Abstract
This study investigated changes in oxidative stress and the relevant mechanisms in the liver of goats fed a high-concentrate (HC) diet for 5 weeks. Twelve goats were randomly assigned to a low-concentrate (concentrate-to-forage = 55:45, LC, n = 6) or HC diet (concentrate-to-forage = 90:10, n = 6), with dry matter as the base. Enzyme activity assays, real-time polymerase chain reaction and western blotting were used to evaluate antioxidant parameters and gene expression in the liver. Superoxide dismutase (SOD), glutathione peroxidase (GPx), catalase (CAT), total nitric oxide synthase activity and total antioxidant capacity (T-AOC) in the liver declined (p < .01) in HC-fed goats compared to those in LC-fed goats. The mRNA levels of GPX1, CAT and SOD1 were down-regulated (3.69, 47.37 and 27.61%, respectively) in HC-fed goats compared to those in LC-fed goats. Furthermore, glutathione S-transferase M1 (GSTM1) was upregulated (466.35%, p < .01) in the liver of HC-fed goats. The mRNA and protein levels of the nuclear factor E2-related factor 2 (NRF2) and total glucocorticoid receptor (GR) declined (p < .05) in HC-fed goats (by 28.57, 33.1, 30.85 and 34%, respectively). However, the nuclear translocation of GR increased (p < .05; by 44.75%) in HC-fed goats. Negative correlations were detected for hepatic nuclear GR protein expression with hepatic CAT activity and GPx activity. In conclusion, feeding an HC diet to goats for 5 weeks suppressed NRF2-dependent antioxidant responses and enhanced GR nuclear translocation in the liver.
Feeding goats high-concentrate diets suppresses NRF2-dependent antioxidant responses in liver, downregulating NRF2 and altering SOD1, GPx and CAT activity.
This suppression of NRF2-dependent antioxidant responses is associated with GR activation in the liver of goats.
Highlights
Introduction
Oxidative stress is an imbalance between the production of cell-damaging free radicals and the body’s ability to neutralise them, which usually causes cellular and tissue damage (Trevisan et al. Citation2001; Zhang et al. Citation2015). Recently, several studies have shown that stress responses arise in ruminants fed high-grain or high-starch diets, resulting in oxidative damage and increased levels of acute-phase proteins (Sgorlon et al. Citation2008; Jia et al. Citation2014; Duanmu et al. Citation2016; Abaker et al. Citation2017). It is widely accepted that oxidative stress is a significant factor responsible for impaired immune and inflammatory responses, and it is positively correlated to the high-grain or high-starch diets of ruminants (Tao et al. Citation2015; Citation2016; Aditya et al. Citation2018). It has also been reported that feeding high-concentrate (HC) diets to lactating cows reduces pH and increases the release of lipopolysaccharide (LPS) from the rumen and hindgut (Zebeli and Ametaj Citation2009). LPS induces hepatic oxidative injury by changing the enzyme activity of glutathione and superoxide dismutase (SOD) (Taira et al. Citation2008; Abdel-Salam et al. Citation2012). The HC diet causes oxidative stress but the relevant mechanism is unclear.
Cortisol is the primary glucocorticoid (GC) and is a regulator of physiological responses to stress (Yamaji et al. Citation2009). Serum cortisol levels are usually elevated after exposure to acute stressors, including oxidative stress in dairy cows (Laven and Peters Citation1996; Gupta et al. Citation2005). GC increases in response to stress via the hypothalamic–pituitary–adrenal (HPA) axis, influencing the intracellular glucocorticoid receptor (GR) and modulating the expression of different target genes (stress-response genes) (Prunet et al. Citation2006; Stahn and Buttgereit Citation2008; Ayroldi et al. Citation2012). Previous studies have shown that hepatic GR protein expression is downregulated in lactating goats fed an HC diet for 9 weeks (Dong et al. Citation2013). Other experiments have demonstrated that the presence of oxidative stress in many chronic inflammatory diseases generally weakens GR function, and leads to GC insensitivity in several cell systems (Chung and Marwick Citation2010; Hakim et al. Citation2013). Oxidative stress levels are also elevated in the primary cells of patients with severe asthma, where GC sensitivity is closely linked to reduced GR nuclear import (Matthews et al. Citation2004). GR activation is associated with the suppression of antioxidant response signalling through the inhibition of nuclear factor E2-related factor 2 (NRF2). This is a pleiotropic transcription factor that contributes to the regulation of the antioxidant cell defense system (Copple et al. Citation2008; Muthusamy et al. Citation2012). This factor also upregulates antioxidant enzymes, including SOD, catalase (CAT), and heme oxygenase-1, in hepatic cells (Chen et al. Citation2017). In contrast, the downregulation of NRF2 signalling is considered to increase the levels of antioxidant enzymes (Kratschmar et al. Citation2012; Alam et al. Citation2017).
