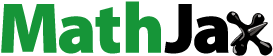
Abstract
The inclusion of by-products in composite pellet feeds could be a strategy to recycle nutritional components and a commercial opportunity for feeds industries. Aim of this study was to evaluate if the use of small amount of dried by-products containing polyphenols could alter rumen fermentation parameters and microbiota in dairy ewes. Thirty-six ewes (9 animals per group) were fed control diet (CON), and diets supplemented with 100 g/d per animal of tomato pomace (TP), 100 g/d per animal of grape marc (GM), and 75 g/d per animal of exhausted myrtle berries (EMB). Treatment showed significant effects only on pH, whereas sampling time affected almost all rumen fermentation parameters. No differences in the proportion of any phylum were detected in GM group compared to CON group. TP, compared to CON, evidenced higher abundance of Proteobacteria phylum (counts of 4.86; log fold chain of 5.61) which was dominated by Acetobacteraceae family with Acetobacter genus. The supplementation of EMB resulted in higher abundance of Succinivibrionaceae and lower abundance of Veillonellaceae family. Furthermore, in EMB group the Paraprevotellaceae and Prevotellaceae families dominated the phylum of Bacteroidetes. This phylum was significantly different between EMB and CON diet (counts of 57.06; log fold change of 1.10). The estimated production of methane and rumen fermentation parameters were not affected by the diets. The supplementation in small doses of GM, TP and EMB in the diets of sheep did not evidence a clear variation in rumen fermentation parameters nor changes in the general structure of rumen microbiota.
The agroindustrial by-products did not affect the rumen biochemical parameters.
The structure of bacteria community was influenced by EMB diet.
GM and TP diets did not increase rumen ammonia.
HIGHLIGHTS
Introduction
By-product feeds can be used to maintain acceptable animal performance while decreasing feed costs (Lourenco et al. Citation2016). Some agro-industrial by-products used in animal diets contain considerable amounts of the bioactive compound, such as polyphenols, that could affect rumen microbiota and rumen fermentation parameters. By-products from the winemaking process (e.g. grape marc, GM) (Spanghero et al. Citation2009), from the tomato industry (e.g. tomato pomace, TP), or those from the myrtle distillation process (e.g. exhausted myrtle berries, EMB) contain interesting amounts of polyphenols (Chedea et al. Citation2017; Correddu et al. Citation2019; Nudda et al. Citation2017) or others bioactive phytochemicals, such as sterols, tocopherols, carotenes (Kalogeropoulos et al. Citation2012). In ruminant diets, polyphenols affect the ruminal microbial populations, inducing community shifts in the bacteria, fungi and protozoa (Patra and Saxena Citation2009; Buccioni et al. Citation2015; Buccioni et al. Citation2017) and causing relevant changes in ruminal metabolism of nutrients (Patra and Saxena Citation2011; Buccioni et al. Citation2012; Cappucci et al. Citation2018). Polyphenols may decrease methanogenesis (Dschaak et al. Citation2011; Liu et al. Citation2011) and protein degradation in the rumen and increase protein production and protein flow to the duodenum targeting specific groups of rumen microbial populations (Patra and Saxena Citation2011). Furthermore, the by-products rich in polyphenols included in ruminant diets can reduce ammonia production (Bhatta et al. Citation2009; Theodoridou et al. Citation2010; Ishida et al. Citation2015). Tannins can interfere with membrane functions of rumen bacteria, binding enzymes, or inducing depletion of bioavailable iron by stable complexation (Patra and Saxena Citation2011). The in vivo and in vitro experiments investigating tannin effects on biohydrogenation showed inconsistent results due to the differences between polyphenols types, amount of polyphenols, and their association with other components of the diet (Buccioni et al. Citation2015; Buccioni et al. Citation2017). For instance, condensed tannins are not usually toxic to ruminants since they are not absorbed, but they may bind several nutrients irreversibly, making them unavailable to the animal, and they can also reduce the functionality of complex gastrointestinal enzymes (Smeriglio et al. Citation2017). On the other hand, the hydrolysable tannins may cause toxicity responses to ruminants when consumed at excessive amounts, whereas they could provide beneficial effects when used at low to moderate concentrations (Reed and Tanprasert Citation1995; Vasta et al. Citation2019). For example, grape marc used in a small dose in the diet of dairy sheep enhanced milk, protein, and fat yield (Nudda et al. Citation2017). In ruminants, the feeding approaches to improve the efficiency of energy and protein utilisation and minimise methane emissions and N excretion is strategic to enhance animal performances and reduce environmental impact. Considering the circular economy principles and the EU regulations on the feed use of food no longer intended for human consumption, the inclusion of agroindustrial by-products and food waste into animal diets could represent a solution to valorise and redirect them towards a more efficient re-use. The use of small doses of by-products in dry form could be an interesting resource to feed industries for their potential inclusion in pelleted formulations. This experiment was designed to evaluate if the inclusion of low dosages of by-products, such as grape marc, tomato pomace and exhausted myrtle berries, affects rumen fermentation parameters and ruminal microbiota populations of lactating dairy ewes.
