Abstract
Acute heat stress (AHS) is known to affect rabbit production and well-being negatively. Yet, early acute heat stress effect on later physiological response is poorly studied. Our study aimed to provide more knowledge of rabbit physiological response to early acute heat stress in two distinguished rabbit breeds. A total of 120 males of New Zealand White (NZW) and Baladi Black (BB) rabbits five weeks old were recruited and randomly divided into two sub-groups, control groups (Control) reared in a controlled environmental chamber 28 °C, and 40% RH and early acute heat stress groups (HS) exposed to 36 °C and 62% RH for 6 hours. At 13 weeks, rabbits of all groups were subjected to the same AHS conditions. Our results, irrespective of breeding differences, demonstrate a significant (p < .05) increase in inflammation markers (TNFα, IL-6 and lipid peroxidation), acute-phase proteins (ceruloplasmin, α-1-acid glycoprotein), and DNA fragmentation with a significant reduction in liver antioxidant enzymes concentrations (glutathione reduced, superoxide dismutase, and catalase) in HS groups compared to breed relative control groups. Furthermore, when all experimental groups at 13 weeks of age were subjected to the same stress conditions, the early HS group showed the same significant adverse physiological effects. It can be suggested that rabbits from different genetic groups are vulnerable to acute heat stress exposure at the early stage of life compared to later stages.
Heat stress is one of the most critical issues facing rabbit production.
Exposure young rabbits to acute heat stress had adverse effects on physiological parameters in later life.
Rabbits exposed to acute heat stress at an early age did not produce thermo-tolerance ability to later time heat exposure.
Highlights
Introduction
Heat stress is a critical issue facing rabbit production in tropical and semi-tropical countries. Rabbits exposed to chronic or cyclic heat stress were associated with a reduction in feed intake, a decline in growth rate (Liu et al. Citation2011), suppress humoural and cell-mediated immune responses (Hassan et al. Citation2011; Ferrian et al. Citation2012). Meanwhile, acute heat stress, a sudden elevation in ambient temperatures for some time, induces oxidative stress by the excessive production of reactive oxygen species (ROS) (Yang et al. Citation2010; Akbarian et al. Citation2016a). ROS's excessive production is a critical mediator in heat stress-induced apoptosis (Gu et al. Citation2014). On the other hand, heat stress exposure to different rabbit breeds (Botucatu vs. Botucatu × NZW) showed relevant differences in physiological responses and growth performance in rabbits (Zeferino et al. Citation2011) as well as in poultry (commercial vs. native chickens (Duangjinda et al. Citation2017).
When animals experience thermal stress, the cell underlines oxidative stress using endogenous defense mechanisms to protect itself from the generated ROS damage. The excessive production of ROS activates cellular apoptosis and necroptosis (Redza-Dutordoir and Averill-Bates Citation2016). Antioxidant enzymes play a crucial role in protecting cells from oxidative stress and keeping cells from damage (Birben et al. Citation2012). In rabbits, heat stress exposure was reported to be associated with a reduction in total antioxidant capacity (TAC), superoxide dismutase (SOD), glutathione peroxidase as well as increasing malondialdehyde (MDA) concentrations in rabbit plasma and liver (Liu et al. Citation2011). At the onset of stress, a cascade of events was reported to bring back homeostasis. The release of inflammatory cytokines (e.g., Tumour necrosis factor-alpha and interleukin 1 & 6) leads to increased production of acute phase proteins (APPs) by the liver to reduce inflammation and repair damaged cells (Cray Citation2013). In rabbits, the changes in plasma APPs indicates an increase in systemic inflammation (Green Citation2015). The comet test (single-cell gel electrophoresis) was proposed to be usefully applied as a biomarker for ecological studies and clinical studies (Costa and Teixeira Citation2014).
Early thermal conditioning regimes, elevating the ambient temperature for a limited time of chicks at an early age, have been used in poultry to alleviate the heat stress adverse effects on later exposure to high environmental temperature conditions inducing thermo-tolerance ability (Nassar and Elsherif Citation2018; Ruzic et al. Citation2020). Our knowledge of rabbit physiological response to acute heat stress is based on limited data. Thus, we undertook this study to clarify the effect of early acute heat stress on the biological and physiological behaviour in two genetic groups (New Zealand White rabbit (NZW) and Baladi Black (BB)) on later heat exposure along the production period.