Existing studies demonstrated that the nuclear translocation of GR is associated with suppressed antioxidant response signalling via NRF2. Yet, knowledge on the underlying mechanism of oxidative stress in response to HC diets in ruminants remains limited. Here, we hypothesised that HC diets would increase the nuclear translocation of GR in the liver of goats. Thus, we aimed to: (1) investigate changes to oxidative stress parameters in the liver of growing goats fed an HC diet for 5 weeks, and (2) explore hepatic GR nuclear translocation and NRF2-mediated antioxidant responses underlying HC diet-induced oxidative stress.
Materials and methods
This experiment was conducted following the recommendations of the Animal Care and Use Guidelines of the Institute of Subtropical Agriculture, Chinese Academy of Sciences, China. The protocol was approved by the Animal Care Committee on the Ethics of Animal Experiments of Institute of Subtropical Agriculture (Permit No. 20170712). All surgeries were performed after euthanasia, and all efforts were made to minimise any suffering of animals.
Animals and experimental procedures
Twelve healthy growing goats with an average initial body weight of 12 ± 1.35 kg were used as the animal model in this study. Animals were housed in individual stalls of a standard animal feeding house at the Institute of Subtropical Agriculture (ISA), Chinese Academy of Sciences (Changsha, China). All animals were randomly allocated to two groups [low-concentrate (LC) group versus HC group, n = 6). The experimental period was 5 weeks. The first week was used as an acclimation period to the diet and the last 4 weeks were used to perform the measurements (Wang et al. Citation2019). The LC group (control) was fed a low-concentrate (concentrate-to-forage = 55:45) diet. The HC group was fed a high-concentrate (concentrate-to-forage = 90:10) diet. Equal quantities of each diet were offered at approximately 08:00 and 18:00. Goats were given free access to fresh water throughout the experimental period. The feed intake of each goat was recorded daily. Dry matter intake was 572 ± 24.12 and 602 ± 62.86 g/day, crude protein intake was 77 ± 1.41 and 106 ± 2.71 g/day and energy intake was 5.77 ± 0.023 and 7.56 ± 0.034 MJ/day for LC and HC goats, respectively (as published in Wang et al. Citation2019). Goats in the LC and HC groups showed weight gains of 1.88 ± 0.25 and 3.5 ± 0.34 kg (Wang et al. Citation2019), respectively. The ingredients and nutrient compositions of the experimental diets are presented in Table . Feed samples were analysed in triplicate for crude protein (method 984.13; AOAC Citation2005), crude fat (method 2003.05; AOAC Citation2005) and crude ash (method 942.05; AOAC, Citation2005). Neutral detergent fibre content and acid detergent fiber content were determined according to Van Soest et al. (Citation1991), using an Ankom 220 Fiber Analyser (Ankom Co.), and were expressed inclusive of residual ash.
Table 1. Ingredients and composition of the experimental diets (% on dry matter).
Sample collection
After 4 weeks of feeding, all goats were euthanized by injecting xylazine (0.5 mg/kg; Xylosol; Ogris Pharma, Wels, Austria) and pentobarbital (50 mg/kg WDT, Garbsen, Germany) in the jugular (before overnight fasting and exsanguination). After euthanasia, liver tissue (the medial lobes of the liver) was carefully removed, immediately frozen in liquid nitrogen and stored at −80 °C until RNA isolation and protein extraction.
Preparation of liver homogenate
Antioxidant assays were performed using assay kits purchased from the Nanjing Jiancheng Bioengineering Institute (Nanjing, China). Frozen liver tissue (1 g) was homogenised in 10-mL physiological saline (0.9% NaCl; 4 °C) on ice using a Polytron-aggregate homogeniser PT-1200E (Lucerne, Switzerland) for 30 s at 12,000×g. The homogenate was centrifuged at 3800×g for 10 min at 4 °C. The resulting supernatant (10% concentrations) was aliquoted and stored at −20 °C until analysis.
Total antioxidant capacity (T-AOC), antioxidant enzyme activity and lipid peroxidation in the liver
Various liver parameters were analysed using their corresponding reagent kits (Nanjing Jiancheng Bioengineering Institute, Nanjing, China). These included T-AOC, hepatic SOD, CAT, glutathione peroxidase (GPx) enzyme activity, malondialdehyde (MDA) concentrations and total nitric oxide synthase (NOS). All procedures were performed according to the manufacturer’s instructions. SOD (EC1.15.1.1), CAT (EC1.11.1.6), GPx (EC1.11.1.9) and NOS (EC1.14.13.39) activity were expressed as units/mg protein. T-AOC and MDA were expressed as mmol/mg protein.