Materials and methods
Animals and diets
Thirty-six Sarda dairy ewes were randomly allocated into 4 experimental groups (9 animals per group) as detailed in a previous publication (Nudda et al. Citation2017). Each group received a TMR as a basal diet (control group, CON) supplemented with 100 g of ground grape marc (GM group), 75 g of ground exhausted myrtle berries (EMB group), or 100 g of ground dry tomato pomace (TP group). The TMR was formulated to meet the energy and protein requirements of the ewes as calculated by the Small Ruminant Nutrition Model (Tedeschi et al. Citation2010). The chemical composition of the diets and of the by-products and the FA and phenol composition of by-products used in this experiment are extensively reported in Nudda et al. (Citation2017) and resumed in Supplemental Table S1.
Table 1. Effects of dietary treatments on rumen parameters.
Sampling and analysis
Rumen liquor sample collection
An individual sample of rumen liquor was collected on days 30 and 45 of the experimental period from the animals assigned to the 4 experimental groups. Rumen content was sampled 2 h after morning feeding using a stomach tube and an evacuation pump. To reduce saliva contamination, the first 30 mL of the rumen fluid was discarded. The sample of the rumen fluid was immediately filtered through sterile gauze. The pH was recorded after sampling using a pH metre (Orion 250 A, Orion Research Inc., Boston, MA) equipped with a glass electrode (model 238405, Hamilton Company, Reno, NV). Individual rumen liquor was divided in 3 aliquots: 2 tubes (10 mL each), added with 0.2 mL H2SO4 (50%) to acidify the sample, were stored to analyse ammonia and volatile fatty acids (VFA), respectively. Another aliquot (about 250 μL) was collected into a stool stabiliser tube included in the extraction Kit (PSP® Spin Stool DNA Kit) for subsequent DNA analysis.
Ammonia content and volatile fatty acids determination in rumen liquor
Ammonia and VFA contents in rumen liquor were determined according to Correddu et al. (Citation2015). Briefly, NH3 content was determined through the colorimetric method, by using a UV-Visible Spectrophotometer (Varian, Inc., Palo Alto, CA) following Chaney and Marbach (Citation1962) by substituting phenol with salicylate. The VFA was determined using a high-performance liquid chromatographic method according to Correddu et al. (Citation2015). The concentration of total and individual VFA was expressed as mmol/L and mol/100 mol of total VFA, respectively.
Estimation of methane emission
The methane emission was estimated according to the equation of Moss et al. (Citation2000):
where C2 is acetate; C3 is propionate and C4 is butyrate expressed as mol/100 mol of VFA.
Microbial analysis
DNA extraction
Total DNA was extracted from 250 μL of rumen liquor following the PSP method commercially available (PSP® Spin Stool DNA kit), already tested on sheep rumen samples (Henderson et al. Citation2013). Briefly, 1.4 mL of stabilised rumen sample was transferred into a 2-ml safe look tube, incubated for 10 min at 95 °C on a thermomixer at 900 rpm; 5 zirconia beads were added to the homogenate, and then the samples were centrifuged at 11,000 × g for 1 min at room temperature. The supernatant was transferred into InviAdsorb-Tube and vortexed vigorously (15 s). The suspension was incubated for 1 min at room temperature, and centrifuged at 14,000 × g, for 3 min at room temperature. The supernatant was transferred into a new tube containing proteinase K (25 μL) and incubated at 70 °C for 10 min in the thermomixer under continuous shaking at 900 rpm. The binding of the DNA was obtained by adding 200 μL of binding buffer to the lysate and immediately vortex. The whole mixture was transferred to the RTA-spin filter and, after incubation for 1 min at room temperature, it was centrifuged at 11,000 × g for 2 min. The filtrate and the tube were discarded, and the RTA Spin Filter was transferred into a new tube and washed two times with the buffer solutions prepared previously with kit tools. To eliminate any traces of ethanol the tube was centrifuged again. The RTA spin filter was placed in a new tube and added with elution buffer (preheated to 70 °C) and centrifuged (11,000 × g for 1 min) two times. The tube with filter (RTA-Spin filter) DNA was discarded and the total DNA was stored at −20 °C.
