). In addition, concentrate typically contains a higher proportion of rumen degradable protein than forage, which contributes to higher ammonia formation (Jayanegara et al. Citation2016). Lower enteric methane emissions of high-concentrate as compared to high-forage diets have been repeatedly observed from both in vitro (Kim et al. Citation2018; Jayanegara, Yogianto, et al. Citation2020) and in vivo experiments (Lovett et al. Citation2003; Aguerre et al. Citation2011).
Effects of insect oils on rumen fermentation and methanogenesis
The addition of any of the insect oils generally had no effect on gas production, except for that of maggot oil, which reduced the gas production of substrates at 48 and 72 h incubation compared to the control (p < .05, Table ). Lower gas production with the addition of maggot oil was seemingly related to the negative effect of oil/fat on ruminal fibre digestion. A previous meta-analysis study showed that increasing dietary fat concentration linearly reduced dry matter and NDF digestibility (Patra Citation2013). The addition of insect oils had no significant effects on ruminal pH, total VFA and ammonia concentrations (Table ). Addition of some insect oils, i.e. mealworm and cricket oils reduced the DMD and OMD values of the substrates (p < .05, Table ). With regard to greenhouse gas methane emissions, the addition of insect oils decreased these (p < .05). The addition of mealworm oil resulted in the lowest methane emissions among the insect oils.
Oil or fat in general, regardless of its source, possesses a methane mitigating property. Fat supplementation from various sources had been demonstrated to linearly reduce methane emissions from cattle (Patra Citation2013). Inhibition of oil on methanogenesis is possible through a reduction in the population or activity of methanogens; partial elimination of protozoa (the host of some methanogens); and a decrease in nutrient degradation and fermentation, which contribute to hydrogen formation and, in the case of unsaturated fatty acids, serve as alternative hydrogen sinks (Beauchemin et al. Citation2009). The contribution of a decrease in methane by means of a reduction in nutrient digestibility is confirmed in this study, as indicated by the lower DMD and OMD values following the addition of insect oil. Variation in methane emissions depending on different insect oils is relatively low, indicating that the different types of fatty acids present in insect oils contribute to methane mitigation. In agreement with the findings of the study, MCFA, long-chain fatty acids, saturated fatty acids and unsaturated fatty acids were shown to be able to reduce enteric methane emissions (Fievez et al. Citation2003; Machmüller Citation2006; Patra Citation2013).
Conclusions
Different insect oils have distinct fatty acid profiles, but generally the oils are rich in MCFA (C12:0 and C16:0) and MUFA (C18:1n-9). Maggot and kroto oils contain low concentrations of PUFA, whereas these fatty acids are high in the superworm, mealworm and cricket oils. However, the omega three fatty acids are low in all the insect oils investigated. Despite their variations in the fatty acid profiles, the insect oils have the ability to mitigate enteric methane emissions when added to both high forage and high concentrate diets. Part of the methane decrease is apparently due to a reduction in nutrient digestibility as indicated by the lower DMD and OMD values following the addition of insect oils.
Ethical approval
All the procedures in this experiment have been approved by the Faculty of Animal Science, IPB University, Indonesia. The cattle used as rumen fluid donors were cared for according to the animal welfare standards of the Research Center for Biotechnology, Indonesian Institute of Sciences.
Disclosure statement
The authors declare that there is no conflict of interest.
Additional information
Funding
References
- Aguerre MJ, Wattiaux MA, Powell JM, Broderick GA, Arndt C. 2011. Effect of forage-to-concentrate ratio in dairy cow diets on emission of methane, carbon dioxide, and ammonia, lactation performance, and manure excretion. J Dairy Sci. 94(6):3081–3093.
- AOAC. 2000. Official methods of analysis, oil and fat. 17th ed. Arlington (VA): AOAC International.
- AOAC. 2005. Official methods of analysis. 18th ed. Arlington (VA): AOAC International.
- Astuti DA, Anggraeny A, Khotijah L, Suharti S, Jayanegara A. 2019. Performance, physiological status, and rumen fermentation profiles of pre- and post-weaning goat kids fed cricket meal as a protein source. Trop Anim Sci J. 42(2):145–151.
- Beauchemin KA, McGinn SM, Benchaar C, Holtshausen L. 2009. Crushed sunflower, flax, or canola seeds in lactating dairy cow diets: effects on methane production, rumen fermentation, and milk production. J Dairy Sci. 92(5):2118–2127.
- Belforti M, Gai F, Lussiana C, Renna M, Malfatto V, Rotolo L, De Marco M, Dabbou S, Schiavone A, Zoccarato I, et al. 2015. Tenebrio molitor meal in rainbow trout (Oncorhynchus mykiss) diets: effects on animal performance, nutrient digestibility and chemical composition of fillets. Ital J Anim Sci. 14:670–676.
- Benzertiha A, Kierończyk B, Rawski M, Józefiak A, Kozłowski K, Jankowski J, Józefiak D. 2019. Tenebrio molitor and Zophobas morio full-fat meals in broiler chicken diets: effects on nutrients digestibility, digestive enzyme activities, and cecal microbiome. Animals. 9(12):1128.
