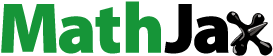
Abstract
This experiment was conducted to evaluate and compare the effects of a dietary inclusion with Aspergillus oryzae and Saccharomyces cerevisiae on productive performance, egg quality, intestinal morphology, and SGLT1 gene expression in laying Japanese quail. A total of 320 laying quails aged 47-day-old were randomly allocated to 8 treatments with 4 replicates of 10 quails each, for 7 weeks. The experimental diets consisted of a basal corn-soybean meal or control, control diet + 50 mg/kg tetracycline, control diet + 100 and 200 mg/kg A. oryzae, control diet + 100 and 200 mg/kg S. cerevisiae, control diet + 100 and 200 mg/kg of the combination of both A. oryzae and S. cerevisiae at equal proportions. At the end of the experiment, 4 quails per treatment were slaughtered, and tissue samples from the jejunum (1.5 cm in length) were removed for intestinal morphology measurements. Samples from the midsegment of the jejunum (150–200 mg) were taken to evaluate the gene expression of sodium-glucose cotransporter (SGLT1). The dietary inclusion of S. cerevisiae at 200 mg/kg significantly improved the feed conversion ratio (FCR) and egg production and reduced the feed intake (FI) (p < .001). The greatest shell thickness values were found in those fed on diets containing S. cerevisiae at 200 mg/kg. The quails fed on diets containing the combination of both probiotics at 100 mg/kg showed significantly higher egg weight (p < .001). Shell percent and yolk index increased significantly in quails fed on S. cerevisiae-supplemented diets at 100 mg/kg (p < .05). The villus height in the S. cerevisiae-supplemented group (200 mg/kg) was significantly higher than other groups (p < .05). The villus width was significantly higher in quails given 200 mg/kg A. oryzae compared to those received 100 mg/kg A. oryzae or 200 mg/kg S. cerevisiae (p < .05). Supplementation of S. cerevisiae at both tested levels resulted in significantly higher SGLT1 gene expression in the jejunum (p < .05). In conclusion, the results of this experiment demonstrated that among the two probiotics, S. cerevisiae at the level of 200 mg/kg as a functional feed additive has a better potential to improve productive performance, intestinal morphology, and SGLT1 gene expression of laying Japanese quails.
Supplementation of diet with 200 mg/kg S. cerevisiae improved egg production, shell thickness, and FCR.
The addition of 200 mg/kg S. cerevisiae to the diets improved the gut development by enhancing the villus height.
Inclusion of diet with S. cerevisiae at both levels upregulated the gene expression of SGLT1.
HIGHLIGHTS
Introduction
The industrial production of poultry eggs strongly contributes to meet the growing demand of protein worldwide. Despite the small size (one chicken egg is equal to 5 or 6 quail eggs), quail eggs can be consumed like chicken eggs to meet the protein requirements. They exhibit better nutritional value due to richer fat, protein, minerals (potassium, zinc, and iron), and vitamins compared to chicken eggs. In addition, quail eggs are generally richer in cholesterol than chicken eggs: this is mainly attributable to the HDL level, the so called good cholesterol (Narinc et al. Citation2013; Tolik et al. Citation2014). In commercial egg farming, feed additives are often used to improve health status, growth of chickens and efficiency of production (Dhama et al. Citation2014). Nevertheless, the use of antibiotics in animal production has been banned and replaced by efficient feed additives such as prebiotics and probiotics, which stimulate growth and favourably affect the immune response (Al-Khalaifah Citation2018). Probiotics are defined as either single or blend of live microorganisms which beneficially promote the health of host animal by production of special metabolites (hydrogen peroxide, short organic fatty acids, and metabolites possessing antimicrobial activity), competitive binding to receptors, and immunostimulatory effects (Madsen et al. Citation2001; Sherman et al. Citation2009). The microorganisms most commonly used as probiotic include several colonising species of Streptococcus, Lactobacillus, Clostridium, Bacillus, Enterococcus, and E. coli, while non-colonising fungal and yeast probiotics which are traditionally used as probiotics include Saccharomyces cerevisiae (S. cerevisiae) and Aspergillus oryzae (A. oryzae) (Chuang et al. Citation2019). S. cerevisiae provides a good source of high-quality protein and B vitamins. In poultry production, the yeast extract is suggested to be the most effective non-antibiotic alternative to overcome pathogenic bacteria (Huff et al. Citation2010). Previous research has indicated that feeding broilers on diets containing S. cerevisiae powder at 0.5% to broilers from 1 to 42 days of age, improve the productive performance, reduce the number of E. coli, and increase the villi height in the intestine (Teng et al. Citation2016). In addition, substantial enzyme secretion capabilities such as xylanases, cellulases, and proteases has been reported for S. cerevisiae (Strauss et al. Citation2001). A. oryzae, generally recognised as safe probiotic, can be used as a probiotic for fermentation. It was also reported that the fermentation of cassava pulp with A. oryzae can improve the CP content and feeding of broilers with 160 g/kg of fermented cassava pulp from 1 to 42 days of age resulted in higher digestive enzymes that are involved in digestion (Khempaka et al. Citation2014). A. oryzae remove actively the oxygen of the fermentation media and provide a favourable environment for the growth and proliferation of Bacillus subtilis, Lactobacillus plantarum, and Lactobacillus acidophilus, and in turn, develop a protective ecosystem against the pathogenic organisms (Jazi et al. Citation2017). The beneficial effects of probiotics in laying hens on egg production percentage, egg weight, egg mass, and serum, and yolk cholesterol concentration have been previously reported (Nahashon et al. Citation1994; Abdulrahim et al. Citation1996; Jin et al. Citation1997). Han et al. (Citation1999) evaluated the effects of dietary A. oryzae on the metabolizability of macronutrients in laying hens and concluded that the fungus increased nutrients digestibility. However, except for extra-large eggs, no differences were found in egg production, egg weight, and shell weight (Han et al. Citation1999). Similarly, Tomaszewska et al. (Citation2018) reported that dietary supplementation with S. cerevisiae lead to positive impacts on the absorptive surface area of the jejunum and improve digestive efficiency in laying quails. Similarly, Gao et al. (Citation2008) reported that dietary inclusion of yeast culture increased the villus height and villus height to crypt depth ratio in the duodenum, and jejunum of broiler chickens. Feng et al. (Citation2007) indicated that dietary supplementation of A. oryzae fermented soybean meal in place of soybean meal can reduce the crypt depth in jejunum and enhance the villus height in both jejunum and duodenum of broiler chickens. The uptake of nutrients from lumen into the intestinal epithelial cells is regulated by nutrient transporters (Kaminski and Wong Citation2018). It has been reported that the probiotic supplementation affected the gene expression of some nutrient transporters in the enterocytes (Buts et al. Citation1999; Silveira et al. Citation2018). Among these transporters, the sodium-glucose cotransporter (SGLT1) is the main glucose transporter in the brush border membrane (Wright Citation2013). The objective of the present trial was to evaluate the effects of A. oryzae and S. cerevisiae individually or synergistically as alternatives to antibiotics on performance, egg quality, intestinal morphology, and mRNA expression of SGLT1 in laying Japanese quails.