Molecular analysis of the bacterial population
The 16S rRNA Metagenomic Sequencing Library was prepared following the manufacturer’s protocol (Illumina Inc., San Diego, CA). Briefly, samples were amplified in the V3 and V4 regions using denaturated primers (Klindworth et al. Citation2013) in a limited cycle PCR, followed by an AMPure XP bead clean-up (A63880l; Beckman Coulter Inc., Brea, CA). A second PCR reaction was then performed to attach dual index and Illumina sequencing adapters using the Nextera XT Index Kit, followed by a final AMPure XP bead clean-up. Finally, library concentration was measured by fluorometric quantification using Qubit 2 (Invitrogen Inc., Carlsbad, CA) and each library was validated using a 1:50 dilution of the library on a Bioanalyzer DNA 1000 chip to verify sample size. Calculate DNA concentration in nM, based on the size of DNA amplicons, was used to pool the obtained 72 libraries in equimolar concentration. Pooled library was sequenced using the Illumina Miseq technology in 2 × 300 bp run with 5% of PhiX library as control. The sequences of raw data were filtered out and the reads were trimmed to a consistent length. Then, the data was denoised, chimaera filtered, and taxonomically assigned using DADA2 v1.1.5 (Callahan et al. Citation2016). For the taxonomic analysis, the sequencing reads were clustered into operational taxonomic units (OTUs) defined as groups of sequencing reads that differ by less than a fixed dissimilarity threshold (97%) generated in DADA2 using the Greengeenes database v13.5 (McDonald et al. Citation2012).
Statistical analysis
Rumen parameters
Data of rumen parameters (AGV, pH, NH3 and estimated methane production) were analysed with the PROC MIXED procedure of SAS (2002). The following mixed linear model was adopted:
where yijk is the rumen parameter; μ is the overall mean; diet is the fixed effect of i-th dietary treatment (CON, TP, EMB and GM); date is the fixed effect of the j-th sampling date (S1 and S2); dieti × datej is the interaction between i-th diet and j-th sampling date; animalk (dieti) is the random effect of the k-th animal nested within the i-th dietary treatment; eijk was the random residual error. Means were separated using the Tukey test and significance was declared at p < .05.
Microbial analysis
Microbial data were analysed using the software packages from the open-source Bioconductor project (Callahan et al. Citation2016). All data was collected into a phyloseq object (McMurdie and Holmes Citation2013) and used for further exploratory analysis. Briefly, alpha diversity (within-sample diversity) was assessed using Simpson and Shannon metrics on inverse Simpson diversity index (Simpson Citation1949; Hill Citation1973) and Shannon diversity index (Shannon and Weaver Citation2001).
Beta diversity (between-sample diversity comparison) was assessed with non-metric multidimensional scaling (NMDS) chosen to represent the dissimilarity between samples in a low-dimensional space based on Bray–Curtis dissimilarity matrix. Variance stabilising transformation (McMurdie and Holmes Citation2014) was used for 16S rRNA generated count data using DESeq2 package (Love et al. Citation2014). Each microbial group was tested individually, measuring its association with sample diet, and then jointly adjust p-values to ensure a False Discovery Rate (FDR) through the Benjamini–Hochberg procedure (Benjamini and Hochberg Citation1995).
Results
Rumen pH, VFA, methane and ammonia
The results of the effects of EMB, TP and GM on the concentration of total VFA, molar proportions of individual VFA, acetate:propionate ratio, NH3 and estimated methane production are presented in Table .
The ruminal pH ranged between 6.30 and 6.44 and it was not different between by-products diets and the CON one, whereas among by-products TP showed a lower pH compared to GM. All other parameters were not significantly influenced by any by-products compared among them and to CON indicating that, at the dosage utilised in this experiment, their polyphenolic compounds did not interfere on ruminal biochemistry. Time of sampling affected almost all rumen parameters, except for acetate mol incidence, propionate percentage and methane estimated emissions.