Materials and methods
Experimental design
New Zealand white (NZW) and Baladi Black (BB) Baladi Black (BB) rabbits, Baladi Black are considered as a local cross breed that resulted from crossing between heavy Baladi does and pure Giant Flander bucks for several generations, with the selection criteria of heavy body weight and pure black colour traits of the resulted offspring. Thus, Baladi Black rabbits are characterised by their high tolerance to climatic stress (Khalil Citation1999), were used to investigate the effect of early acute heat stress at five weeks of age on the physiological and immune response in later life. A total of 120 male rabbits four weeks old from the two breeds, 60 males from each breed were obtained from a commercial farm. Rabbits were reared under normal conditions (28 ± 2 °C and 40 ± 2% relative humidity) for one-week until 35 days of age to adapt to the new conditions and reduce transportation stress. At 35 days of age, both breeds' rabbits were equally divided into two groups; control groups (NZWC and BBC) and heat exposed (HS) groups at 36 ± 1 °C and 62% relative humidity for 6 hours (NZWT and BBT) only 6 hours in a single day. Six rabbits from each group with each breed were randomly taken, slaughtered. Liver samples were removed, immediately dissected, snap-frozen in liquid nitrogen stored at −80 °C until further studies. The remaining rabbits from all groups were kept up to 13 weeks in an automatically controlled environmental chamber at 28 ± 1 °C and 40% relative humidity. At 13 weeks of age, all groups, Control exposed (single exposure) (NWZC2, BBC2), and early acute heat stress exposure groups (Double exposure) (NWZT2 and BBT2) were subjected to acute heat stress at 36 ± 1 °C for six hours. Then another six rabbits were randomly taken, slaughtered. Then liver samples were taken as previously mentioned at seven weeks of age for other traits. The rabbits were offered feed with a commercial diet for ad libitum consumption.
Tissue preparation
Liver samples were washed with saline solution (0.9% NaCl), then the samples were homogenised on ice using phosphate-buffered saline (PBS; pH 7.4) in a 1:10 ratio (w:v). The homogenates were centrifuged at 10,000×g for 15 min at 4 °C. The resulting supernatants were collected and stored at −80 °C until further processing
Pro-inflammatory cytokines and APPs
Tumour necrosis factor-α (TNF-α) was determined using a specialised rabbit quantitative sandwich ELISA kit (CUSABIO, Houston, TX, USA, Cata# CSB-E06998Rb) with a detection range of 78–5000 pg/mL and sensitivity of 19.5 pg/mL.
The rabbit ceruloplasmin (Cp) was determined in rabbit liver using a specialised sandwich ELISA kit manufactured in an ISO 9001:2015 Certified Laboratory (MyBioSource, Southern California, San Diego, USA, Cata# MBS2601217) with a detection range of 3.12–200 ng/ml and sensitivity of 0.6 ng/mL. α1-Acid glycoprotein (AGP) was measured using a quantitative competitive ELISA Kit (MyBioSource, Southern California, San Diego, USA, Cata# MBS726495) for rabbit with a sensitivity of 1.0 pg/mL. Caspase 3 was measured using a specialised rabbit ELISA kit (CUSABIO, Houston, TX, USA, Cata# CSB-E15965Rb) with a detection range of 0.312–20 ng/mL and sensitivity of 0.078 ng/mL.
Antioxidant enzymes activity and status
Hepatic levels of MDA, catalase (CAT), and glutathione reduced (GSH) were determined using colorimetric End-point methods using specialised kits (BioDiagnositc, Giza, Egypt). Superoxide dismutase (SOD) was determined using the kinetic method using specialised kits (BioDiagnositc, Giza, Egypt).