Materials and methods
Birds, husbandry and experimental treatments
A total of 320 laying Japanese quails (Coturnix coturnix japonica) at 37 days of age with the same initial weight were purchased from a local hatchery and fed a commercial diet for 10 days. They were then randomly allocated to eight treatment groups with 4 replicates of 10 quails per each replicate based on a completely randomised design. Quails were individually housed in battery wire cages (50 × 30 × 50 cm3) equipped with individual feeder and nipple drinker. Briefly, quails were fed on eight experimental diets including a basal control diet (containing no supplement), control diet + 50 mg/kg tetracycline, control diet + 100 and 200 mg/kg A. oryzae, control diet + 100 and 200 mg/kg S. cerevisiae, and control diet + 100 and 200 mg/kg mixture of A. oryzae and S. cerevisiae at equal proportions, respectively. A. oryzae and S. cerevisiae were obtained from the Persian Type Culture Collection (PTCC) of the Iranian Research Organisation for Science and Technology. Feed and water were provided ad libitum during the 7-week experimental period. The quails were exposed to a continuous lighting regimen of 16 h. All diets were isocaloric and isonitrogenous and formulated based on corn and soybean meal to meet or exceed the laying Japanese quail requirements, according to NRC (Citation1994) (Table ). All quails were kept under the same care and management conditions during the entire period of the experiment. The Animal Ethics Committee of Saravan Higher Education Complex approved all the animal protocols used in the current experiment.
Table 1. Ingredients and chemical composition (%) of the basal diet.
Productive performance
Egg production (number and weight) was recorded daily, and then hen-day egg production was calculated. Feed intake was recorded at weekly intervals on the cage basis. Feed conversion ratio (FCR) was calculated as the ratio of grams of feed consumed per grams of egg produced. Egg mass was calculated as the multiplying of average egg weight by the number of eggs laid. Defective eggs, including cracked, broken, shell-less eggs, and other abnormal eggs, were recorded as they laid.
Egg quality traits
During a 4-day period in the last week of the experiment, eggs from each replicate were collected (1280 in total). Thereafter, 3 eggs per each replicate were randomly selected to determine external and internal egg quality parameters. Egg length, and egg width, in mm, were measured using a digital micrometer with measuring accuracy of ±1 μm (series 500, Mitutoyo, Tokyo, Japan). Egg shape index was subsequently calculated: egg shape index = egg width/egg length ×100) (Anderson et al. Citation2004). To assess the internal egg quality characteristics, the eggs were broken on a flat glass surface. The egg yolks were separated from the albumen and rolled on a wet paper towel to remove residual albumen and weighed. The yolk index was calculated as yolk index = yolk height/yolk diameter (mm) according to Funk (Citation1958). The yolk colour was evaluated using the Roche colour fan (Hoffman-La Roche Ltd., Basel, Switzerland) where, 1 is the light pale and 15 the dark orange. The shells were carefully separated, washed thoroughly, dried at room temperature for 24 h and then weighed. Shell thickness was measured at three different points of the egg (sharp end, equator and, air cell) using a digital micrometer (series 500, Mitutoyo, Tokyo, Japan). The average value of these measurements was the egg shell thickness. Albumen weight was determined by subtracting yolk and shell weights from the whole egg weight. Haugh unit (HU) was calculated as HU= 100 log (albumen height − 1.7 egg weight 0.37 + 7.57), according to Sari et al. (Citation2016). Relative weights for shell, albumen, and yolk were expressed as a percentage of egg weight.
