Abstract
Soybean agglutinin (SBA) represents the anti-nutritional molecule in soybean. This molecule agglutinates erythrocytes of several animal species. The specific binding of SBA to glycoprotein of the cellular plasma membrane is necessary for SBA to exert its toxic action. The sensitivity of erythrocyte to SBA for various species is different, however, the pathway is unclear. We aimed to optimise the method of extracting erythrocyte membrane (EM) and to analyse the pathway of the different sensitivity of erythrocyte to SBA in three animals species (rabbit as a rodent, pig as an omnivore, and bovine as a ruminant). The method of low osmotic haemolysis was used to extract the EM. To precipitate the EM, we evaluated the purification effects of three lysis bufferes, including NP-40, RIPA (medium) and RIPA (weak). All SBA-specific binding proteins (SSBP) on erythrocyte membranes were conducted using co-immunoprecipitation (CO-IP) and analysed using liquid chromatography coupled to tandem mass spectrometry (LC-MS-MS (Q-E)). The results showed that NP-40 lysis buffer was the most effective than the other two kinds of lysates to extract EM proteins. Functionally, the SSBP of EM could be divided into three categories in each animal, including cytoskeleton proteins, catalytic enzyme proteins and the functional regulatory proteins. The cytoskeleton proteins were the main different expressed proteins among the EM proteins in pig, rabbit than that in bovine, which may be one important reason for the difference of the SBA sensitivity. This research provides basis to reveal the pathway of SBA-induced hemagglutination differences in erythrocytes in different animal species.
We optimised the method of extracting EM to isolate and identify SSBP in different animal species.
NP-40 lysis buffer was the most effective than the other two kinds of lysates to extract EM proteins.
Cytoskeleton proteins were the main different expressed proteins among the EM proteins in pig, rabbit, and cow.
Highlights
Introduction
Soybean agglutinin (SBA) is a kind of glycoprotein with a specific binding to N-acetyl-d-galactosamine/d-galactose. Such glycoprotein possesses a molecular weight of 120 kDa. SBA represents a main anti-nutritional factor in soybean (Lotan et al. Citation1974). This anti-nutritional factor plays a unique biological function as a bioactive protein through direct or indirect contact with human blood cells (Pereira et al. Citation1974). The biological functions of SBA can be shown in two ways. First, anti-nutritional functions that appear in animal digestion and absorption of nutrients and influencing multiple biological functions of cells (Pan et al. Citation2013; Halder et al. Citation2016). The other way is the specificity of sugar binding. Therefore, the specific binding of SBA to glycoprotein is a necessary condition for SBA to show its toxic action.
The understanding of SBA has begun with the discovery of its agglutination activity. SBA can agglutinate the erythrocyte (red blood cells, RBCs), therefore, it was initially called haemagglutinin (Goldstein et al. Citation1980; Tremblay et al. Citation2011). Lotan et al. (Citation1973) found that SBA possesses species-specific coagulation activity and sensitive to the agglutination of rabbit and human erythrocytes. In many researches, SBA showed that the rabbit blood was the most sensitive convenient among the animal species for this coagulation activity (Tateno et al. Citation1998; Kakiuchi et al. Citation2002). Liu (Citation2006) also confirmed that the rabbit is the most sensitive species to SBA agglutination, while pig had less responsive than rabbits, and ruminant erythrocytes can be only agglutinated at a specific concentration, for example the weak agglutinin in sheep erythrocytes only occurred when SBA was diluted between 8 and 64 times. Hughes-Jones et al. (Citation1963) indicated that the immune agglutination of erythrocyte comes from a dynamic process which can be divided into two stages. The first immune agglutination stage starts immediately following the mixing antibody molecules with antigens on the surface of the cells. The second stage begins directly after the first stage and results from fruitful collisions occurring between erythrocyte so that antibody molecules bound to one erythrocyte to another one. Guesdon et al. (Citation1979) proved that SBA can be polymerised due to chemical or physical factors, the monomers can cross-linked with each other, and the sites of reaction with erythrocyte also can be increased, and consequently the agglutination activity of erythrocyte enhances.
