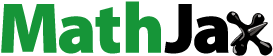
Abstract
Stable isotopes are an important tool to assess livestock diet in binary mixtures of C3-C4 forages. However, the use of stable isotopes to trace livestock diet using tropical arboreal legumes has been limited. The objective of this study was to evaluate the use of 13C stable isotopes to identify the proportions of grass (C4) and legume in sheep diet, when consuming various inclusion levels. We evaluated models correlating faecal δ13C to diet δ13C and inferred which model can better predict the different proportions of grass or legume consumed by sheep. Thirty male lambs (17 ± 1.3 kg) were used, and the experiment was set up in a randomised complete block design with body weight being the criteria for blocking. Treatments included various inclusion levels of Sabiá (Mimosa caesalpiniifolia Benth) in signalgrass (Urochloa decumbens Stapf) hay (100:0, 75:25, 50:50, 25:75 e 0:100%). Total faecal production and in vivo digestibility were calculated, as well as δ13C of diet and faecal samples. Sabia diet (100%) showed the greatest overall intake, and the lowest faecal production was observed when forages were fed alone, both for signalgrass or sabia hays, with 234 and 245 g dry matter animal−1 d−1. The models used within this study estimated with high accuracy the grass-legume proportions within the diets of sheep (R2=0.97). Our results indicate that using additional coefficients (digestibility, discrimination) did not increase the accuracy of the models, since using only isotopes from faeces was sufficient to predict the contribution of C3 or C4 species in the diet.
Stable isotopes are an efficient tool to trace back dietary sources in binary mixtures of C3-C4 forages.
Faecal samples are accurate to use to trace back dietary changes using stable isotopes.
Addition of digestibility and isotope discrimination coefficients did not improve prediction models.
Highlights
Introduction
Evaluation of grazing intake is essential to comprehend the grazing behaviour and dietary sources, improving the overall efficiency of grazing systems (Undi et al. Citation2008). Techniques are available to estimate forage intake by grazing animals (Azevedo et al. Citation2014), however, the prediction of herbage intake from grazing livestock on rangelands is considerably more difficult because of the greater heterogeneity in plant communities, terrain, and climatic conditions (Oltjen and Gunter Citation2015).
Stable carbon isotope ratio analysis is used to determine the proportion of plant functional types consumed as C3 or C4 photosynthetic pathways (Corriale and Loponte Citation2015) by correlating faecal and diet δ13C (Macedo et al. Citation2010). Tropical grasses (C4) and legumes (C3) have different C-fixing pathways, which ultimately results in different mechanisms of discrimination of 13C stable isotope, where in general, C3 species range from −27 to −37‰, while C4 species range from −7 to −17‰ (Fry 2006). Thus, C stable isotopes can act as natural markers from each source to estimate the proportions of each species within a consumed diet (Silva et al. Citation2011). This method could be used in binary mixture of C4 grass and legumes, which is quite common in tropical and subtropical regions. It could also be used in animals feeding on maize, sorghum, and any other C4 crop, when in a mixed diet with other C3 component. So, the application is broad and apply to all productive regions of the world.
Tracing back livestock diet using stable isotopes have been studied by some researchers (Norman et al. Citation2009; Macedo et al. Citation2010; Martins et al. Citation2012) as well as the use of models that can predict the diet of animal that consumed C3 and C4 forage mixtures, via faeces, diet and blood (Norman et al. Citation2009; Pereira Neto et al. Citation2019). A similar approach has not been tested with forages containing high condensed tannins (CT), such as the example of Mimosa caesalpiniifolia. These compounds (i.e. CT) might interfere with digestibility of other compounds and affect faecal output of different dietary components.
The objective of this study was to evaluate the use of 13C stable isotopes to identify the proportions of grass (C4) and of a tannin-rich legume (C3) in sheep diet, when consuming different inclusion levels of the legume in the diet. We assessed various models correlating faecal δ13C to diet δ13C, and made suggestions as to which model can better predict the different proportions of grass or legume consumed by sheep.
Materials and methods
All procedures used in this research are in full compliance with all relevant codes of experimentation and legislation for using experimental animals.
Treatments and experimental design
The experiment was conducted at the Animal Science Department of the Federal Rural University of Pernambuco, in Recife, PE, Brazil. Thirty male Santa Ines lambs were allocated in individual feeding pens, with free access to water and mineral. Average body weight was 17 ± 1.3 kg, and all lambs were six months of age. Treatments consisted of five inclusion levels of the legume sabiá (Mimosa caesalpiniifolia Benth.) in signalgrass (Urochloa decumbens Stapf.) hay (0, 25:75, 50:50, 75:25, and 100%). The experiment was set up as a randomised complete block design, with six replications, with animal body weight being the criteria for blocking.