RNA extraction and cDNA synthesis
Total RNA from the liver tissue of each goat was extracted using Trizol reagent (Invitrogen, Carlsbad, CA) according to the manufacturer’s protocols. The concentration of isolated total RNA was quantified with an ND-1000 UV-vis spectrophotometer (NanoDrop Ltd., Wilmington, DE). The integrity of RNA was inspected using 1% agarose gel electrophoresis (Supplementary Figure S1). Protein contamination was assessed through the spectrophotometric determination of the 260–280 nm absorbance ratio. Only samples with a ratio between 1.8 and 2.1 were used in subsequent experiments. To remove traces of chromosomal DNA, 1 µg of total RNA was treated with 5× g DNA Eraser Buffer, 2.0 μL Eraser Buffer, 1.0 μl g DNA Eraser and RNase-free DNase I (TaKaRa, Japan) for 2 min at 42 °C. Subsequently, first-standard cDNA was synthesised using a PrimeScriptTM RT reagent Kit (TaKaRa, Japan) and stored at −20 °C for real-time polymerase chain reaction (RT-PCR).
RT-qPCR analysis
Oligonucleotide primer sequences for SOD1, CAT, GPx1, GSTM1, GSTP1 and β-actin were obtained from previously published papers (Yang et al. Citation2014; Duanmu et al. Citation2016). NRF2- and GR-specific primers were designed using Premier 5 software (Premier Biosoft, Palo Alto). The RT-PCR products of NRF2 and GR-specific primers were sequence-validated. The identity of NRF2 and GR was 99 and 100%, respectively, indicating that the RT-PCR products were the target genes. Details on the oligonucleotide primer sequences, primer lengths and accession numbers are listed in Table . RT-PCR was performed using SYBR® Premix EX TaqTM (TaKaRa, Japan) on a LightCycler480 system (Roche, Castle Hill, New South Wales, Australia). The cDNA was diluted fourfold before equal amounts were added to duplicate RT-PCR reactions. The thermal profile for all reactions was 30 s at 95 °C, followed by 40 cycles of denaturation at 95 °C for 5 s, annealing at 60 °C for 20 s and extension at 72 °C for 1 min. The specificity of PCR amplification was confirmed by melting curve analysis and 2% agarose gel electrophoresis of the PCR products. The efficiency of PCR amplification for each gene was checked by diluting the samples. Relative expression levels of target genes were normalised to those of β-actin (Jia et al. Citation2014; Yang et al. Citation2014). Data were analysed using the 2−ΔΔCt method, following existing procedures (Livak and Schmittgen Citation2001). All samples were included in the same run of RT-PCR. The experiment was repeated at least three times.
Table 2. Primer sequences of the target genes.
Western blot analysis
Total protein was extracted from frozen liver tissue using 1 mL of ice-cold homogenisation RIPA buffer containing the protease inhibitor cocktail Complete EDTA free (Roche, Penzberg, Germany) and the phosphatase inhibitor Cocktail PhosSTOP (Roche, Penzberg, Germany). Cytosolic proteins, membrane proteins and nuclear proteins were separated with the Nucl-Cyto-Mem Preparation Kit (Applygen Technologies, Beijing, China), according to the manufacturer’s protocol. Protein concentration was determined with an enhanced BCA protein assay kit (P0010, Beyotime Biotechnology, Shanghai, China). Protein extracts were subjected to 10% sodium dodecyl sulphate-polyacrylamide gel electrophoresis. Isolated proteins were then transferred to polyvinylidene fluoride membranes (PVDF membranes, Millipore, Danvers, MA). Western blot analysis for GR and NRF2 was performed with specific primary antibodies (Supplementary Table S1) and corresponding secondary antibodies (Supplementary Table S1). Finally, the blot was washed and detected through enhanced chemiluminescence (ECL) using Luminata Classico Western HRP Substrate (WBLUC0100, Millipore, Danvers, MA). ECL signals were recorded using an imaging system (Bio-Rad), and were analysed using Quantity One software (Bio-Rad). GR and NRF2 protein values were presented as fold-change relative to the average value of the LC group.
Statistical analysis
All data are presented as the mean ± SD. Statistical significance was assessed using the independent sample t-test in SPSS (SPSS version 21.0 for Windows; SPSS Inc., Chicago, IL) under the conditions of normal distribution and homogeneity of variance. Differences were considered significant at p < .05, and .05 < p < .10 was considered a tendency towards significance. Graphs were created using GraphPad Prism version 5.01 (GraphPad Software, La Jolla, CA). Pearson correlations were analysed in GraphPad Prism.
Results
Antioxidant enzyme activity, and T-AOC and MDA content
The results showed that SOD, GPx, CAT and NOS enzyme activities, as well as T-AOC, decreased significantly (p < .01) in HC goats compared to those in LC goats. However, there was no significant difference (p > .01) in MDA between the two groups (Table ).