Single cell gel electrophoresis (comet test)
Comet assay protocol was performed based on Singh et al. (Citation1988) suggested methodology. In brief, rabbit liver samples were homogenised in homogenisation buffer (50 mmol/L cold sodium phosphate buffer (pH 7.0) supplied with 0.1 mmol/L EDTA) in a 1:9 ratio (w:v). Then, the homogenates were centrifuged at 1000 xg for ten min at 4 °C, and the pellet was resuspended again in an ice-chilled homogenising buffer. The suspension was collected, and 250 µL of it was mixed with low-melting agarose (0.65%). The mixture was carefully spread evenly upon fully frosted microscope slides coated with 0.75% normal agarose, which is diluted in phosphate buffer saline (Ca2 + and Mg2+ free). Finally, the slide was covered with a layer of low-melting agarose (0.65%). Slides were placed in lysate solution (1% Triton X-100, 10% DMSO and 89% of 10 mmol/L Tris, 1% sodium lauryl sarcosine, 2.5 mol/L NaCl, 100 mmol/L Na2EDTA, pH 10) at 4 °C and kept for 2 h. Then, slides were pre-incubated for 15 min in cold electrophoresis buffer (300 mmol/L NaOH and 1 mmol/L Na2EDTA, pH 12) and exposed to 25 V/300 mA for 20 min within an ice-bath. Slides were treated with 0.4 M Tris, pH 7.5 for 5 min, and DNA was stained by ethidium bromide (20 mg/mL) for 5 min, then were washed in ddH2O. All steps were done under dimmed light to avoid additional DNA damage. The slide was examined using a Leitz Ortho planepi fluorescence microscope (magnification 2009) supplied with a 515–560 nm excitation filter and 590 nm barrier filter. The microscope was connected with a camera to a computer-based image analysis system (Comet Assay IV software, Perspective Instruments). In each slide, 100 nuclei were randomly selected, and the DNA damage was estimated using the ratio of tail DNA content/the whole cellular DNA content.
Statistical analysis
All data were subjected to analysis of variance using SAS's GLM procedure (SAS Institute Inc., Cary, NC). Data were analysed using heat stress and breed as fixed effects and their interactions. Duncan multiple range tests made comparisons among means; significance was considered as p < .05.
Results
Hepatic inflammation cytokines
Hepatic inflammatory cytokines, TNF-α and IL-6, were measured after acute heat stress exposure to clarify the effect of heat stress on inflammation induction (Table ). Hepatic TNF-α and IL-6 were increased significantly (p < .05) in response to acute HS exposure at five weeks of age by 26 and 42%, respectively, in NZWT and 32 and 40%, respectively, in BBT compared to their relative Control groups NZWC and BBC respectively. Furthermore, when all experimental groups exposed to AHS at week 13 of age, a significant (p < .05) increase in hepatic TNF-α and IL-6 levels was also observed (32 and 42% in NZWT2) and 34 and 40% in BBT2, respectively) in early HS groups compared to their relative Control exposed groups (NZWC2 and BBC2).
Table 1. Hepatic inflammatory cytokines levels and acute phase protein concentration for New Zealand White and Baladi Black rabbits are exposed to early acute heat stress at weeks 5 and 13 of age.
Hepatic acute phase proteins and apoptotic factor
Hepatic acute phase proteins (APPs) levels were used to identify the intensity of acute phase reaction caused by acute heat stress exposure (Table ). Synthesis of ceruloplasmin (Cp) and α-1-acid glycoprotein (AGP) increased significantly (p < 0.05) in HS groups at week 5 compared to control groups. The fold change in AGP in the early heat exposed (NZWT and NZWT2) group was 3.9- and 3.8-fold increase at week fifth and week 13, respectively, compared to its Control group and Control exposed (NZWC and NZWC2). BBT and BBT2 also showed a higher AGP level with 4.2- and 3.5-folds increase at week fifth and week 13, respectively, compared to its Control group. Meanwhile, Cp showed a significant (p < 0.05) moderate increase, 1.6- and 1.2-fold, in the heat-stressed NZW group at weeks 5 (NZWT) and 13 (NZWT2) of age, respectively, compared to its relative Control group (NZWC and NZWC2). In the fifth week, the level of Cp increased significantly (p < .05) by 1.4-fold in the BBT group compared to its relative Control (BBC). Nevertheless, the level of Cp did not differ between (BBC2) and early heat-stressed (BBT2) groups when subjected to the same thermal condition at week 13.