Erythrocyte are the main components of the blood circulation system in the body. The main functions of erythrocyte are carrying oxygen, transporting carbon dioxide and maintaining electrolytes balance (Tomova et al. Citation2008). The erythrocyte membrane (EM) components are complex compound, including bilayer of phospholipid, membrane protein and membrane skeleton (Tse and Lux Citation1999; Gordon-Smith Citation2009). The membrane protein of erythrocyte mainly includes peripheral protein and integrin protein, that represents the basics for the functions of the erythrocytes. Because the structure and function of EM are overlapped, the normal structure and function are directly related to the normal physiological activities of erythrocyte.
The sensitivity of erythrocytes from different animal species to SBA is different (Lotan et al. Citation1973; Tateno et al. Citation1998; Kakiuchi et al. Citation2002; Liu Citation2006). However, no clear reasons indicating why SBA causes the difference of haemagglutinin activity in different animal species. Therefore, this study aims to optimise the method of extracting erythrocyte membrane (EM), and to analyse the pathway of the different sensitivity of different animals’ erythrocyte (rodents, rabbit; omnivore, pig; and ruminant, bovine) to SBA. This research can provide a major basis to reveal the pathway of SBA-induced hemagglutination differences in erythrocytes among different animal species.
Materials and methods
Animal management
To conform to national and international research standards, this study was conducted according to ethical principles governing the usage of animal samples in research studies of this nature. Permission to use samples was also acquired from the Ethical Clearance Committee of Jilin Agricultural University. The management and care of animals were performed according to the guidelines and recommendations of the Jilin Agricultural University Guide (JNDZ [2019] No.19). This study was conducted under the ethical approval no. kt2015010 of Animal Experimental Ethics of Key Laboratory of Animal Production and Product Quality and Safety.
Collection of blood samples
Before blood collection, the animals were fasted for 24 h (Niu et al. Citation2013). Ten millilitre (10 mL) of blood were withdrawn from the jugular vein of pig (2-month old Landrace boar), from the heart of rabbits (2-month old experimental rabbit Japanese big-eared rabbits). Blood samples were withdrawn from the jugular vein of bovine (6-month old Holstein bull) (Yuan et al. Citation2018). In order to prevent blood clotting, anticoagulant (5% sodium citrate, 1 mL) was added and mixed evenly for the preparation of EM.
Preparation and purification of EM
The erythrocytes possess the highest weight compared to serum and the other formed elements. The blood samples were leuko-reduced by first centrifuging at 3000 rpm for 10 min to decant plasma. The packed cells were then centrifuged at 700 xg for 30 min with no brakes to remove the leukocytes. Because there is no nucleus and other organelles in mammalian erythrocyte, the preparation of the membrane was only through simple low permeability solution treatment and centrifugation. To improve the extraction efficiency of EM protein, we compared several common lysis buffers that had no effect on subsequent co-immunoprecipitation assays (CO-IP). All lysis buffers were obtained from Gene Star, Beijing, China. We evaluated the purification effects of three lysis buffers, including NP-40 lysis buffer, RIPA lysis buffer (weak), and RIPA lysis buffer (middle). A sample of 1 mL from each lysis buffer was used to precipitate the EM. After standing for 30 min, the supernatant was separated and then was centrifuged for 15 min at 4 °C and 10,000 xg, for SDS-PAGE electrophoresis. Different lysis buffers were compared through the analysis of electrophoretogram, and the most effective lysis buffer was selected to be used in the next experiment.
The obtained opalescent precipitate was dissolved in 1 mL of lysis buffer (PMSF contained), and ultrasonic breaked for 1 min (5 s per 10 s interval) at 80 Hz and 4 °C. After 40 min of standing at 4 °C, the proteins centrifugated at 4 °C and 10,000 xg for 15 minutes, then the supernatant was used as a protein solution for EM.
Sodium dodecyl sulfate-polyacrylamide gel electrophoresis (SDS-PAGE)
The extracted EM proteins were isolated by SDS-PAGE. EM proteins were re-suspended in a loading buffer (CWBIO, Beijing, China) with the ratio of 4:1 (sample weight to buffer weight). Then the samples were centrifuged and the supernatant was heated to 95 °C for 3 min. The boiled samples were kept in iced condition before loading into a gel.