Intestinal histomorphology, serum metabolites and sampling
At the end of the experiment, one bird per replicate was randomly selected, fasted for 12 h, and weighed. The quails were electrically stunned and then bled from the jugular vein. The blood samples were centrifuged at 3500 × g for 10 min at 4°C and stored at −20°C until analysis of samples for triglyceride, LDL, HDL, and cholesterol. The sera samples were analysed using an automated blood analyser (Random Access Analyser A15, Biosystem Corp, Spain). Thereafter, the selected birds were killed by cervical dislocation. The intestine was collected, and tissue samples from jejunum were removed (1.5 cm in length), simultaneously flushed with cold sterile saline (PH 7.2), and fixed in 10% formalin for further analyses. Tissue samples were then gradually dehydrated, sectioned into 6 mm thickness, embedded in paraffin and, subsequently cut with a 5 μm thickness and placed on slides. The tissues were stained with hematoxylin-eosin and morphological indices, including villus height, villus width, and crypt depth determined using an optical microscope (Olympus BX41TF, Tokyo, Japan) and image analysis system (Olysia Soft Imaging System, Germany). Villus surface area was determined as [(2π) × (villus width/2) × (villus height)] (Sakamoto et al. Citation2000). To evaluate the gene expression of SGLT1, samples from the midsegment of the jejunum (150–200 mg) were taken, rinsed in ice-cold PBS, minced, placed into RNAlater (Qiagen, Hilden, Germany), and stored at −80 °C for subsequent mRNA extraction.
Total RNA extraction and reverse transcription
The total RNA was extracted using a commercial RNA extraction kit (RNX-plus Kit, SinaClon Iran)) according to the manufacturer's instructions. The RNA quality and quantity were determined using a NanoDrop 2000 spectrophotometer (Thermo Fisher Scientific, Waltham, MA, USA) set at 260–280 nm wavelength. Extracted RNA kept at −80 °C until used for cDNA synthesis. Subsequently, the cDNA was synthesised from 1 to 10 micrograms of total RNA in the presence of a 2-steps RT-PCR kit (Cat. No. RTPL12, Vivantis Technologies) according to the manufacturer’s protocol.
Quantitative real-time PCR
A quantitative real-time PCR (qRT-PCR) was performed using a Real-Time PCR System (Applied Biosystems) on 48-well plate format. Each quantitative PCR (qPCR) reaction was performed with forward and reverse primers for either the target genes or the housekeeping gene, cDNA, SYBR Green Master Mix (Applied Biosystems). Each reaction was performed in duplicate, and no template control was included in each run. The primers included SGLT1 and β-actin as the internal control. The primer of SGLT1 used for real-time PCR was that reported in (Santana et al. Citation2019). The real-time PCR reaction was run under the following settings: 95 °C for 10 min, 40 cycles of 95 °C for 15 s, 60 °C for 30 s, and 72 °C for 40 s, followed by 1 cycle of final extension at 72 °C for 10 min; the melting curve was obtained over the range 60–95 °C. The sequences of the primers used in the present study are listed in . The average mRNA expression of the target genes relative to the β-actin as endogenous control was calculated using the 2−ΔΔCt method (Livak and Schmittgen Citation2001).
Table 2. Primer sequences used for the quantitative real-time PCR.
Statistical analysis
Data were statistically analysed using the general linear model (GLM) procedure of SAS (SAS Institute, Citation2003) in a completely randomised design. The experimental unit was the cage of 10 quails. Differences between treatments were determined using Tukey’s test and considered significant at p ≤ .05. The following statistical model was used.
where Yij is the observation, μ is the general mean, Ti is the effect of the treatment, μ is the general mean, eij is the effect of random error.
Results
Productive performance
The effects of dietary treatments on quail's productive performance are summarised in Table . The highest value of egg production was recorded in the group that received S. cerevisiae at the level of 200 mg/kg, while the lowest value was obtained in quails receiving the dietary supplementation with 200 mg/kg mixture of A. oryzae and S. cerevisiae at equal proportions (90.2 and 85.8%, respectively, p < .001). Meanwhile, the differences were significant when compared with those receiving control, A. oryzae-supplemented, and a mixture of A. oryzae and S. cerevisiae-supplemented groups at 100 and 200 mg/kg (90.2 vs. 87.7, 86.7, 86.6, 86.4%, and 85.8% respectively, p < .001). Also, no statistically significant differences were found among laying quails fed both tested levels of S. cerevisiae (p > .05). Concerning egg weight, the highest value was recorded for quails fed on diets included with the mixture of A. oryzae and S. cerevisiae at 100 mg/kg, but the lowest egg weight value was recorded for those fed on A. oryzae-supplemented diets at 200 mg/kg (12.04 vs. 11.71 g, p < .001). The differences were significant as compared to the other groups except for the A. oryzae-supplemented group at 100 mg/kg. Regarding feed intake, the highest value was noted for quails fed a control diet, while the lowest value was observed in those fed on S. cerevisiae-supplemented diets at 200 mg/kg (30.55 vs. 29.12 g, p < .001). The reduction of feed intake in the S. cerevisiae-supplemented group at 200 mg/kg was statistically significant as compared to other groups except those receiving diets supplemented with 100 mg/kg of S. cerevisiae. Results revealed that the quails fed diets supplemented with 200 mg/kg S. cerevisiae had the lowest FCR values (g feed/g egg) followed by S. cerevisiae-supplemented group at 100 mg/kg, and tetracycline-supplemented group (2.77, 2.86, and 2.91, respectively). In contrast, the quails fed on control diets showed the highest FCR values followed by those receiving diets containing the mixture of probiotics at 200 and 100 mg/kg (3.02 and 2.97, respectively). Concerning egg mass, the higher value was noted in quails that given diets containing S. cerevisiae at 200 mg/kg, which was statistically significant compared to the A. oryzae-supplemented group and those receiving the mixture of two probiotics at 200 mg/kg (10.66 vs. 10.15 and 10.16, respectively, p < .001).