Furthermore, the hepatic caspases-3 (casp-3) was determined to investigate its role in rabbit response to acute heat stress (Table ). At week 5, AHS induced a significant (p < .05) increase in casp-3 levels in NZWT and BBT rabbits by 51 and 50%, respectively, compared to their relative Control groups (NZWC and BBC). Furthermore, at week 13, casp-3 levels increased significantly (p < 0.05) in NZWT2 and BBT2 groups by 23 and 42%, respectively, compared to their relative Control exposed groups (NZWC2 and BBC2).
Hepatic antioxidant status
Hepatic MDA levels and antioxidant enzymes activity and for NZW and BB rabbits exposed to acute HS at weeks 5 and 13 of age are presented in Table . At week 5, an exponential increase (p < 0.05) in MDA was observed in NZWT and BBT rabbits groups compared to its relative Control groups (NZWC and BBC), reaching 2.5- and 3.8-fold, respectively. Furthermore, at week 13, when all the experimental groups exposed to the same acute HS, MDA levels showed a higher level in early acute heat stress NZWT2 and BBT2 groups reaching 4.6- and 2.3-fold increase, respectively, compared to their relative control exposed groups (NZWC2 and BBC2).
Table 2. Hepatic lipid peroxidation and antioxidant enzymes levels for New Zealand White and Baladi Black rabbit exposed to acute heat stress at weeks 5 and 13 of age.
Regardless to breed, all the studied hepatic antioxidant enzymes showed a significant (p < .05) decrease in activity with early acute HS exposure at week five and week 13. The intensity of activity reduction at week 5 ranged from high for GSH (41 and 74%), moderate for catalase (34 and 30%) and low for SOD (25 and 21%) in NZWT and BBT, respectively compared to its relative Control groups (NZWC and BBC). At week 13 of age, when all experimental groups subjected to acute heat stress, the early HS groups (NZWT2 and BBT2) showed significant (p < .05) reduction in the activity of GSH (67 and 32%), catalase (41 and 38%) and SOD (37 and 47%) in NZWT2 and BBT2, respectively, compared to its relative Control exposed groups (NZWC2 and BBC2).
DNA fragmentation
According to Comet assay results (Table & Figure ), early acute HS at the fifth week of age-induced significant (p < .05) DNA damage. The acute HS exposure at week 5 of age increased tailed DNA % and tail moment by 3.7- and 1.8-fold in NZWT and by 2.25- and 2-fold in BBT, respectively, compared to their relative Control groups (NZWC and BBC).
Figure 1. Evaluation of DNA damage in the rabbit's hepatic cells using the comet assay. The photos show the electrophoretic DNA pattern as tailed (T) and untailed (UT) DNA. (A) New Zealand white control at five-week, NZWC (B) NZWT New Zealand white exposed to early acute heat-stress at five-week, (C) Baladi Black control at five-week BBC, (D) BBT Baladi Black exposed to early acute heat-stress at five-week, (E) NZWC2 New Zealand white control exposed to heat stress at 13 weeks (single exposure), (F) NZWT2 New Zealand white exposed for the second time to heat stress at 13 weeks (Double exposure), (G) BBC2 Baladi Black control exposed to heat stress at 13 weeks(single exposure) and (H) BBT2 Baladi Black exposed for the second time to heat stress at 13 weeks (Double exposure). At week 5 of age, only heat stress exposed groups were subjected to acute heat stress (36 °C 62% RH for 6h). At week 13 of age, all the experimental rabbit groups were subjected to acute heat stress (36 °C 62% RH for 6h).
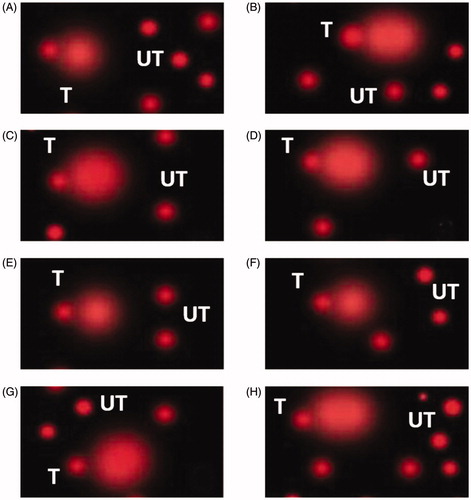
Table 3. DNA damage measured using Comet assay for New Zealand White and Baladi Black rabbit exposed to acute heat stress at weeks 5 and 13 of age.