Both 5% stacking gel and 10% separation gel were prepared for separating the proteins. 10 μL of 1.0 mg/mL protein samples, as well as protein marker (BioLab, Beijing, China) was applied on the gel. The SDS-PAGE was performed at 80 V through the stacking gel and 150 V through the separation gel. After fixation, these proteins were stained with coomassie brilliant blue at room temperature for 2 h, followed by washing with double-distilled water for 3 times.
CO-IP
CO-IP experiment was conducted to separate all specific proteins that specially combined with SBA on EM from pig, rabbit or as previously described in bovine (Yuan et al. Citation2018). CO-IP were carried out using dynabeads CO-IP kiit (Invitrogen, Carlsbad, CA). The experiment was strictly conducted in accordance with the manufacturer’s instructions. Prior to immunoprecipitation, 50 μg of SBA (Sigma, St. Louis, MO) was mixed with beads, and incubated on a roller at 37 °C overnight.
After washed 3 times with 0.01% Tween-20 HB solution, we transferred 1.5 mg SBA-coupled beads to a fresh tube. Then the SBA-coupled beads were re-suspended in 1.0 g EMs and incubated on a roller at 4 °C for 30 min. Then the Protein A beads were finally re-suspended in ethidium bromide (EB) solution and incubated on a roller at room temperature for 5 min. The immunoprecipitate was centrifuged and the supernatant which contained SBA-binding protein complexes was transferred to a clean tube.
Liquid chromatography coupled to tandem mass spectrometry (LC-MS-MS (Q-E)) detection
After separation by CO-IP, all SSBP were detected by Beijing Protein Innovation CO., Ltd. (Beijing, China) and analysed using an electro-spray ionisation tandem mass spectrometry LC-MS-MS (Q-E). MASCOT protein scores (combined MS and MS/MS spectra) >22 in rabbit, MASCOT protein scores > 23 in pig, and >24 in bovine (Yuan et al. Citation2018), were considered statistically significant (p < .05). The individual MS/MS spectrum, with a statistical significant (confidence interval >95%) iron score was accepted.
Retrieval of database
The database search was performed with Mascot Daemon (Version 2.3.0, Matrix Science). Bos taurus database was used for data analysis and was downloaded from UniProt. The database link is http://www.uniprot.org/taxonomy/9913?Sort = score.
Results
Comparison of purification effects of different lysis buffers on EM protein extraction
In this experiment, the time of haemolysis (overnight haemolysis) was prolonged on the basis of traditional hypotonic haemolysis method, and the method of ultrasonic fragmentation was used to accelerate the fragmentation of erythrocyte, so the breakage of erythrocyte and the cleavage of proteins from the membrane were more thorough.
The SDS-PAGE electrophoretogram results are shown in Figure . The results showed that NP-40 was the most effective lysis buffer compared to RIPA lysis buffer (medium) and RIPA lysis buffer (weak). In addition, the mild NP-40 lysis buffer had no effect on subsequent CO-IP. Such effective buffer was selected as the lysis buffer for the extraction of EM proteins.
Figure 1. Comparison of extraction effect of different lysis buffers on erythrocyte membrane in sodium salt (sds)-polyacrylamide gel electrophoresis (SDS-PAGE). M. Standard protein maker 1. Octylphenoxypolyethoxyethanol (NP-40) lysis buffer 2. Radio immunoprecipitation assay (RIPA) lysis buffer (medium) 3. RIPA lysis buffer (weak).
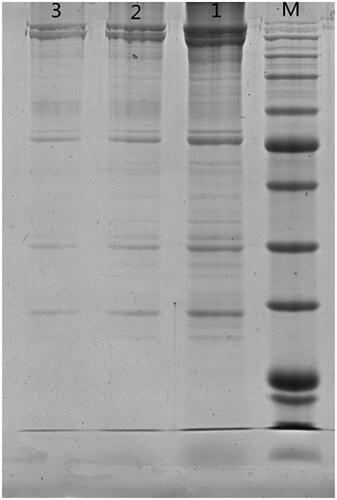
Comparison among EM proteins in different animal species
The protein compositions of EM of pig and rabbit were similar (Figure ). Most of these compositions were distributed between 25 kDa and 250 kDa, and the proportion of high molecular weight protein was significantly higher than that of low molecular weight protein. The difference was the protein abundance of 180 kDa, 65 kDa and 35 kDa in rabbit EM which was higher than that in EM of pig.