Table 3. Effect of A. oryzae and S. cerevisiae dietary inclusion on the productive performance of laying Japanese quails.
Egg quality parameters
As shown in Table , the greatest value of shell thickness was noted in S. cerevisiae-supplemented group at 100 mg/kg and the lowest in the tetracycline-supplemented group (0.190 vs. 0.170 mm, respectively). The shell thickness was significantly influenced by S. cerevisiae, particularly at the level of 200 mg/kg, which was significantly different compared to other groups except the group receiving S. cerevisiae-supplemented diets at 100 mg/kg and those given the mixture of probiotics at 200 mg/kg (p < .001). Regarding shell weight, no significant differences were observed between dietary treatments during the experimental period (p > .05). The greatest shell percent was recorded for quails fed on diets containing 100 mg/kg S. cerevisiae followed by a mixture-supplemented group at 100 mg/kg, and those given control diets (9.63, 9.44, and 9.36%, respectively), while the lowest shell percent was obtained in the mixture-supplemented group at 200 mg/kg, which was significantly different compared to the control group (7.24 vs. 9.36%, p < .01). All yolk characteristics (yolk weight, yolk percent, and yolk colour) were not significantly influenced by dietary treatments (p > .05), except for yolk index that was the greatest in A. oryzae-supplemented group (100 mg), and significantly different when compared to control group (p < .05). No significant differences in albumen percentage, and egg shape index (p > .05) were found among dietary treatments. Data on the Haugh unit showed that the quails fed on A. oryzae-supplemented diets had the highest value, and those fed on tetracycline-supplemented diets revealed the lowest value among the groups (88.5 vs. 86.0, p < .05). However, no statistically significant differences were found when compared to the control group (88.5 and 86.0 vs. 86.2, p > .05).
Table 4. Effect of A. oryzae and S. cerevisiae dietary inclusion on egg quality traits of laying Japanese quails.
Intestinal histomorphology
As shown in Table , villus height in the S. cerevisiae-supplemented group (200 mg/kg) was greatest than other groups while the lowest value was found in the mixture-supplemented group at 200 mg/kg (553.25 vs. 395, p < .05). Also, the differences were statistically significant compared to all other treatments except those fed on 100 mg/kg S. cerevisiae, and mixture of probiotics (p < .05). The quails fed on diets containing 200 mg/kg A. oryzae showed the highest value of villus width. On the other hand, the lowest value was obtained in those fed on tetracycline-supplemented diets (146 vs. 108.75, p < .05). The villus width was significantly higher in quails given 200 mg/kg A. oryzae compared to those receiving 100 mg/kg A. oryzae or 200 mg/kg S. cerevisiae (p < .005), but did not differ from the control group (p > .05). No statistically significant effects were found on crypt depth, villus surface area, and villus height/crypt depth ratio (p > .05). However, a near-significant trend for villus surface area (p = .07) and a substantial trend towards significant (p = .067) for villus height/crypt depth ratio were found.
Table 5. Effect of A. oryzae and S. cerevisiae dietary inclusion on jejunum morphology of laying Japanese quails.
Serum metabolites
According to the results illustrated in Table , dietary treatments did not significantly affect blood serum parameters (p > .05). Dietary supplementation of A. oryzae, S. cerevisiae, or a mixture of them did not affect triglyceride, LDL, HDL, and cholesterol compared to control or tetracycline-supplemented group (p > .05).
Table 6. Effect of A. oryzae and S. cerevisiae dietary inclusion on blood serum parameters of laying Japanese quails.
SGLT1 gene expression
The fold change gene expression of SGLT1 is presented in Figure . Results showed that dietary inclusion of S. cerevisiae at two levels (i.e. 100 and 200 mg/kg) up-regulated the expression of SGLT1 in the jejunum compared to control and other dietary treatments (p = .047).
Discussion
This feeding experiment was designed to evaluate the effects of two probiotics A. oryzae, and S. cerevisiae, in comparison with tetracycline antibiotic as beneficial agents on productive performance, intestinal morphology, and SGLT1 gene expression in laying Japanese quails. In agreement with the present study, Yalçın et al. (Citation2010) found that dietary supplementation with yeast autolysate (S. cerevisiae) at 2, 3, and 4 g/kg in laying hens increased hen-day egg production, egg weight, and improved the feed efficiency. Regarding the FI, similar results were noted by Özsoy et al. (Citation2018), who reported that the laying hens fed with the diets containing S. cerevisiae at the level of 0.2% revealed the lowest feed intake. Contrary to the findings of the present study, Yalçın et al. (Citation2009) reported that except for egg weight, other performance parameters were not significantly influenced by dietary inclusion of dried baker’s yeast (S. cerevisiae at the levels of 4–20%) in laying quails. This divergence may be the result of the differences in the birds age, doses, feeding trial duration, and strains of yeast. In the present experiment, the improvement of productive performance traits by dietary inclusion of S. cerevisiae in laying quails could be related to the influence on the gut health that led to better FCR. It has been reported that dietary supplementation of S. cerevisiae in the animal diets increased the activities of ɑ-amylase, trypsin, and chymotrypsin in duodenal chyme that may result in higher protein digestibility and nutrient utilisation (Ahiwe et al. Citation2020). Moreover, yeast is a rich source of nutrients and it may act as an efficient substrate for beneficial microorganisms, which leads to the increase in proliferation of these gut microbiota (Zhang et al. Citation2005). It should be noted that intestinal bacteria that exist in the gastrointestinal tract play a key role in nutrients digestion and absorption and may consequently influence the animals FCR (Zhang et al. Citation2005). It has been found that mannan oligosaccharides derived from the yeast cell wall reducing the pathogenic bacterial flora in the digestive gut, so lead to the efficient diversion of dietary nutrients towards production in birds fed yeast supplement, which in turn might improve egg production in breeders and layers (Spring et al. Citation2000). It appears that the improvements of egg production and egg weight in the present experiment could be related to the promoting potency of probiotics on metabolic processes of nutrients digestion and utilisation (Yeo and Kim Citation1997). It is well illustrated that probiotics can alter the gastrointestinal flora by reducing the pH, increasing the activity of intestinal enzymes, and nutrients digestibility (Yang et al. Citation2005). Inclusion of probiotics in the diets exert beneficial effects on nutrients availability in the digestive gut, improve the intestinal absorption of nutrients, and led to an effective reduction of feed consumption