Moreover, at 13 weeks of age, the same trend was observed in early HS-exposed groups (NZWT2 and BBT2) with increasing tailed DNA % and tail moment by 2.2- and 1.9-fold increase in NZWT2 and 1.3- and 1.7-fold increase in BBT2, respectively, compared to their relative control exposed groups (NZWC2 and BBC2). However, the intensity of DNA damage was lower (p < .05) in the local BB compared to NZW rabbits when subjected to the same thermal stress.
Discussion
Heat stress is an environmental stressor that stimulates excessive ROS production, which induces oxidative stress (Slimen et al. Citation2014; Akbarian et al. Citation2016b). The early acute heat stress exposure in the current study induced inflammatory response and increased the production levels of pro-inflammatory, TNF-α and IL-6, cytokines. A positive relationship between oxidative stress and inflammation was reviewed (Biswas Citation2016; Chatterjee Citation2016). Biswas (Citation2016) stated that IL-6 produces ROS through increased expression of the oxidative stress mediator NADPH oxidase 4 (NOX4), whereas the NOX4 overexpression has also been found to stimulate IL-6 release. Acute heat stress was reported to activate inflammatory signalling in porcine skeletal muscles (Ganesan et al. Citation2017). It has been reported that the increasing levels of TNF-α and IL-1 induced the release of IL-6 from immune cells (i.e. macrophage, lymphocytes, and fibroblasts) during heatstroke, which indicate progressive inflammation response (Hashim Citation2010). In mice, heat stress-induced TNF-α, and IL-6 release subsequently caused intestinal tissue injury (Liu et al. Citation2011).
APPs were presented as appropriate non-specific markers and biological effects reactants to assess and monitor animal health and its relatively long time before cleaning from circulation compared to cytokines (Gruys et al. Citation2005). Animals subjected to environmental disturbance events caused unbalanced homeostasis to exert acute phase response, which is a spontaneous reaction mediated by APPs synthesis from hepatocytes (Khalil and Al-Humadi Citation2020). A significant increase in Cp and AGP was observed in HS groups (Table ). The increasing levels of APPs were reported to be associated with systemic inflammation and, consequently, clinical adverse events (Green Citation2015). Sobotta et al. (Citation2017) identified IL-6 as the central mediator of the instant induction of hepatic APPs release during infection or after injury. The currently studied APPs are positive acute phase reactants meaning that they increase with stress exposure, which can explain the significant elevation in the production of these proteins due to AHS exposure (Jain et al. Citation2011). Since the pattern of APPs is yet to be understood and may be species-dependent (Jain et al. Citation2011), thus, we can conclude from our results that AGP can be used as a moderated stress indicator for rabbit species.
HS exposed groups showed significantly high MDA levels (Table ). The induction of ROS production can explain the increasing level of MDA in response to acute heat stress exposure (Yang et al. Citation2010). There was an observed negative correlation between heat stress exposure and antioxidant enzymes activity in the present study. Our finding was inconsistent with the previously reported negative effect of heat stress exposure on antioxidant enzyme activity (Heise et al. Citation2006; Wang et al. Citation2014). The recruitment of cellular antioxidants can justify the reduction in antioxidant enzymes to scavenge free radicals generated by heat exposure causing its depletion (Belhadj Slimen et al. Citation2016). (Zeng et al. Citation2014) reported a significant reduction in liver antioxidant enzymes activity (catalase and SOD) and total antioxidant capacity in Pekin ducks subjected to acute heat stress for one hour.
Meanwhile, they reported higher antioxidant enzymes activity in Muscovy ducks subjected to the same acute heat stress condition. Furthermore, our results contrast to earlier findings reviewed by (Hu et al. Citation2019), who reported an increase in poultry antioxidant enzyme activity subjected to acute heat stress. These controversial results can be explained by the difference in thermo-tolerance response due to heat stress regime conditions (e.g., intensity and duration) in addition to species and tissue differences (Akbarian et al. Citation2016a,Citationb). The differences in antioxidant enzymes concentrations between the two breeds when subjected to the same heat stress conditions fluctuated and had no common trend.