The experimental period lasted 21 days, in which there was an adaptation period of 16 days and 5 days of collection. Total faecal collections were conducted by placing collection bags on each animal for 24 h and collecting twice daily (6h00 and 16h00) for five days.
Nutritive value
Samples of the five diets were collected to chemical composition analyzes (Table .) and carbon isotope determination, as well as orts, that were collected daily and weighed. The faecal collection bags were weighed upon every collection and thoroughly homogenised. A 400-g representative subsample was obtained from every collection for DM determination, and subsequent analysis. The subsamples were dried in a forced air oven at 55 °C for 24 h, weighed, and ground to pass through 1-mm screen using a Wiley Mill (Thomas-Wiley Scientific). Faecal samples were composited and analysed for dry matter (DM), mineral material (MM), organic matter (OM), ether extract (EE), and neutral detergent fibre (NDF) following methodologies described by AOAC (2005). Total carbohydrate concentration (TC) was estimated according to Sniffen et al. (Citation1992), using the following formula: TC = 100 – (CPdiet + NDF + EE + MM). Dietary CP was estimated by multiplying N concentrations by 6.25 (Table ). The total digestibility of the dietary DM was estimated through the equation described by Azevedo et al. (Citation2014):
Table 1. Chemical composition of diets as fed, with legume sabiá (Mimosa caesalpiniifolia Benth.) in different inclusion levels in signalgrass hay.
δ13C Isotope analysis and calculations
All individual hay samples, as-fed diets, orts, and faecal samples were analysed for the C stable isotope ratio (δ13C). The δ13C denotes a difference measurement made relative to a standard during the actual analysis. The δ13C is estimated as follows: δ13C = [(Rsample/Rstandard)-1]*1000, and results are expressed in ‰. The R represents the ratio between the light to the heavy isotope. The standard for carbon is PeeDee Belemnite (Fry Citation2006). After each sample was ground at 1 mm, they were ball milled using a Mixer Mill MM400 (Retsch) at 25 Hz for 9 min. Samples were then analysed for total C and 13C through the Dumas dry combustion method (Vario Micro Cube, Elementar), coupled to an isotope ratio mass spectrometer (IsoPrime 100, IsoPrime).
Several models were used to calculate the different proportions of C3 and C4 species in the faecal material. Using the two-pool mixing model, described by Fry (2006), the individual proportions of each C3 or C4 could be estimated.
where f1 represents the contribution from the C4 (grass) species, and f2 from C3 (legume) species.
Four models described by Norman et al. (Citation2009) were also tested in this study. The following equation is similar to the two-pool mixing model described above:
(1)
(1)
where %C4 is the proportion of C4 in the sample, A = δ13C of faeces, B = δ13C sabiá, and C = δ13C of signalgrass.
The following equation takes into account 13C discrimination from the diet, in the faeces, and assumes that the discrimination is similar in C3 or C4 species:
(2)
(2)
where J is the difference between the diet δ13C and faeces. In this study, J was a constant variable obtained through the average discrimination across all treatments. The J value used was −0.68‰.
The following equations were used to evaluate the effect of diet digestibility:
(3a)
(3a)
(3b)
(3b)
where D is the digestibility of the C3 species, E is the digestibility of C4 species, F is 100-D, and G is 100-E.
The following equation uses δ13C of faeces from animals consuming exclusively C3 or C4 plants:
where H is the δ13C of faeces from animals consuming only C3 diet, and I is the δ13C of the faeces from animals consuming only C4 species.
Statistical analysis
Data were analysed using the PROC REG procedure in SAS (SAS Institute Citation2000). Fixed effects included the diets, and blocks were considered random effect. A regression equation was calculated between digestibility and intake using proc reg from SAS. Differences were considered significant at the 5% significance level. The probability of difference between diet δ13C and faecal δ13C was compared using a T-distribution test with a paired comparison within each treatment level.
Results
Intake, faecal production, and digestibility
The inclusion of legume in the grass diet did not affect the intakes of DM, OM, NDF, and TC in g DM d−1. Dietary treatments affected the intakes of MM and EE in a linear increasing pattern, and the intakes of CP and NSC in a quadratic pattern, as the inclusion level of the legume hay increased in the diet (Table ). Both treatments with 100% signalgrass and 100% sabiá hay showed the least amount of total faecal production, indicating greater digestibility (DMD) g DM d−1 (Table ).
Table 2. Dry matter, organic matter, nutrient intake, faecal production, body weight, and DM digestibility of sheep fed signalgrass hay with various inclusion levels of legume sabiá (Mimosa caesalpiniifolia Benth.) hay.