because laying birds have adequate amounts of nutrients for maintenance and production (Markowiak and Śliżewska Citation2018). The obtained results from our study regarding the beneficial effects of supplementary S. cerevisiae on egg shell thickness coincide with the findings of Elkaiaty et al. (Citation2019), who stated that the egg shell thickness values were significantly higher for laying hens fed on the diets containing 0.4 and 0.6% yeast (S. cerevisiae). The findings are consistent with a previous report of Lemos et al. (Citation2014) who showed that dietary inclusion of cell wall of S. cerevisiae at 0.15% in the diets of laying Japanese quails resulted in improvement of the shell thickness and percentage. Elkaiaty et al. (Citation2019) reported that egg shell thickness in the layers fed yeast-supplemented diets may be related to the enhancement of calcium absorption and retention. The positive impacts of the feeding of probiotic-supplemented diets on egg shell quality may be related to a favourable environment in the gastrointestinal tract, which may support the absorption of more nutrients (Panda et al. Citation2008). The improvement of shell quality in quails fed on yeast-supplemented diets, noted in the present experiment, confirms the hypothesis that the acidic environment developed in the digestive gut by lactic acid bacteria facilitates the ionisation and subsequent absorption of the minerals (Haddadin et al. Citation1996). The results of the present study concerning the egg yolk index are consistent with previous research by Yoruk et al. (Citation2004), who found that supplementation of probiotic (containing A. oryzae 7.98 × 109 cfu) during the late laying period in hens significantly improved the yolk index. The egg yolk index, defined as an index of the freshness of an egg, the ratio of yolk height over yolk diameter. The yolk index reduces as a consequence of egg deterioration (Khan et al. Citation2011). The increase in yolk index values is related to the insertion of yellow pigments in the yolk membrane between the lipid molecules, which might be related to the higher uptake of corn xanthophylls. A. oryzae can utilise the fibre and starch of the carbon source substances to generate energy for the growth of beneficial microbes and thereby influencing the egg yolk index (Gnanadesigan et al. Citation2014). The present findings provided evidence that the villus height in the jejunum increased in the S. cerevisiae-supplemented group (200 mg/kg). In agreement with the present observations, Rahimi et al. (Citation2019) found that cell wall preparations from S. cerevisiae IMW50 in broilers challenged with Salmonella and Campylobacter increased the villus height. Similar findings were found in a study by Tomaszewska et al. (Citation2018), where the villus height was significantly increased in laying Japanese quails receiving the S. cerevisiae-supplemented diets at the level of 1.5%. These alterations may be related to the fact that dietary yeast affects the local metabolism and increases the synthesis of short chain fatty acids (SCFAs), which in turn stimulate the proliferation of intestinal epithelial cells resulting in broader and longer villi (Tomaszewska et al. Citation2018). Also, improved intestinal alterations due to dietary probiotics are related to their ability to develop a better environment for beneficial microbes (Awad et al. Citation2010). Similarly, Shah et al. (Citation2019) reported that lactobacillus bacteria may increase the villus height via the digestion of carbohydrates, production of VFAs, and consequent nourishment of the intestinal villi. The higher values of villus height could result in a greater capacity for available nutrients (Jazi et al. Citation2018), which may be reflected by the improvement of the FCR values in the present experiment. Concerning the effects of A. oryzae on villus width, our findings are in agreement with the results of Mathivanan et al. (Citation2006), who reported that fermentation products of Aspergillus niger, one of the most common members of the genus Aspergillus, significantly increased the ileum villi width. Probiotics can occupy the surface of the intestinal wall resulting in the reduction of the pathogenic bacteria, and the birds fed probiotic-supplemented diets can develop changes in intestinal tissues, such as the increase in the number of cells in mitosis and nuclear size. Similar to the results of the present study, other investigations have found that the serum blood triglyceride, LDL, HDL, and cholesterol were not affected by S. cerevisiae or A. oryzae supplementation (Sohail et al. Citation2011; Jazi et al. Citation2018). The results demonstrated that A. oryzae, S. cerevisiae, or the combination of two probiotics up to 200 mg/kg in quails' diet had no significant impact on the physiological state, and the supplemental probiotics did not affect the ability of cholesterol transport from peripheral tissues to the liver. Regarding the effects of dietary S. cerevisiae on gene expression of SGLT1, these results are consistent with a previous report of Silveira et al. (Citation2018), who stated that the yeast culture increased the gene expression of SGLT1 in porcine jejunal explants. Similarly, Buts et al. (Citation1999) reported that the administration of S. boulardii by orogastric intubation at the level of 1 mg/g body weight per day for seven days increased twofold, the expression of SGLT1 in brush border membranes of resected rats. The SGLT1 transporter is responsible for Na+-dependent uptake of glucose from the intestinal lumen into mucosal epithelial cells (Kellett et al. Citation2008). It has been shown that the supplementary yeast provides the high amounts of polyamines, that are taken up via the polyamine transport system and act as signalling molecules. As a consequence, transcription, translation, and kinase activities are activated, thereby resulting in enhanced expression of digestive enzymes, SGLT1, and alkaline phosphatase in the intestinal mucosa (More and Vandenplas Citation2018). Polyamines play a vital role in the maturation of the glucose transporters (Glut2 and SGLT1) in the digestive gut during development (Wild et al. Citation2007). It has been found that the prominent role of polyamines is their potential to stimulate the eukaryotic translation initiation factor 5 A (EIF5A). Polyamines affect the activity of EIF5A through the post-translational alteration of a specific lysine residue. As a result of this modification, the active EIF5A can bind to ribosomes and initiate translation (Schuller et al. Citation2017). On the other hand, it has been reported that the control of pathogenic bacteria in the birds given supplementary probiotic may affect the expression of intestinal carriers (Santana et al. Citation2019). Previous studies found that microbial-elicited reactive oxygen species (ROS) as a second messenger may play a detrimental role in cell signalling, growth, motility, and differentiation of epithelial cells and thereby influence the expression of genes and absorptive capacity of the gastrointestinal tract (Jones et al. Citation2012).