Furthermore, the catalase levels in both breeds did not differ in the control groups at fifth or 13 weeks of age. It is interesting to note that MDA levels were significantly higher in BB compared with NZW rabbits subjected to the same heat condition at all time of the experiment. Under heat stress, rabbits with different genetic backgrounds showed relevant differences in the physiological responses (Zeferino et al. Citation2011).
The present results suggest that HS exposure induced higher apoptotic activity in the rabbit's liver cells. Further, early HS exposure increases the odds of hepatic cell apoptosis occurrence compared to later heat stress exposure. Rabbit exposed to thermal stress was reported to show moderate to severe degenerative and necrotic alterations of liver tissues (Abdelnour et al. Citation2020). Acute heat stress was suggested to accelerate apoptosis by decreasing protein translation and increasing protein degradation with ROS production (Wang et al. Citation2014). The mechanisms of acute heat stress underline cell death depend on stress intensity (temperature and duration) and cell type (Velichko et al. Citation2013). The biological effect of acute heat stress was suggested to be mediated by activating the mitochondrial pathway and activation of Casp-9 and Casp-3 that induce cell apoptosis (Gu et al. Citation2014). Casp-3 is presented as the executioner of cell apoptosis and responsible for DNA fragmentation (Porter and Jänicke Citation1999; Stoyanovsky and Billiar Citation2007). D'Mello et al. (Citation2000) reviled that casp-3 is responsible for events associated with apoptotic death, such as chromatin condensation and DNA fragmentation. Brentnall et al. (Citation2013) stated that casp-3 is the primary executioner of apoptotic death and that the effector caspases (casp-3 and casp-7) are responsible for initiating the distinguished degradation phase of apoptosis, including DNA fragmentation. Moreover, Mehaisen et al. (Citation2016) concluded that the significant increase in casp-3 gene expression was responsible for increasing DNA damage in brain and liver tissues of laying hen. Heat stress is reported to not only induce DNA damage but also inhibits the DNA repair system (Velichko et al. Citation2013).
Conclusions
The present study indicated that rabbits exposed to acute heat stress at an early age did not produce thermo-tolerance ability to later time heat exposure. Results also showed that there are breed differences in the concentration of the inflammatory cytokines, APPs, and antioxidant enzymes concentration; nevertheless, it follows the same physiological changes trend for heat stress exposure. Further investigations are needed to clarify the biological and physiological responses of rabbits subjected to acute heat stress at different life stages to set mitigation strategies to overcome heat stress.
Animal welfare statement
The authors confirm that they have followed EU standards for the protection of animals used for scientific purposes.
Ethical approval
The experimental design and all the research protocols were approved by the Medical Research Ethics Committee (MREC) of the National Research Centre with ethical approval code 20/128.
Acknowledgments
This paper is based upon work supported by the National Research Center (#12050406).
Disclosure statement
The authors reported no potential conflict of interest.
Additional information
Funding
References
- Abdelnour SA, El-Saadony MT, Saghir SAM, Abd El-Hack ME, Al-Shargi OYA, Al-Gabri N, Salama A. 2020. Mitigating negative impacts of heat stress in growing rabbits via dietary prodigiosin supplementation. Livestock Sci. 240:104220.
- Akbarian A, Michiels J, Degroote J, Majdeddin M, Golian A, Smet SD. 2016a. Association between heat stress and oxidative stress in poultry; mitochondrial dysfunction and dietary interventions with phytochemicals. J Anim Sci Biotechnol. 7:37–37.
- Akbarian A, Michiels J, Degroote J, Majdeddin M, Golian A, Smet SD. 2016b. Association between heat stress and oxidative stress in poultry; mitochondrial dysfunction and dietary interventions with phytochemicals. J Animal Sci Biotechnol. 7(1): 37.
- Belhadj Slimen I, Najar T, Ghram A, Abdrrabba M. 2016. Heat stress effects on livestock: molecular, cellular and metabolic aspects, a review. J Anim Physiol Anim Nutr (Berl)). 100(3):401–412.