Identification of SSBP of EMs in different animal species
In herein experiment, SSBP on the surface of EM of pig and rabbit were detected and analysed using (LC-MS-MS (Q-E)). The Mascot software showed that the proteins with score higher than 23 in pig and with score higher than 22 in rabbit were all positive results. Then, we compared and analysed the SSBP of EMs in different animal species (pig, rabbit and that in bovine). Functionally, SSBP proteins could be divided into three protein categories including cytoskeleton, catalytic enzymes, and functional regulatory proteins. The cytoskeleton proteins were the main different expressed proteins among the EM proteins of pig, rabbit and that in bovine, as shown in Tables .
Table 1. The soybean agglutinin specific binding cytoskeleton protein of EMs in three animal species.
Table 2. The soybean agglutinin specific binding catalytic enzyme proteins in the EMs of three different animal species.
Table 3. Differences of soybean agglutinin specific binding functional regulatory proteins in EMs of different animal species.
The SSBP on bovine EM were the most abundant than the other two animal species (23 proteins in rabbit, 25 proteins in pig and 35 proteins in bovine). The cytoskeleton proteins that specifically bound with SBA on the EMs of these three species were mainly family members of the keratin, as depicted in Table and Figure . We found 12 overlapping SBA-specific binding cytoskeleton proteins among pig, rabbit, and bovine EM. There were mainly some family members of keratin, spectrin, actin and ankyrin 1. There were three overlapping SBA-specific binding cytoskeleton proteins in both pig and rabbit (some family members of keratin and ankyrin 2), three overlapping SSBP in both rabbit and bovine (family members of keratin), and five overlapping SSBP in both pig and bovine (family members of keratin). To expect the difference of cytoskeleton protein keratin subunits among these three animal species, the difference was detected for tubulin alpha chain specially in rabbit, while plakoglobin and protein 4.2 were specially expressed in pig, whereas band 3 protein, protein 4.1 and vimentin were specially expressed in bovine.
Figure 3. The overlapping and differential soybean agglutinin specific binding cytoskeleton proteins in three animal species.
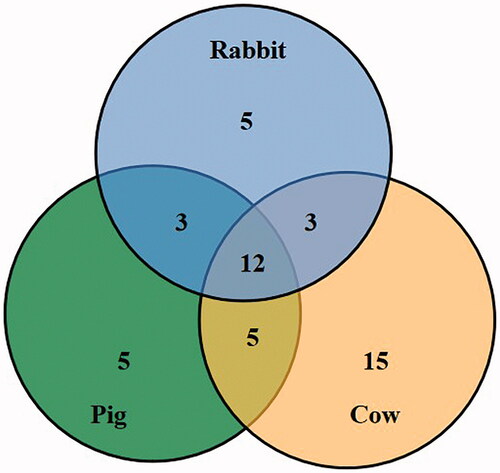
Regarding the SBA binding catalytic enzyme proteins, only 1 overlapping protein, (arginine methyltransferase 5) was expressed in the EM of these three animal species (Table and Figure ). The methyltransferase like 3, pyruvate kinase, enolase 2 and glutathione S-transferase theta-4 specially have expressed in rabbit, while trypsinogen and phosphodiesterase 4D interacting proteins were specially expressed in pig. Yet, adenosine deaminases, RNA-specific B1, receptor for activated C kinase 1, serine/threonine-protein kinase 38 were specially expressed in the bovine.
Figure 4. The overlapping and differential soybean agglutinin specific binding catalytic enzyme proteins in three animal species.
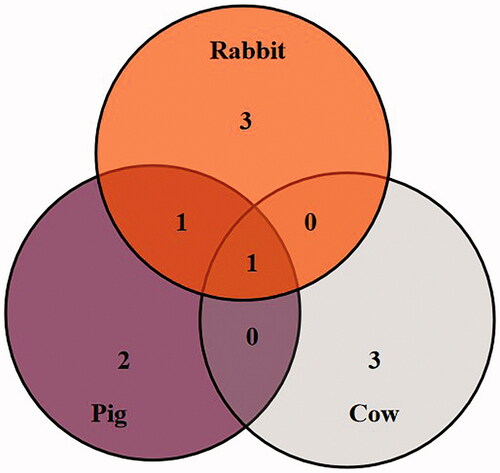
The highest difference was detected in the functional regulatory proteins expression (Table and Figure ). We found 1 overlapping SSBP, called solute carrier family 4 member 1 in both rabbit and pig. The calmodulin regulated spectrin associated protein 1 was specially expressed in pig. While three proteins were specially expressed in the bovine including heat shock 70 kDa protein 1A, T-complex protein 1 subunit beta, and 78 kDa glucose-regulated protein, while other five proteins were expressed in rabbit, including anion exchange protein, sodium channel protein, cullin 9, rap guanine nucleotide exchange factor like 1, family with sequence similarity 71member B.