Regarding the interaction sampling time × treatment, only rumen ammonia content was significantly different (p < .01; Figure ). The rumen NH3 concentration did not change in GM and TP groups between the first and the second sampling, whereas increases in CON and EMB groups. Hydrolysable tannins followed by ellagic acid and gallic were the main components in EMB (Supplemental Table S2). The analysis of TP in our trial allowed the identification of naringenin and rutin as the major phenolic constituents (Supplemental Table S2. The main phenolic compounds in GM were gallic acid, catechin and anthocyanins (Supplemental Table S2).
Table 2. Diet’s effect on biodiversity indices of rumen microbial population.
Rumen bacterial population
The effects of the dietary by-products on the alpha diversity of rumen bacteria are shown in Table . The Shannon index, which measures the diversity of the bacterial communities, did not revealed difference between any by-product and the CON group; whereas significant differences between EMB and GM were evidenced (p < .05) The Shannon index of bacteria did not change between sampling time for any treated group. The Simpson’s index was significantly higher for EMB compared to GM at sampling 1, but any group receiving by-product differs from CON.
The plot of alpha-diversity indices is reported in Figure . In general, although the alpha diversity scores tended to be higher for the EMB group and lower for the GM group, there was no significant difference of species abundance in rumen microbiota among diets.
Figure 2. Violin plot of alpha diversity as measured with different metrics. Different colours indicate different diet treatments, violin shapes represent all possible results, with thickness indicating density. No significant differences at p < .01 were observed between groups.
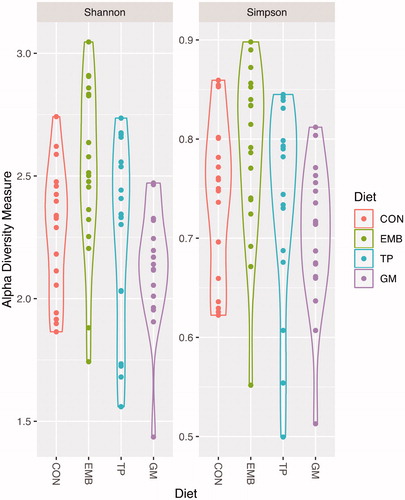
In terms of beta diversity, nonmetric dimensional scaling (NMDS) revealed statistical difference in the microbiota between sampling time (Figure ). Total genus abundance grouped by diet and separate for sampling time (Supplemental Figure S3(a)), and the Log transformed abundance and rank coloured by diet (Supplemental Figure S3(b)) confirm the differences only between sampling time.
Figure 3. Beta diversity. Non-metric multidimensional scaling (NMDS) based on Bray–Curtis dissimilarity matrix shows time as possible element of diversity between samples.
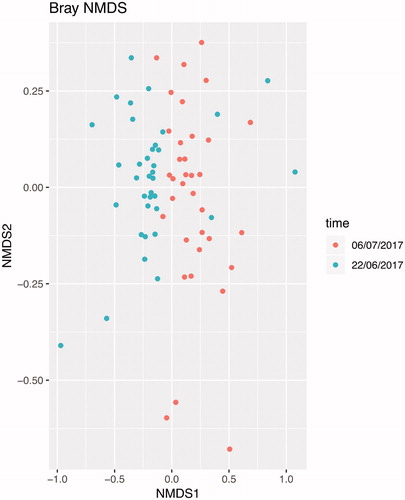
The Bacterial Community Structure is reported in . shows the top 10 bacterial families or genera ranked for DESeq2 results in EMB compared to CON diet, whereas the remaining taxa were not listed.
Table 3. Taxonomic identity of the rejected hypotheses in EMB vs CON Diet.
Table 4. Taxonomic identity of the rejected hypotheses in GM vs CON Diet.
Table 5. Taxonomic identity of the rejected hypotheses in TP vs CON Diet.
The rumen microbiota for the EMB versus CON diet was dominated by OTUs assigned to the Bacteroidetes, Proteobacteria, Cyanobacteria and Firmicutes phylum. The proportion of sequences assigned to the Bacteroidetes, Proteobacteria, and Cyanobacteria phyla was higher, whereas Firmicutes was lower in the EMB than CON group. In particular, supplementation of EMB resulted in higher abundance of Succinivibrionaceae family. Differences in OTU abundances were also detected for the Veillonellaceae family (Table ), whereas the Paraprevotellaceae and Prevotellaceae families dominated the phylum Bacteroidetes (Table ).