Conclusions
Based on the above-mentioned findings, it was demonstrated that the inclusion of 200 mg/kg S. cerevisiae in the diets for laying Japanese quails could be a valid alternative to antibiotics as it improved egg production, shell thickness, FCR, gut development, and SGLT1 gene expression. Differently, the sole supplementation of A. oryzae as well as the simultaneous administration of A. oryzae and S. cerevisiae did not affect productive performance, intestinal morphology, and SGLT1 gene expression. Despite these encouraging findings, further research is needed to determine the exact mechanism of action of the S. cerevisiae, especially on the productive performance, egg quality traits, intestine morphology, and gene expression of nutrient transporters in laying Japanese quails.
Ethical approval
The Animal Ethics Committee of Saravan Higher Education Complex approved all the animal protocols used in the current experiment.
Disclosure statement
No potential conflict of interest was reported by the author(s).
References
- Abdulrahim SM, Haddadin SY, Hashlamoun EA, Robinson RK. 1996. The influence of Lactobacillus acidophilus and bacitracin on layer performance of chickens and cholesterol content of plasma and egg yolk. Br Poult Sci. 37(2):341–346.
- Ahiwe EU, Abdallh ME, Chang’a EP, Omede AA, Al-Qahtani M, Gausi H, Graham H, Iji PA. 2020. Influence of dietary supplementation of autolyzed whole yeast and yeast cell wall products on broiler chickens. Asian-Australas J Anim Sci. 33(4):579–587.
- Al-Khalaifah HS. 2018. Benefits of probiotics and/or prebiotics for antibiotic-reduced poultry. Poult Sci. 97(11):3807–3815.
- Anderson K, Tharrington J, Curtis P, Jones F. 2004. Shell characteristics of eggs from historic strains of single comb white leghorn chickens and the relationship of egg shape to shell strength. Int J Poultry Sci. 3(1):17–19.
- Awad WA, Ghareeb K, Bohm J. 2010. Effect of addition of a probiotic micro-organism to broiler diet on intestinal mucosal architecture and electrophysiological parameters. J Anim Physiol Anim Nutr. 94(4):486–494.
- Buts JP, De Keyser N, Marandi S, Hermans D, Sokal EM, Chae YH, Lambotte L, Chanteux H, Tulkens PM. 1999. Saccharomyces boulardii upgrades cellular adaptation after proximal enterectomy in rats. Gut. 45(1):89–96.
- Chuang WY, Lin WC, Hsieh YC, Huang CM, Chang SC, Lee TT. 2019. Evaluation of the combined use of Saccharomyces Cerevisiae and Aspergillus Oryzae with phytase fermentation products on growth, inflammatory, and intestinal morphology in Broilers. Animals. 9(12):1051.
- Dhama K, Tiwari R, Khan RU, Chakrabort S, Gopi M, Karthik K, Saminathan M, Desingu PA, Sunkara LT. 2014. Growth promoters and novel feed additives improving poultry production and health, bioactive principles and beneficial applications: the trends and advances-a review. Int J Pharmacol. 10(3):129–159.
- Elkaiaty AM, Badran AM, Bayoumi A, Eshera AA, El-Sayed O. 2019. Effect of dietary yeast supplementation on productive performance, eggshell quality and lipid profile of laying hens. Egypt Poult Sci J. 39(2):567–578.
- Feng J, Liu X, Xu ZR, Wang YZ, Liu JX. 2007. Effects of fermented soybean meal on digestive enzyme activities and intestinal morphology in broilers. Poult Sci. 86(6):1149–1154.
- Funk EM. 1958. Quality of eggs laid by caged layers. Missouri: University of Missouri, College of Agriculture, Agricultural Experiment Station.
- Gao J, Zhang HJ, Yu SH, Wu SG, Yoon I, Quigley J, Gao YP, Qi GH. 2008. Effects of yeast culture in broiler diets on performance and immunomodulatory functions. Poult Sci. 87(7):1377–1384.
- Gnanadesigan M, Isabella S, Saritha P, Ramkumar L, Manivannan N, Ravishankar R. 2014. Quality evaluation of egg composition and productivity of layers in EM (Effective Microorganisms) treatments: a field report. Egypt J Basic Appl Sci. 1(3–4):161–166.