Discussion
In this experiment, the extraction method of EM protein was optimised. The time of haemolysis (overnight haemolysis) was prolonged on the basis of traditional hypotonic haemolysis method. The method of ultrasonic fragmentation was used to accelerate the fragmentation of erythrocyte. Therefore, the breakage of erythrocyte and the cleavage of proteins from the membrane were more thorough than the original method.
The active ingredients in RIPA lysis buffer (Medium) were 50 mM Tris HCl (pH7.4), 150 mM NaCl, 1% NP-40, 0.5% sodium deoxycholate, 0.1% SDS with some other components like sodium pyrophosphate, sodium fluoride, EDTA. The difference in RIPA lysis buffer (low) and RIPA lysis buffer (medium) was noticed in sodium deoxycholate which was decreased by 0.25%. This difference was noticed in NP-40 lysis buffer in another component (sodium orthovanadate). Sodium deoxycholate, an anionic surfactant, can destroy the membranes of the damaged or non-viable cells to enhance permeability of cells (Ling et al. Citation2020). A related research indicated that sodium deoxycholate-based extraction buffer with heat denaturation was the most effective approach for highly efficient extraction of proteins from complex tissues such as the zebrafish liver tumour (Wang et al. Citation2015). In the present research, the increased content of sodium deoxycholate could improve the extraction effect of cell membrane protein. In addition, the sodium orthovanadate could effectively inhibit protein degradation and maintain the original protein–protein interaction. Therefore, the NP-40 lysis buffer was more effective than the other two kinds of lysates to extract of cell membrane protein.
The sensitivity of animal species to SBA is different (Kakiuchi et al. Citation2002), and the premise of SBA hemagglutination or anti-nutritional functions are related to species-specific effect with cell membrane protein (Dam and Brewer Citation2010). Hasan et al. (Citation2018) also reported that the decreased hemagglutination activity may be related to the effective irreversibly binding proteins of human kidney lectins to the erythrocyte membrane, lectins oligomerization or both actions. Moreover, chitosan nanoparticles can promote agglutination of rabbit blood cells by binding to the EM (Fan et al. Citation2012). Therefore, we speculated that the different sensitivity of erythrocyte to SBA among animal species may be ascribed to the variations of the SSBP on EM in such species.
Functionally, the results of SSBP in rabbit, pig and the precious report of bovine (Yuan et al. Citation2018). EM proteins were divided into three categories including cytoskeleton proteins, catalytic enzyme proteins, and the functional regulatory proteins. According to the current experimental results, the cytoskeleton proteins were the main different expressed proteins among the EM proteins in pig and rabbit than that in bovine. In these proteins, overlapping and differential proteins are mainly family members of keratin. Thakur et al. (Citation2016) reported that several herbal secondary bioactive compounds such as flavonoids and polyphenols possess anti-oxidant properties to stabilise EM by scavenging free radicals, and thereby protecting host cells from oxidative damage, which can be caused by haemolysis and hemagglutination. Therefore, the stability of EM is of great significance for erythrocyte agglutination. Previous studies have shown that there is a significant difference in SBA sensitivity among erythrocyte of different species (Contreras and Tagle Citation1974; Liu Citation2006). The most sensitive animal species for erythrocyte agglutination is rabbit, followed by pig, and bovine. The recent species shows agglutinate at a specific concentration (since there was a weak agglutination reaction in 8–64 times dilution of SBA for ruminant). From our results, the SSBP on bovine EM were the most abundant than the other two species (23 proteins in rabbit, 25 proteins in pig and 35 proteins in bovine). Additionally, these proteins were mainly family members of keratin and the functions of keratin, that can maintain the integrity and continuity of the cells. Band 3 protein and protein 4.1 were only detected in SSBP of the bovine. EM mechanical function is regulated by the spectrin-based membrane cytoskeleton, which composed of α-and β-spectrin, actin, protein 4.1R and adducing (Manno et al. Citation2005). One of EM major organising centres is the cytoplasmic domain of band 3, which links multiple proteins to the membrane (Zhang et al. Citation2000). Because the effect of SBA-specific binding cytoskeleton proteins on bovine EM is more abundant than the other to animals, the stability of bovine erythrocytes was higher than that of pig and rabbit. However, there is no research report on the relationship between keratin/band 3 protein/protein 4.1 and erythrocyte agglutination, these cytoskeleton proteins play an important role in maintaining EM stability. When SBA binds to these proteins, it can inhibit changes in cell structure and stability. Therefore, this inhibition can lead to decrease the sensitivity of bovine's erythrocytes to SBA than those of rabbit and pig.