The comparison of GM with CON diet did not evidenced differences in the proportion of any phylum between the two groups.
Results of TP compared to CON group, evidenced the higher abundance of Proteobacteria phylum (Table ). This phylum was dominated by Acetobacteraceae family with the genus Acetobacter.
Discussion
The inclusion of by-products in ruminant diets can affect rumen function, protein and fibre digestion and microbial population. Values of ruminal pH were within the normal range for rumen liquor pH in Sarda sheep (Correddu et al. Citation2019) and was not influenced by treatments compared to the control. No differences were observed, also in the molar proportion of rumen VFA among treatments, in line with previous findings in sheep diet by using both condensed (Hervás et al. Citation2003), and hydrolysable tannins (Liu et al. Citation2011). In addition, some phenolic compounds as gallic and ellagic acids present in the by-products () may be metabolised to acetic and butyric acids, compensating for a possible contrasting effect of some polyphenols on the formation of these VFA.
The effects of pure polyphenol or inclusion of by-products containing polyphenol in animal diet did not give univocal results on protein digestion. Some authors observed a decrease in protein degradation with polyphenols in ruminants (Abarghuei et al. Citation2010; Dschaak et al. Citation2011). This process is caused by the ability of polyphenols to bind protein, reducing the microbial enzymes activity and decreasing the growth of proteolytic bacteria (Molan et al. Citation2001). In the present study, the rumen NH3 remained stable in GM and TP compared to CON diet. The increase of rumen ammonia to the EBM was surprising because hydrolysable tannins, at dose of 1–3% dietary DM in sheep has been found to reduce ruminal NH3 concentration (Liu et al. Citation2011). In addition, gallic acid one of the main components of HT, has been found to reduce NH3 in beef (Aboagye et al. Citation2019). Several rumen microorganisms, have the ability to degrade gallic acid to acetate and butyrate (Vasta et al. Citation2019), and this could be one of the reasons of the ineffectiveness of EMB to affect protein degradation. However, a recent in vitro study showed that the abundance and the efficiency of rumen microorganisms able to reduce gallic acid are very low in rumen liquor (Mannelli et al. Citation2019) and therefore, others dietary components may have interfered. Moreover, the pattern of NH3 in TP group does not agree with previous observation in beef cattle where rumen ammonia increases with the inclusion of dried tomato, as an alternative to soybean meal, in a dose (2 g of dried tomato pomace per kg of body weight; Yuangklang et al. Citation2010) similar to the one used in our experiment (2.2 g/kg of body weight; Nudda et al. Citation2017). Therefore, the discrepancy in the results could be related to the species-specific differences and/or to basal diet.
Data about the microbial population of rumen fluid showed that the supplementation of EMB evidenced higher abundance of Succinivibrionaceae family, Veillonellaceae family, and Paraprevotellaceae and Prevotellaceae families dominated the phylum Bacteroidetes. These bacteria have been reported to contain urease genes and exert urease activity (Patra and Aschenbach Citation2018), and they have been associated to low methane emissions in Tammar wallabies (Pope et al. Citation2011). Veillonellaceae family are considered involved in the production of propionate as its major fermentation product (Kishimoto et al. Citation2006). The TP group had higher abundance of Proteobacteria phylum, dominated by Acetobacteraceae family with the genus Acetobacter, which has been found positively correlated with CH4 emissions in dairy heifers (Cunha et al. Citation2019). This could explain the tendency for highest CH4 emission per kg of DMI and per kg of milk observed in TP group. Differences in the rumen microbiota between sampling could reflect the variation of numbers and diversity of microbial population according to the changes in dry matter intake (Carberry et al. Citation2012), that in our study decrease significantly from the first to the second sampling (1.78–1.66 kg/d of DM, respectively; p < .05). The lower dry matter intake could be reflecting the evolution of energy requirement during the natural evolution of the lactation curve of Sarda dairy ewes (Pulina et al. Citation2007).
Conclusions
The inclusion of low doses of by-products rich in polyphenols in the diets of dairy sheep did not cause consistent change in rumen parameters nor variations in the structure of rumen microbiota in comparison to CON diet. Moreover, the by-products used in the low dose in the trial, did not evidence any effects on estimated methane emitted by in dairy sheep. Almost all biochemical and physiochemical parameters and the structure of the microbiota showed a clear difference between sampling time, related to the evolution of feed intake during the experiment. Finally, the use of these by-products at low dosage did not evidence any negative effects on rumen bacteria or animal performance, suggesting their potential use in commercial feed.