- Birben E, Murat Sahiner U, Sackesen C, Erzurum S, Kalayci O. 2012. Oxidative stress and antioxidant defense. World Allergy Organ J. 5(1):9–19.
- Biswas SK. 2016. Does the interdependence between oxidative stress and inflammation explain the antioxidant paradox? Oxid Med Cell Longev. 2016:5698931
- Brentnall M, Rodriguez-Menocal L, De Guevara RL, Cepero E, Boise LH. 2013. Caspase-9, caspase-3 and caspase-7 have distinct roles during intrinsic apoptosis. BMC Cell Biol. 14:32.
- Chatterjee S. 2016. Chapter Two - Oxidative Stress, Inflammation, and Disease. In: Thomas Dziubla and D. Allan Butterfield (eds.), Oxidative Stress and Biomaterials. USA: Academic Press.
- Costa S, and Teixeira JP. 2014. Comet Assay. In Philip Wexler (ed.), Encyclopedia of Toxicology 3rd Ed. Oxford: Academic Press.
- Cray C. 2013. Biomarkers of inflammation in exotic pets. J Exotic Pet Med. 22(3):245–250.
- D'Mello SR, Kuan CY, Flavell RA, Rakic P. 2000. Caspase-3 is required for apoptosis-associated DNA fragmentation but not for cell death in neurons deprived of potassium. J Neurosci Res. 59:24–31.
- Duangjinda M, Tunim S, Duangdaen C, Boonkum W. 2017. Hsp70 genotypes and heat tolerance of commercial and native chickens reared in hot and humid conditions. Rev Bras Cienc Avic. 19(1):7–17.
- Ferrian S, Guerrero I, Blas E, Garcia-Diego FJ, Viana D, Pascual JJ, Corpa JM. 2012. How selection for reproduction or foundation for longevity could have affected blood lymphocyte populations of rabbit does under conventional and heat stress conditions. Vet Immunol Immunopathol. 150(1-2):53–60.
- Ganesan S, Volodina O, Pearce SC, Gabler NK, Baumgard LH, Rhoads RP, Selsby JT. 2017. Acute heat stress activated inflammatory signaling in porcine oxidative skeletal muscle. Physiol Rep. 5(16):e13397.
- Green MD. 2015. Acute phase responses to novel, investigational vaccines in toxicology Studies: The relationship between C-reactive protein and other acute phase proteins. Int J Toxicol. 34(5):379–383.
- Gruys E, Toussaint MJ, Niewold TA, Koopmans SJ. 2005. Acute phase reaction and acute phase proteins. J Zhejiang Univ Sci B. 6(11):1045–1056.
- Gu ZT, Wang H, Li L, Liu YS, Deng XB, Huo SF, Yuan FF, Liu ZF, Tong HS, Su L. 2014. Heat stress induces apoptosis through transcription-independent p53-mediated mitochondrial pathways in human umbilical vein endothelial cell. Sci Rep. 4:4469–4469.
- Hashim IA. 2010. Clinical biochemistry of hyperthermia. Ann Clin Biochem. 47(Pt 6):516–523.
- Hassan RA, Ebeid TA, El-Lateif AIA, Ismail NB. 2011. Effect of dietary betaine supplementation on growth, carcass and immunity of New Zealand White rabbits under high ambient temperature. Livestock Sci. 135(2-3):103–109.
- Heise K, Puntarulo S, Nikinmaa M, Abele D, Pörtner H-O. 2006. Oxidative stress during stressful heat exposure and recovery in the North Sea eelpout < em > Zoarces viviparus</em > L. J Exp Biol. 209:353.
- Hu R, He Y, Arowolo MA, Wu S, He J. 2019. Polyphenols as Potential Attenuators of Heat Stress in Poultry Production. Antioxidants (Basel, Switzerland). 8:67.
- Jain S, Gautam V, Naseem S. 2011. Acute-phase proteins: As diagnostic tool. J Pharm Bioallied Sci. 3(1):118–127.
- Khalil MH. 1999. Rabbit genetic resources of Egypt. Anim Genet Resour Inf. 26:95–111.
- Khalil RH, Al-Humadi N. 2020. Types of acute phase reactants and their importance in vaccination (Review). Biomed Rep. 12(4):143–152.