Table 3. Comparison of liver antioxidant enzyme activity, lipid peroxidation, total antioxidant capacity and NOS activity in goats fed high- (HC) versus low-concentrate (LC) grain diets.
mRNA expression of antioxidant enzymes, encoding genes GR and NRF2
Hepatic GPX1, CAT, SOD1, NRF2 and GR mRNA expression levels decreased significantly (p < .05) in HC goats compared to those in LC goats (Table ). Among the GST family genes, hepatic GSTP1 mRNA expression tended to decrease more (p = .061) in the liver of HC versus that in LC goats, whereas GSTM1 gene expression increased significantly (p < .01).
Table 4. Real-time PCR analysis of relative mRNA expression in the liver of goats fed the high- (HC) versus low-concentrate (LC) grain diets.
Liver protein expression levels of GR and NRF2
Total liver protein expression of NRF2 decreased significantly (p < .05) in HC goats compared to that in LC goats (Figure ). However, liver GR protein expression in the whole cell and cytoplasm extract also decreased significantly (p < .05) in HC goats versus that in LC goats (Figure ), whereas GR protein expression in the cell nuclear extract significantly increased (p < .05; Figure ). There was no significant difference (p = .254) in GR protein expression in the liver cell membrane between the two groups.
Figure 1. Western blot analysis of the relative expression of liver NRF2, β-actin, GR, Lamin B1, β-tubulin and Na+/K+-ATPase protein in goats fed high- (HC) versus low-concentrate (LC) grain diets. (A) NRF2 expression in total liver protein; (B) GR expression in the cell membrane (M), cytoplasm (C) and nucleus (N), and hepatic total GR protein expression (T). Cell membrane, cytoplasm and nucleus fractions were obtained by standard subcellular fractionation and were analysed by western blotting. Controls of the cell membrane, cytoplasm and nucleus fractions were performed using an antibody against Na+/K+-ATPase, β-tubulin and Lamin B1, respectively. The Mw of β-actin, GR, NRF2, Lamin B1, β-tubulin and Na+/K+-ATPase proteins is 42, 95, 68, 67, 55 and 100 kDa, respectively. These autorads shown from a single goat are representative of six goats. *p < .05 versus LC. All data are presented as the mean ± SD. n = 6/group. GR: glucocorticoid receptor; NRF2: nuclear factor E2-related factor 2; Na+/K+-ATPase: sodium-potassium ATPase pump.
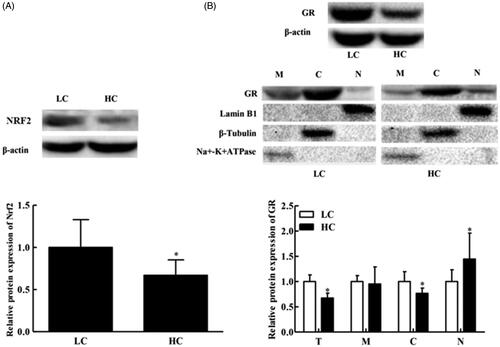
Correlation between hepatic GR protein expression and antioxidant enzymes
Hepatic nuclear GR protein expression was negatively correlated with hepatic CAT (r = −0.56, p = 0.048) and GPx activity (r = −0.59, P = .04; Figure ). No significant correlations were observed between nuclear GR protein expression and SOD, NOS, T-AOC or MDA levels (Figure ).
Figure 2. Pearson’s correlation of hepatic nuclear GR protein expression with hepatic antioxidant enzyme activity, as well as NOS, T-AOC and MDA level in goats. (A) SOD versus the nuclear expression of GR protein in the liver; (B) CAT versus the nuclear expression of GR protein in the liver; (C) GPx versus the nuclear expression of GR protein in the liver; (D) NOS versus the nuclear expression of GR protein in the liver; (E) T-AOC versus the nuclear expression of GR protein in the liver; (F) MDA versus the nuclear expression of GR protein in the liver. Data were analysed using Pearson’s model with SPSS (SPSS version 21.0 for Windows; SPSS Inc., Chicago, IL). All data are presented as the mean ± SD. n = 6/group. The results indicate a significant correlation based on the P-value at the 0.05 and 0.01 levels. CAT: catalase; GPx: glutathione peroxidase; GR: glucocorticoid receptor; MDA: malondialdehyde; NOS: total nitric oxide synthase; T-AOC: total antioxidant capacity; SOD: superoxide dismutase.