We analysed other SBA-specific binding cytoskeleton proteins that were related to erythrocyte agglutination on EMs of these three animal species. Except some members of keratin family, tubulin alpha chains are SSBP that could only be expressed on the EM of rabbit. Tubulin, the subunit protein of microtubules, is found in all eukaryotic cells. Microtubules play an important in various cellular activities such as mitosis, cell shaping, secretion, and motility. Previous investigations reported that tubulin plays an important role in hemagglutination, 100 μg of tubulin per 1 mL of packed formalinized cells during the coating procedure can cause a significant agglutination (Gozes et al. Citation1977; Kim et al. Citation2001). Therefore, when the tubulin alpha protein interacts with SBA, may be leading to significant agglutination sensitivity to erythrocyte of rabbit. In addition to ankyrin1, the overlapped proteins of rabbit and pig also contained ankyrin 2, which was lack in bovine EM. Minion and Goguen (Citation1985) showed that the attachment of EM proteins to a semi-rigid cytoskeletal network prevents or greatly reduces their lateral diffusion. The erythrocyte from ankyrin-deficient mice in which the linkage between glycophorin and cytoskeleton is disrupted and can be agglutinated by M. pulmonis. Thus, ankyrin 2 may declare the sensitive agglutination potential for SBA in the erythrocytes of rabbit and pig than bovine. After the binding of SBA to microproteins, the expression or activity of ankyrin may cause erythrocyte agglutination. These proteins may be some pathways among SBA-induced-hemagglutination in different animal species; however, these conjectures still need to be confirmed by further studies.
Protein kinases and transferases are the most important enzymes in cell membrane signal transduction (Pajaud et al. Citation2012). Once these enzymes have activated, they can induce many intracellular reactions. Most catalytic enzymes detected in our results belong to these two enzymes. Among the results of SBA-specific binding catalytic enzymes on EMs of different animal species, the major enzyme related to erythrocyte agglutination was erythrocyte pyruvate kinase (PK), which detected only in rabbit. Erythrocyte PK represents one of the rate limiting key enzymes in the Embden–Meyerhof pathway, which the erythrocyte depends to get the energy and viability (Nakashima Citation1974). The deficiency of this enzyme leads to decrease of energy supply. Pollack and Reckel (Citation1977) reported that the immune agglutination of erythrocyte also needs two major opposing energy sources. First, the attraction energy which mostly comes from interfacial energy, the kinetic or thermally driven Brownian motion. Such attraction energy is opposed by the second energy source, the repulsion energy, due to ζ-potential. Therefore, when SBA combined with erythrocyte PK, this may affect the energy supply, and finally leading to exhibit the agglutination sensitivity of rabbit's erythrocyte to SBA.
The functional regulatory proteins in the erythrocyte are necessary for the cell metabolism. These functions involve transportation for oxygen, carbon dioxide, electrolytes, glucose, amino acids, etc. The results from the SBA-binding functional regulatory proteins only showed a typical trans-membrane protein, solute carrier family 4 member 1 in rabbits and pig. Such functional proteins play an important role in maintaining the stability of EM structure and regulating energy metabolism. Other proteins are responsible for the transport of amino acids, sugars, nucleotides and inorganic ions through the cell membranes. Therefore, we speculated that functional regulatory proteins may be a marginal reason for the sensitivity difference of EM proteins to SBA in different animal species.