Ethical approval
The data used in the present study were obtained from an experiment carried out according to the Italian laws on animal experiments and ethics (LD 04/03/2014, n.26).
Author contributions
G. Buffa conducted the experiment as a part of her PhD; N. P. Mangia and D. Licastro conducted and interpreted the microbiological part of this experiment; A. Cesarani and S. Sorbolini elaborated the data; G. Pulina reviewed the paper; A. Nudda conceived and wrote the paper. The responsibility for what is written in this paper rests with all authors.
Supplemental Material
Download MS Word (1 MB)Acknowledgements
The authors thank Gesumino Spanu, Antonio Fenu, Antonio Mazza, Alessandra Marzano for technical assistance during the experiment and Roberto Rubattu for help in laboratory analysis. The authors also thank the PhD students of Animal Science Unit of Department of Agricultural, who gave a valuable support in animal feeding and sample collection, and to Mr. Emiliano Deligios for animal care.
Disclosure statement
No potential conflict of interest was reported by the author(s).
Additional information
Funding
References
- Abarghuei MJ, Rouzbehan Y, Alipour D. 2010. The influence of the grape pomace on the ruminal parameters of sheep. Livest Sci. 132(1–3):73–79.
- Aboagye IA, Oba M, Koenig KM, Zhao GY, Beauchemin KA. 2019. Use of gallic acid and hydrolyzable tannins to reduce methane emission and nitrogen excretion in beef cattle fed a diet containing alfalfa silage. J Anim Sci. 97(5):2230–2244.
- Benjamini Y, Hochberg Y. 1995. Controlling the false discovery rate: a practical and powerful approach to multiple testing. J R Stat Soc Series B Stat Methodol. 57(1):289–300.
- Bhatta R, Uyeno Y, Tajima K, Takenaka A, Yabumoto Y, Nonaka I, Enishi O, Kurihara M. 2009. Difference in the nature of tannins on in vitro ruminal methane and volatile fatty acid production and on methanogenic archaea and protozoal populations. J Dairy Sci. 92(11):5512–5522.
- Buccioni A, Pallara G, Pastorelli R, Bellini L, Cappucci A, Mannelli F, Minieri S, Roscini V, Rapaccini S, Mele M, et al. 2017. Effect of dietary chestnut or quebracho tannin supplementation on microbial community and fatty acid profile in the rumen of dairy ewes. Biomed Res Int. 2017:4969076.
- Buccioni A, Decandia M, Minieri S, Molle G, Cabiddu A. 2012. Lipid metabolism in the rumen: new insights on lipolysis and biohydrogenation with an emphasis on the role of endogenous plant factors. Anim Feed Sci Technol. 174(1–2):1–25.
- Buccioni A, Pauselli M, Viti C, Minieri S, Pallara G, Roscini V, Rapaccini S, Trabalza Marinucci M, Lupi P, Conte G, et al. 2015. Milk fatty acid composition, rumen microbial population, and animal performances in response to diets rich in linoleic acid supplemented with chestnut or quebracho tannins in dairy ewes. J Dairy Sci. 98(2):1145–1156.
- Callahan BJ, Sankaran K, Fukuyama JA, McMurdie PJ, Holmes SP. 2016. Bioconductor workflow for microbiome data analysis: from raw reads to community analyses. F1000Res. 5:1492.
- Carberry CA, Kenny DA, Han S, McCabe MS, Waters SM. 2012. Effect of phenotypic residual feed intake and dietary forage content on the rumen microbial community of beef cattle. Appl Environ Microbiol. 78(14):4949–4958.
- Cappucci A, Alves SP, Bessa RJB, Buccioni A, Mannelli F, Pauselli M, Viti C, Pastorelli R, Roscini V, Serra A, et al. 2018. Effect of increasing amounts of olive crude phenolic concentrate in the diet of dairy ewes on rumen liquor and milk fatty acid composition. J Dairy Sci. 101(6):4992–5005.
- Chaney AL, Marbach EP. 1962. Modified reagents for determination of urea and ammonia. Clin Chem. 8:130–132.