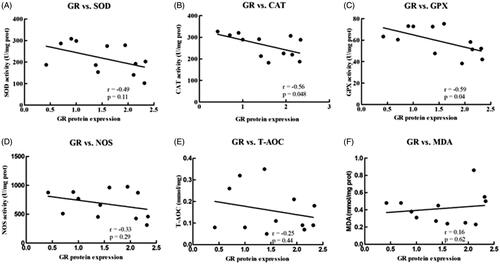
Discussion
The current study demonstrates that antioxidant activity declines in the liver of goats fed HC diets versus those fed LC diets for 5 weeks. Oxidative stress occurs when oxidant–antioxidant systems become imbalanced, potentially through the generation of more free radicals and the decline in antioxidant activity. Reduced SOD, CAT and GPx activity probably enhances the production of O2 and H2O2, and the dysfunction of the antioxidant defense system (Spolarics Citation1996; Fischer et al. Citation2012), as demonstrated previously. For example, feeding an HC diet to lactating goats reduces SOD and GPx activity (Tao et al. Citation2015; Duanmu et al. Citation2016). SOD and CAT are the primary antioxidant enzymes that scavenge superoxide anions in the tissue and are generally regulated by oxidative stress (Xiang et al. Citation2016). The antioxidant capacity in the liver of goats fed an HC diet appeared to decline, based on reduced SOD, GPx and CAT activity, and corresponding changes to the relative mRNA levels of SOD1, GPX1 and CAT genes. GPx activity in the brain and liver was previously reported to decline in lactating mice, which exhibited 254% higher gross energy intake than that in non-lactating mice (Zheng et al. Citation2015). The energy intake of HC goats in the current study was 7.56 ± 0.034 MJ/day, which was 31% higher than that of LC goats. Hence, the reduced antioxidant capacity of goats fed an HC diet might be the result of increased energy intake.
Furthermore, cows fed a high-grain diet exhibited higher levels of LPS, both in rumen fluid and plasma (Abaker et al. Citation2017). The decreased antioxidant capacity in goats fed an HC diet might also be explained by an increase in LPS concentrations (Tao et al. Citation2014). Reduced T-AOC levels also explained the decline in antioxidant capacity in this study. Moreover, nitric oxide (NO) strongly contributes towards mediating oxidative stress at a certain physiological concentration. In comparison, excessive NO generated by NOS (inducible NOS and endothelial NOS) forms nitrogen-free radicals, causing oxidative stress (Zhang et al. Citation2017). However, our results showed that NOS activity in the liver declined under an HC diet. This finding supports the results of Chen et al. (Citation2017), who documented a similar down-regulation in neuronal NOS expression for the hippocampus under acute stress. One possible explanation is the feedback inhibition of hepatic NOS activity via NO (Alderton et al. Citation2001). Oxidative stress-related genes (GSTM1 and GSTP1) also contribute to the control of cellular redox regulation, providing protection against cellular DNA oxidative damage. The absence of GSTM1 and GSTP1 increases the accumulation of oxidative DNA damage (Savic-Radojevic et al. Citation2013; Mian et al. Citation2016). In this study, the mRNA expression of GSTM1 increased, whereas that of GSTP1 tended to decrease. This result was consistent with a report stating that HC diets mediated decreases in antioxidant capacity, up-regulating GSTM1 and down-regulating GSTP1 mRNA levels, in lactating dairy goats (Duanmu et al. Citation2016). The upregulation of GSTM1 expression in this study might be an adaptive response to increased peroxidative stress. The reduced expression of GSTP1 might explain why HC diets suppress antioxidant responses in goats.
Oxidative stress is generally activated in response to challenges that arise when organisms are exposed to external stimuli (Gessner et al. Citation2013). NRF2 is an important mechanisms used to protect against external stimuli. As an essential transcription factor, NRF2 regulates the expression of antioxidant enzymes by binding to the antioxidant-response element (ARE), thus enhancing cellular defense against oxidative stress (Huang et al. Citation2014). In contrast, the suppression of the NRF2 antioxidant pathway might give rise to oxidative stress in tissues. Goats fed an HC diet in this study exhibited down-regulation in the expression of NRF2 and NRF2-regulated antioxidative genes, such as SOD1, CAT, GPX1 and GSTP1. These results are consistent with reduced SOD, CAT and GPx activity in the liver of HC-fed goats. Thus, the HC diets fed to goats might reduce antioxidant responses in a NRF2-dependent manner. This finding was consistent with an earlier report showing that hepatic NRF2 expression is inhibited higher in HC-fed dairy cows compared to that in LC-fed dairy cows (Abaker et al. Citation2017). Furthermore, the decreased expression of NRF2 might be attributed to GR activation (Ki et al. Citation2005). The activation of GR usually suppresses the NRF2-dependent antioxidant response (Kratschmar et al. Citation2012). GR is a low-affinity receptor, contributing to the regulation of many genes involved in biotransformation reactions, and in adjustments to altered energy demands.