In this study, we just compared the differences of SSBP on EM proteins from three representative animal species, as the agglutination activity of SBA on erythrocytes of these three animals is quite different. Although we have detected the binding between the proteins, the current study has a limitation in evaluating the strength of the various proteins. Therefore, further studies should be conducted to evaluate the binding strength of SSBP on the EM of different animal species, and to perform a systematic analysis of these differences. In addition, we could also verify the function of these different SSBP, and indicate the reason and pathway for the difference of SBA agglutination activity of erythrocyte of different animal species. This study is of great theoretical significance for further understanding and analysing the toxic mechanism of SBA. Such study is of great practical value to the diversity and precision feeding of different animal groups, as well as to optimise the feed resource allocation and feed processing and utilisation.
Conclusions
In conclusion, NP-40 lysis buffer was the most effective lysates in the extraction of EM proteins. The specific binding cytoskeleton proteins on erythrocyte membrane of different animal species may be one important reason for the difference of the sensitivity of rabbit, pig, and bovine erythrocyte to SBA. The protein kinases (erythrocyte PK) and the functional regulatory proteins (solute carrier family 4 member 1) may be another reason for the most sensitive agglutination in rabbit erythrocyte than that of pig and bovine to SBA. This research provides an important experimental basis for revealing the pathway of the SBA-induced hemagglutination variations among erythrocytes of different animal species.
Disclosure statement
We certify that there is no conflict of interest with any financial organisation regarding the material discussed in the manuscript. The authors alone are responsible for the content and writing of this article.
Additional information
Funding
References
- Contreras S, Tagle M. 1974. Factores toxicos de leguminosas cultivadas en Chile. III. Hemaglutininas. Arch Latinoam Nutr. 24(2):191–199.
- Dam TK, Brewer CF. 2010. Chapter 5 – multivalent lectin–carbohydrate interactions: energetics and mechanisms of binding. In: Horton D, editor. Advances in carbohydrate chemistry and biochemistry. London: Academic Press; p. 139–164.
- Fan W, Yan W, Xu Z, Ni H. 2012. Erythrocytes load of low molecular weight chitosan nanoparticles as a potential vascular drug delivery system. Colloids Surf B Biointerfaces. 95:258–265.
- Goldstein IJ, Hughes RC, Monsigny M, Osawa T, Sharon N. 1980. What should be called a lectin? Nature. 285(5760):66–66.
- Gordon-Smith T. 2009. Structure and function of red and white blood cells. Medicine. 37(3):119–124.
- Gozes I, Littauer UZ, Geiger B, Fuchs S. 1977. Immunochemical determination of tubulin. FEBS Lett. 73(1):109–114.
- Guesdon JL, Ternynck T, Avrameas S. 1979. The use of avidin-biotin interaction in immunoenzymatic techniques. J Histochem Cytochem. 27(8):1131–1139.
- Halder S, Surolia A, Mukhopadhyay C. 2016. Dynamics simulation of soybean agglutinin (SBA) dimer reveals the impact of glycosylation on its enhanced structural stability. Carbohydr Res. 428:8–17.
- Hasan HR, Husain MK, Jaseim RH. 2018. Detection of malignant human kidney lectins and study of its optimum hemagglutination conditions. Proceedings of 3rd Scientific Conference of the College of Science, University of Baghdad, 24–26 March Baghdad; p. 1563–1577.
- Hughes-Jones NC, Gardner B, Telford R. 1963. Studies on the reaction between the blood-group antibody anti-D and erythrocytes. Biochem J. 88(3):435–440.
- Kakiuchi M, Okino N, Sueyoshi N, Ichinose S, Omori A, Kawabata S-i, Yamaguchi K, Ito M. 2002. Purification, characterization, and cDNA cloning of alpha-N-acetylgalactosamine-specific lectin from starfish, Asterina pectinifera. Glycobiology. 12(2):85–94.
- Kim SW, Ihn KS, Han SH, Seong SY, Kim IS, Choi MS. 2001. Microtubule- and dynein-mediated movement of Orientia tsutsugamushi to the microtubule organizing center. Infect Immun. 69(1):494–500.
- Ling N, Shen J, Guo J, Zeng D, Ren J, Sun L, Jiang Y, Xue F, Dai J, Li B. 2020. Rapid and accurate detection of viable Vibrio parahaemolyticus by sodium deoxycholate-propidium monoazide-qPCR in shrimp. Food Control. 109:106883.