- Bessa LW, Pieterse E, Marais J, Hoffman LC. 2020. Why for feed and not for human consumption? The black soldier fly larvae. Compr Rev Food Sci Food Saf. 19(5):2747–2763.
- Biasato I, Renna M, Gai F, Dabbou S, Meneguz M, Perona G, Martinez S, Lajusticia ACB, Bergagna S, Sardi L, et al. 2019. Partially defatted black soldier fly larva meal inclusion in piglet diets: effects on the growth performance, nutrient digestibility, blood profile, gut morphology and histological features. J Anim Sci Biotechnol. 10(1):12.
- Borrelli L, Coretti L, Dipineto L, Bovera F, Menna F, Chiariotti L, Nizza A, Lembo F, Fioretti A. 2017. Insect-based diet, a promising nutritional source, modulates gut microbiota composition and SCFAs production in laying hens. Sci Rep. 7(1):16269.
- Bovera F, Loponte R, Pero ME, Cutrignelli MI, Calabrò S, Musco N, Vassalotti G, Panettieri V, Lombardi P, Piccolo G, et al. 2018. Laying performance, blood profiles, nutrient digestibility and inner organs traits of hens fed an insect meal from Hermetia illucens larvae. Res Vet Sci. 120:86–93.
- Chakravorty J, Ghosh S, Megu K, Jung C, Meyer-Rochow VB. 2016. Nutritional and anti-nutritional composition of Oecophylla smaragdina (Hymenoptera: Formicidae) and Odontotermes sp. (Isoptera: Termitidae): two preferred edible insects of Arunachal Pradesh. India. J Asia-Pac Entomol. 19(3):711–720.
- Chaudhari SS, Arakane Y, Specht CA, Moussian B, Boyle DL, Park Y, Kramer KJ, Beeman RW, Muthukrishnan S. 2011. Knickkopf protein protects and organizes chitin in the newly synthesized insect exoskeleton. Proc Natl Acad Sci USA. 108(41):17028–17033.
- Fievez V, Dohme F, Danneels M, Raes K, Demeyer D. 2003. Fish oils as potent rumen methane inhibitors and associated effects on rumen fermentation in vitro and in vivo. Anim Feed Sci Technol. 104(1-4):41–58.
- Finke MD, Rojo S, Roos N, van Huis A, Yen AL. 2015. The European Food Safety Authority scientific opinion on a risk profile related to production and consumption of insects as food and feed. J Insects Food Feed. 1(4):245–247.
- Gasco L, Acuti G, Bani P, Zotte AD, Danieli PP, De Angelis A, Fortina R, Marino R, Parisi G, Piccolo G, et al. 2020. Insect and fish by-products as sustainable alternatives to conventional animal proteins in animal nutrition. Ital J Anim Sci. 19(1):360–372.
- Gasco L, Biasato I, Dabbou S, Schiavone A, Gai F. 2019. Animals fed insect-based diets: state-of-the-art on digestibility, performance and product quality. Animals. 9(4):170.
- Gasco L, Henry M, Piccolo G, Marono S, Gai F, Renna M, Lussiana C, Antonopoulou E, Mola P, Chatzifotis S. 2016. Tenebrio molitor meal in diets for European sea bass (Dicentrarchus labrax L.) juveniles: growth performance, whole body composition and in vivo apparent digestibility. Anim Feed Sci Technol. 220:34–45.
- Getachew G, Blummel M, Makkar HPS, Becker K. 1998. In vitro gas measuring techniques for assessment of nutritional quality of feeds: a review. Anim Feed Sci Technol. 72(3–4):261–281.
- Jabir MDAR, Razak SA, Vikineswary S. 2012. Chemical composition and nutrient digestibility of super worm meal in red tilapia juvenile. Pak Vet J. 32:489–493.
- Jayanegara A, Anzhany D, Despal 2020. Maggot oil as a feed supplement for reducing methanogenesis of rumen microbial culture in vitro. IOP Conference Series: Earth and Environmental Science. https://www.scimagojr.com/journalsearch.php?q=19900195068&tip=sid&clean=0in press.
- Jayanegara A, Dewi SP, Laylli N, Laconi EB, Nahrowi N, Ridla M. 2016. Determination of cell wall protein from selected feedstuffs and its relationship with ruminal protein digestibility in vitro. Med Pet. 39(2):134–140.
- Jayanegara A, Harahap RP, Rozi RF. 2018. Effects of lipid extraction on nutritive composition of winged bean (Psophocarpus tetragonolobus), rubber seed (Hevea brasiliensis), and tropical almond (Terminalia catappa. Vet World. 11:446–451.
- Jayanegara A, Yantina N, Novandri B, Laconi EB, Nahrowi N, Ridla M. 2017. Evaluation of some insects as potential feed ingredients for ruminants: chemical composition, in vitro rumen fermentation and methane emissions. J Indonesian Trop Anim Agric. 42(4):247–254.