Previous studies have proposed that the HPA is activated by HC-diet challenge or other stressors in domestic farm animals (Minton Citation1994; Jia et al. Citation2014). Hepatic GR mRNA and protein expression levels were down-regulated in HC goats in this study. HC diets reduce hepatic GR protein expression in goats to modulate the immune response and nutrient metabolism (Dong et al. Citation2013). Evaluation of the sub-cellular distribution of GR in this study showed that (compared to those in the LC group) GR nuclear translocation was higher, while cytoplasmic retention of GR was lower in the HC group. Thus, HC diets are likely to activate the HPA. The nuclear translocation of GR suppresses NRF2-mediated antioxidant responses. This phenomenon was demonstrated in peripheral blood mononuclear cells. Specifically, reduced GR nuclear translocation and increased cytoplasmic retention of GR under melatonin treatment causes the up-regulation of NRF2 (Kratschmar et al. Citation2012; Singh and Haldar Citation2016). Moreover, decreased liver antioxidant enzyme activity (SOD, CAT and GPx) generally contributes towards reducing total GR levels and GR activation (GR nuclear translocation) (Liu et al. Citation2008; Skuza et al. Citation2011; Djikic et al. Citation2012; Wei et al. Citation2013). In this study, the expression levels of hepatic nuclear GR proteins were negatively correlated with hepatic CAT and GPx activity. Thus, GR likely contributes towards regulating antioxidant responses in goats fed HC diets.
Conclusions
Our data suggest that feeding goats HC diets induces a reduction in the antioxidant response, which was associated with GR activation in goat liver within the 5-week experimental period.
Ethical Approval
The protocol was approved by the Animal Care Committee on the Ethics of Animal Experiments of Institute of Subtropical Agriculture (Permit No. 20170712).
Supplemental Material
Download MS Word (167.2 KB)Acknowledgments
The authors thank all staff from the Laboratory of Animal Production.
Disclosure statement
We certify that there is no conflict of interest with any financial organisation regarding the material presented in the manuscript.
Additional information
Funding
References
- Abaker JA, Xu TL, Jin D, Chang GJ, Zhang K, Shen XZ. 2017. Lipopolysaccharide derived from the digestive tract provokes oxidative stress in the liver of dairy cows fed a high-grain diet. J Dairy Sci. 100(1):666–678.
- Abdel-Salam OME, Salem NA, Hussein JS. 2012. Effect of aspartame on oxidative stress and monoamine neurotransmitter levels in lipopolysaccharide-treated mice. Neurotox Res. 21(3):245–255.
- Aditya S, Humer E, Pourazad P, Khiaosa-Ard R, Zebeli Q. 2018. Metabolic and stress responses in dairy cows fed a concentrate-rich diet and submitted to intramammary lipopolysaccharide challenge. Animal. 12(4):741–749.
- Alam MM, Okazaki K, Nguyen LTT, Ota N, Kitamura H, Murakami S, Shima H, Igarashi K, Sekine H, Motohashi H. 2017. Glucocorticoid receptor signaling represses the antioxidant response by inhibiting histone acetylation mediated by the transcriptional activator NRF2. J Biol Chem. 292(18):7519–7530.
- Alderton WK, Cooper CE, Knowles RG. 2001. Nitric oxide synthases: structure, function and inhibition. Biochem J. 357(Pt 3):593–615.
- AOAC. 2005. Official methods of analysis. Association of Official Analytical Chemists Publ., 18th ed. Arlington: AOAC.
- Ayroldi E, Cannarile L, Migliorati G, Nocentini G, Delfino DV, Riccardi C. 2012. Mechanisms of the anti-inflammatory effects of glucocorticoids: genomic and nongenomic interference with MAPK signaling pathways. Faseb J. 26(12):4805–4820.
- Chen J, Wang F, Zhou X, Cao Y, Li Y, Li C. 2017. Bama miniature pigs' liver possess great heat tolerance through upregulation of NRF2-mediated antioxidative enzymes. J Therm Biol. 67:15–21.
- Chung KF, Marwick JA. 2010. Molecular mechanisms of oxidative stress in airways and lungs with reference to asthma and chronic obstructive pulmonary disease. Ann N Y Acad Sci. 1203:85–91.
- Copple IM, Goldring CE, Kitteringham NR, Park BK. 2008. The NRF2-Keap1 defence pathway: role in protection against drug-induced toxicity. Toxicology. 246(1):24–33.
- Djikic D, Budec M, Vranjes-Djuric S, Todorovic V, Drndarevic N, Vignjevic S, Mitrovic O. 2012. Ethanol and nitric oxide modulate expression of glucocorticoid receptor in the rat adrenal cortex. Pharmacol Rep. 64(4):896–901.
- Dong H, Wang S, Jia Y, Ni Y, Zhang Y, Zhuang S, Shen X, Zhao R. 2013. Long-term effects of subacute ruminal acidosis (SARA) on milk quality and hepatic gene expression in lactating goats fed a high-concentrate diet. PLoS One. 8(12):e82850.
- Duanmu Y, Cong R, Tao S, Tian J, Dong H, Zhang Y, Ni Y, Zhao R. 2016. Comparative proteomic analysis of the effects of high-concentrate diet on the hepatic metabolism and inflammatory response in lactating dairy goats. J Anim Sci Biotechnol. 7:5.
- Fischer LR, Li Y, Asress SA, Jones DP, Glass JD. 2012. Absence of SOD1 leads to oxidative stress in peripheral nerve and causes a progressive distal motor axonopathy. Exp Neurol. 233(1):163–171.