- Haddadin MS, Abdulrahim SM, Hashlamoun EA, Robinson RK. 1996. The effect of Lactobacillus acidophilus on the production and chemical composition of hen’s eggs. Poult Sci. 75(4):491–494.
- Han S, Lee K, Lee B, Sung C. 1999. Effect of feeding Aspergillus oryzae culture on fecal microflora, egg qualities, and nutrient metabolizabilities in laying hens. Asian Australas J Anim Sci. 12(3):417–421.
- Huff GR, Huff WE, Farnell MB, Rath NC, Solis de Los Santos F, Donoghue AM. 2010. Bacterial clearance, heterophil function, and hematological parameters of transport-stressed turkey poults supplemented with dietary yeast extract. Poult Sci. 89(3):447–456.
- Jazi V, Ashayerizadeh A, Toghyani M, Shabani A, Tellez G, Toghyani M. 2018. Fermented soybean meal exhibits probiotic properties when included in Japanese quail diet in replacement of soybean meal. Poult Sci. 97(6):2113–2122.
- Jazi V, Boldaji F, Dastar B, Hashemi SR, Ashayerizadeh A. 2017. Effects of fermented cottonseed meal on the growth performance, gastrointestinal microflora population and small intestinal morphology in broiler chickens. Br Poult Sci. 58(4):402–408.
- Jin L, Ho Y, Abdullah N, Jalaludin S. 1997. Probiotics in poultry: modes of action. Worlds Poult Sci J. 53(4):351–368.
- Jones RM, Mercante JW, Neish AS. 2012. Reactive oxygen production induced by the gut microbiota: pharmacotherapeutic implications. Curr Med Chem. 19(10):1519–1529.
- Kaminski NA, Wong EA. 2018. Differential mRNA expression of nutrient transporters in male and female chickens. Poult Sci. 97(1):313–318.
- Kellett GL, Brot-Laroche E, Mace OJ, Leturque A. 2008. Sugar absorption in the intestine: the role of GLUT2. Annu Rev Nutr. 28:35–54.
- Khan SH, Atif M, Mukhtar N, Rehman A, Fareed G. 2011. Effects of supplementation of multi-enzyme and multi-species probiotic on production performance, egg quality, cholesterol level and immune system in laying hens. J Appl Anim Res. 39(4):386–398.
- Khempaka S, Thongkratok R, Okrathok S, Molee W. 2014. An evaluation of cassava pulp feedstuff fermented with A. oryzae, on growth performance, nutrient digestibility and carcass quality of broilers. J Poult Sci. 51 (1):71–79.
- Lemos MJd, Calixto LFL, Lima CARd, Reis TL, Rego RS, Nak SY, Aroucha RJN. 2014. Prebiotic levels in the diet on performance and egg quality of Japanese quail. Rev Bras Saúde Prod Anim. 15(3):613–625.
- Livak KJ, Schmittgen TD. 2001. Analysis of relative gene expression data using real-time quantitative PCR and the 2(-Delta Delta C(T)) Method. Methods. 25(4):402–408.
- Madsen K, Cornish A, Soper P, McKaigney C, Jijon H, Yachimec C, Doyle J, Jewell L, De Simone C. 2001. Probiotic bacteria enhance murine and human intestinal epithelial barrier function. Gastroenterol. 121(3):580–591.
- Markowiak P, Śliżewska K. 2018. The role of probiotics, prebiotics and synbiotics in animal nutrition. Gut Pathog. 10:21.
- Mathivanan R, Selvaraj P, Nanjappan K. 2006. Feeding of fermented soybean meal on broiler performance. Int J Poultry Sci. 5(9):868–872.
- More MI, Vandenplas Y. 2018. Saccharomyces boulardii CNCM I-745 improves intestinal enzyme function: a trophic effects review. Clin Med Insights Gastroenterol. 11:1179552217752679.
- Nahashon SN, Nakaue HS, Mirosh LW. 1994. Phytase activity, phosphorus and calcium retention, and performance of single comb White Leghorn layers fed diets containing two levels of available phosphorus and supplemented with direct-fed microbials. Poult Sci. 73(10):1552–1562.
- Narinc D, Aksoy T, Karaman E, Aygun A, Firat MZ, Uslu MK. 2013. Japanese quail meat quality: characteristics, heritabilities, and genetic correlations with some slaughter traits. Poult Sci. 92(7):1735–1744.
- NRC. 1994. National Research Council. Nutrient requirements of poultry. 9th revised ed. Washington, DC: Natl. Acad. Press.
- Özsoy B, Karadağoğlu Ö, Yakan A, Önk K, Çelik E, Şahin T. 2018. The role of yeast culture (Saccharomyces cerevisiae) on performance, egg yolk fatty acid composition, and fecal microflora of laying hens. R Bras Zootec. 47:e20170159.
- Panda AK, Rama Rao SS, Raju MV, Sharma SS. 2008. Effect of probiotic (Lactobacillus sporogenes) feeding on egg production and quality, yolk cholesterol and humoral immune response of White Leghorn layer breeders. J Sci Food Agric. 88(1):43–47.
- Rahimi S, Kathariou S, Fletcher O, Grimes JL. 2019. Effect of a direct-fed microbial and prebiotic on performance and intestinal histomorophology of turkey poults challenged with Salmonella and Campylobacter. Poult Sci. 98(12):6572–6578.
- Sakamoto K, Hirose H, Onizuka A, Hayashi M, Futamura N, Kawamura Y, Ezaki T. 2000. Quantitative study of changes in intestinal morphology and mucus gel on total parenteral nutrition in rats. J Surg Res. 94(2):99–106.