- Jayanegara A, Yogianto Y, Wina E, Sudarman A, Kondo M, Obitsu T, Kreuzer M. 2020. Combination effects of plant extracts rich in tannins and saponins as feed additives for mitigating in vitro ruminal methane and ammonia formation. Animals. 10(9):1531.
- Józefiak D, Józefiak A, Kierończyk B, Rawski M, Świątkiewicz S, Długosz J, Engberg RM. 2016. Insects – a natural nutrient source for poultry – a review. Ann Anim Sci. 16(2):297–313.
- Kim SH, Mamuad LL, Kim EJ, Sung HG, Bae GS, Cho KK, Lee C, Lee SS. 2018. Effect of different concentrate diet levels on rumen fluid inoculum used for determination of in vitro rumen fermentation, methane concentration, and methanogen abundance and diversity. Ital J Anim Sci. 17(2):359–367.
- Kondo M, Hirano Y, Ikai N, Kita K, Jayanegara A, Yokota H. 2014. Assessment of anti-nutritive activity of tannins in tea by-products based on in vitro Rumen Fermentation. Asian-Australas J Anim Sci. 27(11):1571–1576.
- Lovett D, Lovell S, Stack L, Callan J, Finlay M, Conolly J, O'Mara FP. 2003. Effect of forage/concentrate ratio and dietary coconut oil level on methane output and performance of finishing beef heifers. Livest Prod Sci. 84(2):135–146.
- Machmüller A. 2006. Medium-chain fatty acids and their potential to reduce methanogenesis in domestic ruminants. Agric Ecosyst Environ. 112(2–3):107–114.
- Makkar HPS, Tran G, Heuze V, Ankers P. 2014. State-of-the-art on use of insects as animal feed. Anim Feed Sci Technol. 197:1–33.
- Medina I, Aubourg S, Gallardo JM, Perez-Martin R. 2007. Comparison of six methylation methods for analysis of the fatty acid composition of albacore lipid. Int J Food Sci Technol. 27(5):597–601.
- Meneguz M, Schiavone A, Gai F, Dama A, Lussiana C, Renna M, Gasco L. 2018. Effect of rearing substrate on growth performance, waste reduction efficiency and chemical composition of black soldier fly (Hermetia illucens) larvae. J Sci Food Agric. 98(15):5776–5784.
- Nowak V, Persijn D, Rittenschober D, Charrondiere UR. 2016. Review of food composition data for edible insects. Food Chem. 193:39–46.
- Patra AK. 2013. The effect of dietary fats on methane emissions, and its other effects on digestibility, rumen fermentation and lactation performance in cattle: a meta-analysis. Livest Sci. 155(2–3):244–254.
- Paul A, Frederich M, Megido RC, Alabi T, Malik P, Uyttenbroeck R, Francis F, Blecker C, Haubruge E, Lognay G, et al. 2017. Insect fatty acids: a comparison of lipids from three Orthopterans and Tenebrio molitor L. larvae. J Asia-Pac Entomol. 20(2):337–340.
- Sanchez-Muros MJ, Barroso FG, Manzano-Agugliaro F. 2014. Insect meal as renewable source of food for animal feeding: a review. J Cleaner Prod. 65:16–27.
- Schiavone A, De Marco M, Martínez S, Dabbou S, Renna M, Madrid J, Hernandez F, Rotolo L, Costa P, Gai F, et al. 2017. Nutritional value of a partially defatted and a highly defatted black soldier fly larvae (Hermetia illucens L.) meal for broiler chickens: apparent nutrient digestibility, apparent metabolizable energy and apparent ileal amino acid digestibility. J Anim Sci Biotechnol. 8:51.
- Spranghers T, Ottoboni M, Klootwijk C, Ovyn A, Deboosere S, De Meulenaer B, Michiels J, Eeckhout M, De Clercq P, De Smet S. 2017. Nutritional composition of black soldier fly (Hermetia illucens) prepupae reared on different organic waste substrates. J Sci Food Agric. 97(8):2594–2600.
- Starčević K, Gavrilović A, Gottstein Z, Mašek T. 2017. Influence of substitution of sunflower oil by different oils on the growth, survival rate and fatty acid composition of Jamaican field cricket (Gryllus assimilis). Anim Feed Sci Technol. 228:66–71.
- Tabata E, Kashimura A, Kikuchi A, Masuda H, Miyahara R, Hiruma Y, Wakita S, Ohno M, Sakaguchi M, Sugahara Y, et al. 2018. Chitin digestibility is dependent on feeding behaviors, which determine acidic chitinase mRNA levels in mammalian and poultry stomachs. Sci Rep. 8(1):1461.
- Theodorou MK, Williams BA, Dhanoa MS, McAllan AB, France J. 1994. A simple gas production method using a pressure transducer to determine the fermentation kinetics of ruminant feeds. Anim Feed Sci Technol. 48(3-4):185–197.
- Van Huis A. 2013. Potential of insects as food and feed in assuring food security. Annu Rev Entomol. 58:563–583.
- Van Soest PJ, Robertson JB, Lewis BA. 1991. Methods for dietary fiber, neutral detergent fiber, and nonstarch polysaccharides in relation to animal nutrition. J Dairy Sci. 74(10):3583–3597.