- Gessner DK, Schlegel G, Keller J, Schwarz FJ, Ringseis R, Eder K. 2013. Expression of target genes of nuclear factor E2-related factor 2 in the liver of dairy cows in the transition period and at different stages of lactation. J Dairy Sci. 96(2):1038–1043.
- Gupta S, Kumar H, Soni J. 2005. Effect of vitamin E and selenium supplementation on concentrations of plasma cortisol and erythrocyte lipid peroxides and the incidence of retained fetal membranes in crossbred dairy cattle. Theriogenology. 64(6):1273–1286.
- Hakim A, Barnes PJ, Adcock IM, Usmani OS. 2013. Importin-7 mediates glucocorticoid receptor nuclear import and is impaired by oxidative stress, leading to glucocorticoid insensitivity. FASEB J. 27(11):4510–4519.
- Huang XS, Chen HP, Yu HH, Yan YF, Liao ZP, Huang QR. 2014. NRF2-dependent upregulation of antioxidative enzymes: a novel pathway for hypoxic preconditioning-mediated delayed cardioprotection. Mol Cell Biochem. 385(1-2):33–41.
- Jia YY, Wang SQ, Ni YD, Zhang YS, Zhuang S, Shen XZ. 2014. High concentrate-induced subacute ruminal acidosis (SARA) increases plasma acute phase proteins (APPs) and cortisol in goats. Animal. 8(9):1433–1438.
- Ki SH, Cho IJ, Choi DW, Kim SG. 2005. Glucocorticoid receptor (GR)-associated SMRT binding to C/EBPbeta TAD and NRF2 Neh4/5: role of SMRT recruited to GR in GSTA2 gene repression. Mol Cell Biol. 25(10):4150–4165.
- Kratschmar DV, Calabrese D, Walsh J, Lister A, Birk J, Appenzeller-Herzog C, Moulin P, Goldring CE, Odermatt A. 2012. Suppression of the NRF2-dependent antioxidant response by glucocorticoids and 11β-HSD1-mediated glucocorticoid activation in hepatic cells. PLoS One. 7(5):e36774.
- Laven RA, Peters AR. 1996. Bovine retained placenta: aetiology, pathogenesis and economic loss. Vet Rec. 139(19):465–471.
- Liu LY, Zeng M, Xie CM, Gao JH, Yan YS, Lu GF, Wang H, He YP. 2008. Oxidative stress status in patients with chronic obstructive pulmonary disease and its relation to glucocorticoid receptor levels. Nan Fang Yi Ke Da Xue Xue Bao. 28(6):992–996.
- Livak KJ, Schmittgen TD. 2001. Analysis of relative gene expression data using real-time quantitative PCR and the 2(-Delta Delta C(T)) method. Methods. 25(4):402–408.
- Matthews JG, Ito K, Barnes PJ, Adcock IM. 2004. Defective glucocorticoid receptor nuclear translocation and altered histone acetylation patterns in glucocorticoid-resistant patients. J Allergy Clin Immunol. 113(6):1100–1108.
- Mian OY, Khattab MH, Hedayati M, Coulter J, Abubaker-Sharif B, Schwaninger JM, Veeraswamy RK, Brooks JD, Hopkins L, Shinohara DB, et al. 2016. GSTP1 Loss results in accumulation of oxidative DNA base damage and promotes prostate cancer cell survival following exposure to protracted oxidative stress. Prostate. 76(2):199–206.
- Minton JE. 1994. Function of the hypothalamic-pituitary-adrenal axis and the sympathetic nervous system in models of acute stress in domestic farm animals. J Anim Sci. 72(7):1891–1898.
- Muthusamy VR, Kannan S, Sadhaasivam K, Gounder SS, Davidson CJ, Boeheme C, Hoidal JR, Wang L, Rajasekaran NS. 2012. Acute exercise stress activates NRF2/ARE signaling and promotes antioxidant mechanisms in the myocardium. Free Radic Biol Med. 52(2):366–376.
- Prunet P, Sturm A, Milla S. 2006. Multiple corticosteroid receptors in fish: from old ideas to new concepts. Gen Comp Endocrinol. 147(1):17–23.
- Savic-Radojevic A, Djukic T, Simic T, Pljesa-Ercegovac M, Dragicevic D, Pekmezovic T, Cekerevac M, Santric V, Matic M. 2013. GSTM1-null and GSTA1-low activity genotypes are associated with enhanced oxidative damage in bladder cancer. Redox Rep. 18(1):1–7.
- Sgorlon S, Stradaioli G, Gabai G, Stefanon B. 2008. Variation of starch and fat in the diet affects metabolic status and oxidative stress in ewes. Small Ruminant Res. 74(1-3):123–129.