- Liu HW, Dong XF, Tong JM, Zhang Q. 2011. A comparative study of growth performance and antioxidant status of rabbits when fed with or without chestnut tannins under high ambient temperature. Anim Feed Sci Technol. 164(1-2):89–95.
- Liu Z, Sun X, Tang J, Tang Y, Tong H, Wen Q, Liu Y, Su L. 2011. Intestinal inflammation and tissue injury in response to heat stress and cooling treatment in mice. Mol Med Rep. 4(3):437–443.
- Mehaisen GM, Eshak MG, El Sabry MI, Abass AO. 2016. Expression of inflammatory and cell death program genes and comet DNA damage assay induced by Escherichia coli in layer hens. PLoS One. 11(6):e0158314.
- Nassar FS, Elsherif HR. 2018. Increasing heat tolerance in broiler by using thermal manipulation during embryonic development. Approaches Poultry, Dairy Vet Sci. 5:447–449.
- Porter AG, Jänicke RU. 1999. Emerging roles of caspase-3 in apoptosis. Cell Death Differ. 6(2):99–104.
- Redza-Dutordoir M, Averill-Bates DA. 2016. Activation of apoptosis signalling pathways by reactive oxygen species. Biochimica et Biophysica Acta (BBA) - Molecular Cell Res. 1863(12):2977–2992.
- Ruzic Z, Kanacki Z, Jokanovic M, Vidakovic S, Knezevic S, Jovic S, Paras S. 2020. The influence of vitamin C and early-age thermal conditioning on the quality of meat and specific production characteristics of broilers during heat stress. Turk J Vet Anim Sci. 44:314–322.
- Singh NP, McCoy MT, Tice RR, Schneider EL. 1988. A simple techniquefor quantitation of low levels of DNA damage in individual cells. Exp Cell Res 175:184–191.
- Slimen IB, Najar T, Ghram A, Dabbebi H, Ben Mrad M, Abdrabbah M. 2014. Reactive oxygen species, heat stress and oxidative-induced mitochondrial damage. A review. Int J Hyperthermia. 30:513–523.
- Sobotta SA, Raue X, Huang J, Vanlier A, Jünger S, Bohl U, Albrecht MJ, Hahnel Stephanie Wolf NS, Mueller LA, D'Alessandro , et al. 2017. Model Based Targeting of IL-6-Induced Inflammatory Responses in Cultured Primary Hepatocytes to Improve Application of the JAK Inhibitor Ruxolitinib. Front Physiol. 8:775.
- Stoyanovsky DA, Billiar TR. 2007. CHAPTER 12 - Cellular non-heme iron modulates apoptosis and caspase 3 activity. ' In: Ernst Van Faassen and Anatoly Fyodorovich Vanin (eds.), Radicals for Life. Elsevier: Amsterdam.
- Velichko AK, Markova EN, Petrova NV, Razin SV, Kantidze OL. 2013. Mechanisms of heat shock response in mammals. Cell Mol Life Sci. 70(22):4229–4241.
- Wang SH, Cheng CY, Chen CJ, Chen HH, Tang PC, Chen CF, Lee YP, Huang SY. 2014. Changes in protein expression in testes of L2 strain Taiwan country chickens in response to acute heat stress. Theriogenology. 82(1):80–94.
- Yang L, Tan GY, Fu YQ, Feng JH, Zhang MH. 2010. Effects of acute heat stress and subsequent stress removal on function of hepatic mitochondrial respiration, ROS production and lipid peroxidation in broiler chickens. Comp Biochem Physiol C Toxicol Pharmacol. 151(2):204–208.
- Zeferino CP, Moura ASAMT, Fernandes S, Kanayama JS, Scapinello C, Sartori JR. 2011. Genetic group x ambient temperature interaction effects on physiological responses and growth performance of rabbits. Livestock Sci. 140(1-3):177–183.
- Zeng T, Li J-j, Wang D-q, Li G-q, Wang G-l, Lu L-z. 2014. Effects of heat stress on antioxidant defense system, inflammatory injury, and heat shock proteins of Muscovy and Pekin ducks: evidence for differential thermal sensitivities. Cell Stress Chaperones. 19(6):895–901.