Dietary and faecal δ13C
Faecal δ13C became more depleted as sabiá inclusion increased in the diets. In general, faecal material was more depleted in 13C than were the diets (Table ), with the exception of 75% and 100% Sabiá. The fractionation (difference between the dietary and faecal δ13C) observed in the treatment with 0% of sabiá was − 0.53‰, 25% of sabiá was −1.03‰, and 50% of both forages was −1.95‰. It is interesting to note that at 75% and 100% inclusion of sabiá the fractionation did not occur, with similar δ13C for diet and faeces.
Table 3. Dietary and faecal δ13C at different inclusion levels of legume sabiá (Mimosa caesalpiniifolia Benth.) and signalgrass hay.
All the models described were capable of predicting the animal diet, based on faecal 13C (R2 > 0.97: Figure ). The inclusion of additional coefficients within the models (Equations 3a and 3b) did not show significant differences in predicting the animal diet. Overall, all the aforementioned models can successfully predict the proportions of C3 and C4 in the animal diet based on 13C of faecal material (Figure ). Using stable isotopes, animal preference can be determined based on faecal δ13C.
Figure 1. Proportion of C4 plants in the diet, predicted by different models. Equation 1: %C4 = 100- {100*[(δ13C faeces - δ13C; C4 plant)/ (δ13C; C3 plant - δ13C; C4 plant)]}; Equation 2: %C4 = 100-{100*[(δ13C faeces - δ13C; C4 plant-Δδ)/(δ13C; C3 plant - δ13C; C4 plant)]}; Eq 3a: %C4 = 100-100/ ({[Indigestibility C3*((δ13C; C3 plant + Δδ)- δ13C faeces)]/[Indigestibility C4*(δ13C faeces -(δ13C; C4 plant +Δδ)))]} + 1); Equation 3b: %C4 = 100-100/({[Digestibility C3*((δ13C; C3 plant + Δδ)- δ13C faeces)]/[Digestibility C4*(δ13C faeces -( δ13C; C4 plant +Δδ)))]} + 1); Equation 4: %C4 = 100- {100*[(δ13C faeces - δ13C faeces; C3)/(δ13C faeces; C4- δ13C faeces; C3)]}.
![Figure 1. Proportion of C4 plants in the diet, predicted by different models. Equation 1: %C4 = 100- {100*[(δ13C faeces - δ13C; C4 plant)/ (δ13C; C3 plant - δ13C; C4 plant)]}; Equation 2: %C4 = 100-{100*[(δ13C faeces - δ13C; C4 plant-Δδ)/(δ13C; C3 plant - δ13C; C4 plant)]}; Eq 3a: %C4 = 100-100/ ({[Indigestibility C3*((δ13C; C3 plant + Δδ)- δ13C faeces)]/[Indigestibility C4*(δ13C faeces -(δ13C; C4 plant +Δδ)))]} + 1); Equation 3b: %C4 = 100-100/({[Digestibility C3*((δ13C; C3 plant + Δδ)- δ13C faeces)]/[Digestibility C4*(δ13C faeces -( δ13C; C4 plant +Δδ)))]} + 1); Equation 4: %C4 = 100- {100*[(δ13C faeces - δ13C faeces; C3)/(δ13C faeces; C4- δ13C faeces; C3)]}.](/cms/asset/185aeb86-7d42-4b92-bc5b-4f2507df4f10/tjas_a_1881413_f0001_b.jpg)
Discussion
Intake, faecal production, and digestibility
Total DMI and OMI did not increase with legume inclusion likely because the legume hay had similar chemical composition (Table ) and nutritive value than the grass hay (Table ). It is expected an increase when legume is included in the low-quality grass diet, where the digestibility and the intake are changed by that inclusion (Muir et al. Citation2011). Pereira Neto et al. (Citation2019) feeding sheep with mixtures diet of Tifton 85 and Alfalfa hay, found a linear increase in intake when a forage legume (Medicago sativa L.) was added to the grass (Tifton 85 bermudagrass; Cynodon spp.) diet. Differences from these results is likely due to better nutritive value of alfalfa compared with the Mimosa hay used in the current study.
The positive linear increase in the CPI when the legume was included in the diet (Table ) was expected, and it was possible because of the greater CP concentration in the legume hay. The legume CP was 148 g kg−1 compared with the signalgrass CP of 34 g kg−1. Therefore, the greater CP concentration and similar DM intake resulted in greater CP intake for the legume treatment. The signalgrass hay used in this study had low CP if compared with signalgrass hay from other studies likely due to greater maturity at harvesting (Vieira et al. Citation2005; Lima et al. Citation2008; Ramirez Citation2010; Franco et al. Citation2016). Crude protein concentration of sabiá hay was lesser than the ones reported by other studies (Apolinário et al. Citation2015; Vieira et al. Citation2005). The NDF intake did not change with legume inclusion (Table ). This result might be related with the DM intake, that did not increase with legume inclusion as well. Signalgrass hay had great NDF concentration and that might have limited DMI as well.