- Singh AK, Haldar C. 2016. Melatonin modulates glucocorticoid receptor mediated inhibition of antioxidant response and apoptosis in peripheral blood mononuclear cells. Mol Cell Endocrinol. 436:59–67.
- Skuza G, Szymańska M, Budziszewska B, Abate C, Berardi F. 2011. Effects of PB190 and PB212, new σ receptor ligands, on glucocorticoid receptor-mediated gene transcription in LMCAT cells. Pharmacol Rep. 63(6):1564–1568.
- Spolarics Z. 1996. Endotoxin stimulates gene expression of ROS-eliminating pathways in rat hepatic endothelial and Kupffer cells. Am J Physiol. 270(4 Pt 1):G660–666.
- Stahn C, Buttgereit F. 2008. Genomic and nongenomic effects of glucocorticoids. Nat Clin Pract Rheumatol. 4(10):525–533.
- Taira M, Sasaki M, Kimura S, Araki Y. 2008. Dose-dependent effects of Ni (II) ions on production of three inflammatory cytokines (TNF-alpha, IL-1beta and IL-6), superoxide dismutase (SOD) and free radical NO by murine macrophage-like RAW264 cells with or without LPS-stimulation. J Mater Sci Mater Med. 19(5):2173–2178.
- Tao S, Han Z, Tian J, Cong R, Duanmu Y, Dong H, Ni Y, Zhao R. 2016. Downregulation of prostaglandin E2 is involved in hindgut mucosal damage in lactating goats fed a high-concentrate diet. Exp Physiol. 101(2):272–281.
- Tao S, Tian J, Cong R, Sun L, Duanmu Y, Dong H, Ni Y, Zhao R. 2015. Activation of cellular apoptosis in the caecal epithelium is associated with increased oxidative reactions in lactating goats after feeding a high-concentrate diet. Exp Physiol. 100(3):278–287.
- Tao S, Duanmu Y, Dong H, Ni Y, Chen J, Shen X, Zhao R. 2014. High concentrate diet induced mucosal injuries by enhancing epithelial apoptosis and inflammatory response in the hindgut of goats. PLoS One. 9(10):e111596.
- Trevisan M, Browne R, Ram M, Muti P, Freudenheim J, Carosella AM, Armstrong D. 2001. Correlates of markers of oxidative status in the general population. Am J Epidemiol. 154(4):348–356.
- Van Soest PJ, Robertson JB, Lewis BA. 1991. Methods fordietary fiber, neutral detergent fiber, and non-starch polysaccharides in relation to animal nutrition. J. Dairy Sci. 74(10):3583–3597.
- Wang KJ, Zheng MG, Ren A, Zhou CH, Yan QX, Tan ZL, Zhang PZ, Yi KL. 2019. Effects of high rice diet on growth performance, nutrients apparent digestibility, nitrogen metabolism, blood parameters and rumen fermentation in growing goats. Kafkas Univ Vet Fak Derg. 25(6):749–755.
- Wei N, Yu Y, Schmidt T, Stanford C, Hong L. 2013. Effects of glucocorticoid receptor antagonist, RU486, on the proliferative and differentiation capabilities of bone marrow mesenchymal stromal cells in ovariectomized rats. J Orthop Res. 31(5):760–767.
- Xiang P, He RW, Han YH, Sun HJ, Cui XY, Ma LQ. 2016. Mechanisms of housedust-induced toxicity in primary human corneal epithelial cells: oxidative stress, proinflammatory response and mitochondrial dysfunction . Environ Int. 89-90:30–37.
- Yamaji M, Tsutamoto T, Kawahara C, Nishiyama K, Yamamoto T, Fujii M, Horie M. 2009. Serum cortisol as a useful predictor of cardiac events in patients with chronic heart failure: the impact of oxidative stress. Circ Heart Fail. 2(6):608–615.
- Yang T, Li X, Zhu W, Chen C, Sun Z, Tan Z, Kang J. 2014. Alteration of antioxidant enzymes and associated genes induced by grape seed extracts in the primary muscle cells of goats in vitro. PLoS One. 9(9):e107670.
- Zebeli Q, Ametaj BN. 2009. Relationships between rumen lipopolysaccharide and mediators of inflammatory response with milk fat production and efficiency in dairy cows. J Dairy Sci. 92(8):3800–3809.
- Zhang HQ, Davies KJA, Forman HJ. 2015. Oxidative stress response and NRF2 signaling in aging. Free Radic Biol Med. 88(Pt B):314–336.
- Zhang N, Diao Y, Hua RR, Wang J, Han S, Li JF, Yin Y. 2017. Nitric oxide-mediated pathways and its role in the degenerative diseases. Front Biosci. 22:824–834.
- Zheng GX, Lin JT, Zheng WH, Cao J, Zhao ZJ. 2015. Energy intake, oxidative stress and antioxidant in mice during lactation. Dongwuxue Yanjiu. 36 (2):95–102.