- Chedea VS, Pelmus RS, Lazar C, Pistol GC, Calin LG, Toma SM, Dragomir C, Taranu I. 2017. Effects of a diet containing dried grape pomace on blood metabolites and milk composition of dairy cows. J Sci Food Agric. 97(8):2516–2523.
- Correddu F, Nudda A, Battacone G, Boe R, Francesconi AHD, Pulina G. 2015. Effects of grape seed supplementation, alone or associated with linseed, on ruminal metabolism in Sarda dairy sheep. Anim Feed Sci Technol. 199:61–72.
- Correddu F, Fancello F, Chessa L, Atzori AS, Pulina G, Nudda A. 2019. Effects of supplementation with exhausted myrtle berries on rumen function of dairy sheep. Small Rumin Res. 170:51–61.
- Cunha CS, Marcondes MI, Veloso CM, Mantovani HC, Pereira LGR, Tomich TR, Dill-McFarland KA, Suen G. 2019. Compositional and structural dynamics of the ruminal microbiota in dairy heifers and its relationship to methane production. J Sci Food Agric. 99(1):210–218.
- Dschaak CM, Williams CM, Holt MS, Eun JS, Young AJ, Min BR. 2011. Effects of supplementing condensed tannin extract on intake, digestion, ruminal fermentation, and milk production of lactating dairy cows. J Dairy Sci. 94(5):2508–2519.
- Henderson G, Cox F, Kittelmann S, Miri VH, Zethof M, Noel SJ, Waghorn GC, Janssen PH. 2013. Effect of DNA extraction methods and sampling techniques on the apparent structure of cow and sheep rumen microbial communities. PLoS One. 8(9):e74787.
- Hervás G, Frutos P, Giráldez FJ, Mantecón AR, Álvarez Del Pino MC. 2003. Effect of different doses of quebracho tannins extract on rumen fermentation in ewes. Anim Feed Sci Technol. 109(1–4):65–78.
- Hill MO. 1973. Diversity and evenness: a unifying notation and its consequences. Ecology. 54(2):427–432.
- Ishida K, Kishi Y, Oishi K, Hirooka H, Kumagai H. 2015. Effects of feeding polyphenol-rich winery wastes on digestibility, nitrogen utilization, ruminal fermentation, antioxidant status and oxidative stress in wethers. Anim Sci J. 86(3):260–269.
- Kalogeropoulos N, Chiou A, Pyriochou V, Peristeraki A, Karathanos VT. 2012. Bioactive phytochemicals in industrial tomatoes and their processing byproducts. Lebensm Wiss Technol. 49(2):213–216.
- Kishimoto A, Ushida K, Phillips GO, Ogasawara T, Sasaki Y. 2006. Identification of intestinal bacteria responsible for fermentation of gum arabic in pig model. Curr Microbiol. 53(3):173–177.
- Klindworth A, Pruesse E, Schweer T, Peplies J, Quast C, Horn M, Glöckner FO. 2013. Evaluation of general 16S ribosomal RNA gene PCR primers for classical and next-generation sequencing-based diversity studies. Nucleic Acids Res. 41(1):e1.
- Liu H, Vaddella V, Zhou D. 2011. Effects of chestnut tannins and coconut oil on growth performance, methane emission, ruminal fermentation, and microbial populations in sheep. J Dairy Sci. 94(12):6069–6077.
- Lourenco JM, DiLorenzo N, Stelzleni AM, Segers JR, Stewart RL, Jr. 2016. Use of by-product feeds to decrease feed cost while maintaining performance of developing beef bulls. Prof Anim Sci. 32(3):287–294.
- Love MI, Huber W, Anders S. 2014. Moderated estimation of fold change and dispersion for RNA-seq data with DESeq2. Genome Biol. 15(12):550.
- Mannelli F, Daghio M, Alves SP, Bessa R, Minieri S, Giovannetti L, Conte G, Mele M, Messini A, Rapaccini S, et al. 2019. Effects of chestnut tannin extract, vescalagin and gallic acid on the dimethyl acetals profile and microbial community composition in rumen liquor: an in vitro study. Microorganisms. 7(7):202.
- McDonald D, Price MN, Goodrich J, Nawrocki EP, DeSantis TZ, Probst A, Andersen GL, Knight R, Hugenholtz P. 2012. An improved Greengenes taxonomy with explicit ranks for ecological and evolutionary analyses of bacteria and archaea. ISME J. 6(3):610–618.