- Liu LN. 2006. Comparative studies on the sensitivity to SBA in different species of animal [MSc]. Changchun City (China): Jilin Agricultural University.
- Lotan R, Lis H, Rosenwasser A, Novogrodsky A, Sharon N. 1973. Enhancement of the biological activities of soybean agglutinin by cross-linking with glutaraldehyde. Biochem Biophys Res Commun. 55(4):1347–1355.
- Lotan R, Siegelman HW, Lis H, Sharon N. 1974. Subunit structure of soybean agglutinin. J Biol Chem. 249(4):1219–1224.
- Manno S, Takakuwa Y, Mohandas N. 2005. Modulation of erythrocyte membrane mechanical function by protein 4.1 phosphorylation. J Biol Chem. 280(9):7581–7587.
- Minion FC, Goguen JD. 1985. Diffusion of target cell membrane proteins allows recognition of cryptic binding sites by Mycoplasma pulmonis hemagglutinin. Infect Immun. 48(2):579–580.
- Nakashima K. 1974. Further evidence of molecular alteration and aberration of erythrocyte pyruvate kinase. Clin Chim Acta. 55(2):245–254.
- Niu W-Y, Wu S, Zhang Y, Feng Y-N, BiaN J-Q, Zhang Y, Xiao H-B. 2013. Biological study on the components of erythrocyte membrane of Qi deficiency and blood stasis rats. Inform Tradit Chin Med. 4:332.
- Pajaud J, Kumar S, Rauch C, Morel F, Aninat C. 2012. Regulation of signal transduction by glutathione transferases. Int J Hepatol. 2012:137676.
- Pan L, Qin G, Zhao Y, Wang J, Liu F, Che D. 2013. Effects of soybean agglutinin on mechanical barrier function and tight junction protein expression in intestinal epithelial cells from piglets. IJMS. 14(11):21689–21704.
- Pereira MEA, Kabat EA, Sharon N. 1974. Immunochemical studies on the specificity of soybean agglutinin. Carbohydr Res. 37(1):89–102.
- Pollack W, Reckel RP. 1977. A reappraisal of the forces involved in hemagglutination. Int Arch Allergy Appl Immunol. 54(1):29–42.
- Tateno H, Saneyoshi A, Ogawa T, Muramoto K, Kamiya H, Saneyoshi M. 1998. Isolation and characterization of rhamnose-binding lectins from eggs of steelhead trout (Oncorhynchus mykiss) homologous to low density lipoprotein receptor superfamily. J Biol Chem. 273(30):19190–19197.
- Thakur P, Chawla R, Narula A, Goel R, Arora R, Sharma RK. 2016. Anti-hemolytic, hemagglutination inhibition and bacterial membrane disruptive properties of selected herbal extracts attenuate virulence of carbapenem resistant Escherichia coli. Microb Pathog. 95:133–141.
- Tomova E, Arnaudov A, Velcheva I. 2008. Effects of zinc on morphology of erythrocytes and spleen in Carassius gibelio. J Environ Biol. 29(6):897–902.
- Tremblay R, Feng M, Menassa R, Huner NPA, Jevnikar AM, Ma SW. 2011. High-yield expression of recombinant soybean agglutinin in plants using transient and stable systems. Transgenic Res. 20(2):345–356.
- Tse WT, Lux SE. 1999. Red blood cell membrane disorders. Br J Haematol. 104(1):2–13.
- Wang J, Lee YM, Li C, Li P, Li Z, Lim TK, Gong Z, Lin Q. 2015. Dramatic improvement of proteomic analysis of zebrafish liver tumor by effective protein extraction with sodium deoxycholate and heat denaturation. Int J Anal Chem. 2015:763969.
- Yuan ZJ, Zhao Y, Pan L, Sun ZY, Naren GW, Qin G. 2018. Separation and identification of specific soybean agglutinin binding proteins to cow erythrocyte membrane. Chin J Vet Sci. 38(4):814–818.
- Zhang D, Kiyatkin A, Bolin JT, Low PS. 2000. Crystallographic structure and functional interpretation of the cytoplasmic domain of erythrocyte membrane band 3. Blood. 96(9):2925–2933.