- Santana TP, Jesus JASd, Bastos MS, Nunes AS, Júnior GO, Nascimento CSd, Barbosa LT, Brito CO, Gasparino E, Del Vesco AP. 2019. Antioxidant activity and duodenum transporter gene expression in quail fed citric acid. SA J An Sci. 49(4):636–643.
- Sari M, Tilki M, Saatci M. 2016. Genetic parameters of egg quality traits in long-term pedigree recorded Japanese quail. Poult Sci. 95(8):1743–1749.
- SAS Institute. 2003. SAS 9.1. Cary (NC): SAS Institute.
- Schuller AP, Wu CC, Dever TE, Buskirk AR, Green R. 2017. eIF5A functions globally in translation elongation and termination. Mol Cell. 66(2):194–205.
- Shah M, Zaneb H, Masood S, Khan RU, Ashraf S, Sikandar A, Rehman HFU, Rehman HU. 2019. Effect of dietary supplementation of zinc and multi-microbe probiotic on growth traits and alteration of intestinal architecture in broiler. Probiotics Antimicro Prot. 11(3):931–937.
- Sherman PM, Ossa JC, Johnson-Henry K. 2009. Unraveling mechanisms of action of probiotics. Nutr Clin Pract. 24(1):10–14.
- Silveira H, Melo ADB, Bortoluzzi C, Costa LB, Rostagno MH, Schinckel AP, Garbossa CAP, Cantarelli VS. 2018. Feed additives can differentially modulate NF-κB (RelA/p65), IGF-1, GLUT2, and SGLT1 gene expression in porcine jejunal explants. Rev Bras de Zootec. 47:e20180105.
- Sohail MU, Rahman ZU, Ijaz A, Yousaf MS, Ashraf K, Yaqub T, Zaneb H, Anwar H, Rehman H. 2011. Single or combined effects of mannan-oligosaccharides and probiotic supplements on the total oxidants, total antioxidants, enzymatic antioxidants, liver enzymes, and serum trace minerals in cyclic heat-stressed broilers. Poult Sci. 90(11):2573–2577.
- Spring P, Wenk C, Dawson KA, Newman KE. 2000. The effects of dietary mannaoligosaccharides on cecal parameters and the concentrations of enteric bacteria in the ceca of salmonella-challenged broiler chicks. Poult Sci. 79(2):205–211.
- Strauss ML, Jolly NP, Lambrechts MG, van Rensburg P. 2001. Screening for the production of extracellular hydrolytic enzymes by non-Saccharomyces wine yeasts. J Appl Microbiol. 91(1):182–190.
- Teng P-Y, Chung C-H, Chao Y-P, Chiang C-J, Chang S-C, Yu B, Lee T-T. 2016. Administration of Bacillus amyloliquefaciens and Saccharomyces cerevisiae as direct-fed microbials improves intestinal microflora and morphology in broiler chickens. J Poult Sci. 54(2):134–141.
- Tolik D, Poławska E, Charuta A, Nowaczewski S, Cooper R. 2014. Characteristics of egg parts, chemical composition and nutritive value of Japanese quail eggs-a review. Folia Biol. 62(4):287–292.
- Tomaszewska E, Dobrowolski P, Muszyński S, Kwiecień M, Kasperek K, Knaga S, Tomczyk-Warunek A, Kowalik S, Jeżewska-Witkowska G, Grela ER. 2018. Intestinal mucosa develops in a sex-dependent manner in Japanese quail (Coturnix japonica) fed Saccharomyces cerevisiae. Br Poult Sci. 59(6):689–697.
- Wild G, Searles L, Koski K, Drozdowski L, Begum-Hasan J, Thomson A. 2007. Oral polyamine administration modifies the ontogeny of hexose transporter gene expression in the postnatal rat intestine. Am J Physiol Gastrointest Liver Physiol. 293(2):G453–G460.
- Wright EM. 2013. Glucose transport families SLC5 and SLC50. Mol Aspects Med. 34(2–3):183–196.
- Yalçın S, Oguz F, Guclu B, Yalcin S. 2009. Effects of dietary dried baker’s yeast on the performance, egg traits and blood parameters in laying quails. Trop Anim Health Prod. 41(1):5–10.
- Yalçın S, Yalçın S, Çakın K, Eltan Ö, Dağaşan L. 2010. Effects of dietary yeast autolysate (Saccharomyces cerevisiae) on performance, egg traits, egg cholesterol content, egg yolk fatty acid composition and humoral immune response of laying hens. J Sci Food Agric. 90(10):1695–1701.
- Yang SC, Chen JY, Shang HF, Cheng TY, Tsou SC, Chen JR. 2005. Effect of synbiotics on intestinal microflora and digestive enzyme activities in rats. World J Gastroenterol. 11(47):7413–7417.
- Yeo J, Kim KI. 1997. Effect of feeding diets containing an antibiotic, a probiotic, or yucca extract on growth and intestinal urease activity in broiler chicks. Poult Sci. 76(2):381–385.
- Yoruk MA, Gul M, Hayirli A, Macit M. 2004. The effects of supplementation of humate and probiotic on egg production and quality parameters during the late laying period in hens. Poult Sci. 83(1):84–88.
- Zhang AW, Lee BD, Lee SK, Lee KW, An GH, Song KB, Lee CH. 2005. Effects of yeast (Saccharomyces cerevisiae) cell components on growth performance, meat quality, and ileal mucosa development of broiler chicks. Poult Sci. 84(7):1015–1021.