- McMurdie PJ, Holmes S. 2013. Phyloseq: an R package for reproducible interactive analysis and graphics of microbiome census data. PloS One. 8(4):e61217.
- McMurdie PJ, Holmes S. 2014. Waste not, want not: why rarefying microbiome data is inadmissible. PLoS Comput Biol. 10:1003531.
- Molan AL, Attwood GT, Min BR, McNabb WC. 2001. The effect of condensed tannins from Lotus pedunculatus and Lotus corniculatus on the growth ofproteolytic rumen bacteria in vitro and their possible mode of action. Can J Microbiol. 47(7):626–633.
- Moss AR, Jouany JP, Newbold J. 2000. Methane production by ruminants: its contribution to global warming. Ann Zootech. 49(3):231–253.
- Nudda A, Correddu F, Atzori AS, Marzano A, Battacone G, Nicolussi P, Bonelli P, Pulina G. 2017. Whole exhausted berries of Myrtuscommunis L. supplied to dairy ewes: effects on milk production traits and blood metabolites. Small Rumin Res. 155:33–38.
- Patra AK, Saxena J. 2009. Dietary phytochemicals as rumen modifiers: a review of the effects on microbial populations. Antonie Van Leeuwenhoek. 96(4):363–375.
- Patra AK, Saxena J. 2011. Exploitation of dietary tannins to improve rumen metabolism and ruminant nutrition. J Sci Food Agric. 91(1):24–37.
- Patra AK, Aschenbach JR. 2018. Ureases in the gastrointestinal tracts of ruminant and monogastric animals and their implication in urea-N/ammonia metabolism: a review. J Adv Res. 13:39–50.
- Pope PB, Smith W, Denman SE, Tringe SG, Barry K, Hugenholtz P, McSweeney CS, McHardy AC, Morrison M. 2011. Isolation of Succinivibrionaceae implicated in low methane emissions from Tammar wallabies. Science. 333(6042):646–648.
- Pulina G, Nudda A, Macciotta NPP, Battacone G, Rassu SPG, Cannas A. 2007. Non-nutritional factors affecting lactation persistency in dairy ewes: a review. Ital J Anim Sci. 6(2):115–141.
- Reed BM, Tanprasert P. 1995. Detection and control of bacterial contaminants of plant tissue cultures. A review of recent literature. Plant Tissue Cult Biotechnol. 1:137–142.
- Shannon CE, Weaver W. 2001. The mathematical theory of communication. ACM SIGMOBILE Mob Comput Commun Rev. 5(1):3–55.
- Simpson EH. 1949. Measurement of diversity. Nature. 163(4148):688–688.
- Smeriglio A, Barreca D, Bellocco E, Trombetta D. 2017. Proanthocyanidins and hydrolysable tannins: occurrence, dietary intake and pharmacological effects. Br J Pharmacol. 174(11):1244–1262.
- Spanghero M, Salem AZM, Robinson PH. 2009. Chemical composition, including secondary metabolites, and rumen fermentability of seeds and pulp of Californian (USA) and Italian grape pomaces. Anim Feed Sci Technol. 152(3–4):243–255.
- Tedeschi LO, Cannas A, Fox DG. 2010. A nutrition mathematical model to account for dietary supply and requirements of energy and other nutrients for domesticated small ruminants: the development and evaluation of the small ruminant nutrition system. Small Rumin Res. 89(2–3):174–184.
- Theodoridou K, Aufrère J, Andueza D, Pourrat J, Le Morvan A, Stringano E, Mueller-Harvey I, Baumont R. 2010. Effects of condensed tannins in fresh sainfoin (Onobrychisviciifolia) on in vivo and in situ digestion in sheep. Anim Feed Sci Technol. 160(1–2):23–38.
- Vasta V, Daghio M, Cappucci A, Buccioni A, Serra A, Viti C, Mele M. 2019. Invited review: plant polyphenols and rumen microbiota responsible for fatty acid biohydrogenation, fiber digestion, and methane emission: experimental evidence and methodological approaches. J Dairy Sci. 102(5):3781–3804.
- Yuangklang C, Vauspen K, Wongsuthavas S, Bureenok S, Panyakaew P, Alhairdary A, Mohamed HE, Beynen AC. 2010. Effect of replacement of soybean meal by dried tomato pomace on rumen fermentation and nitrogen metabolism in beef cattle. Am J Agric Biol Sci. 5:256–260.