Total faecal production was reduced when either signalgrass hay or sabiá hay were fed alone, reflecting the greater digestibility of the individual diets compared with the mixed diets. The diet with lower digestibility had greater faecal production, as expected. The digestibility of signalgrass was considerably low. The values obtained was under 400 g kg−1. The DMD of sabia hay in this study was superior to the values (394 g kg−1) observed by Alves et al. (Citation2011) using Sabiá hay to feed sheep.
Dietary and faecal δ13C
The grass and legume δ13C data obtained corroborate the values of C3 and C4 forages described in the literature. Plants with C3 metabolic pathway have δ13C ranging from −20‰ to −32‰ and C4 plants range from −9‰ to −17‰ (Ballentine et al. Citation1998). The signalgrass δ13C observed in the current research (−17.84 ± 0.39) was 13C depleted in relation to some other C4 plants reported in the literature. Norman et al. (Citation2009) reported δ13C of −12.6‰ for C4 grass and Macedo et al. (Citation2010) using Brachiaria dictyoneura observed δ13C of −12.1‰. Pereira Neto et al. (Citation2019) observed δ13C −14.98‰ for Tifton-85 bermudagrass. The δ13C values observed for Sabiá (−33.89 ± 0.50) followed a similar trend, being more depleted in 13C if compared with some other values reported in the literature. Norman et al. (Citation2009) found in alfalfa hay −28.1‰, Macedo et al. (Citation2010) −26.6‰ in Desmodium ovalifolium, while Martins et al. (Citation2012) and Pereira Neto et al. (Citation2019) −31.15 and −30.64‰, respectively. However, the result reported by Costa (Citation2015) working with sabiá (−32.04‰) was closer to the one found in the current study. Biotic and abiotic factors might affect plant isotopic composition along the season (O’Leary Citation1988).
The result from the models, that were used in our study are in accordance with Norman et al. (Citation2009) that used Trifolium incarnatum L. as a C3 plant, and Atriplex nummularia Lindl as a C4 plant species and Pereira Neto et al. (Citation2019) assessing Tifton 85 (Cynodon spp.) and Alfalfa (Medicago sativa L.) hays. In both cases, they applied those models to predict the diet by using the faecal 13C of sheep. Our results indicate that the dietary proportion of C3 and C4 plants can be determined using faecal δ13C with a high precision, and a R2 superior of 0.97. Isotopic fractionation typically occurs when assessing the diet and animal tissues. Oftentimes, animal proteins are naturally 15N enriched relative to the diet of ruminants (Cantalapiedra-Hijar et al. Citation2018). Enrichment typically occurs because the light isotope is preferred in biological reactions and the heavy isotope is left behind, accumulating in the tissues (Fry Citation2006). The enrichment, however, varies with the tissue being analysed. Cantalapiedra-Hijar et al. (Citation2015) observed a negative correlation between Δ15N (δ15Nanimal – δ15Ndiet) and efficiency of N utilisation by the animal, but the highest correlation occurred when Δ15N was calculated from plasma protein. In the current study, however, we analysed the isotopic composition in the faeces using δ13C. There is typically some 13C fractionation between the diet and the faeces, but it is typically of a small magnitude. Macedo et al. (Citation2010) assessed this fractionation (diet vs. faeces) in a study replacing signalgrass by Desmodium ovalifolium and observed a Δ13C (δ13Cfeces – δ13Cdiet) ranging from −0.01 uo to −2.07‰. In the current study, fractionation was significant only when legume and grass inclusion were both 50%. Fractionation might occur because some chemical compounds are more depleted than others. In a study assessing δ13C of different compounds in Lolium perene, lignin, cellulose, and the bulk sample had δ13C of −30.3, −26.5, and −28.5‰, respectively (Fernandez et al. Citation2003), indicating lignin as more depleted than other more soluble compounds. This might partially explain why faeces tend to be more depleted in δ13C. The addition of digestibility and indigestibility in the model, however, did not improve the prediction if compared with the other models. This might be due the relative reduced concentration of lignin compared to other compounds. Moreover, our results also corroborated the possibility to use spot faecal sampling as to avoid total faecal collections; spot-faecal-sampling showed accurate, less stressful, manner of obtaining similar results.
Conclusions
Identifying the different proportions of components within animal diets is important when it comes to reconstruction of animal diets consuming different C3 and C4 species. Stable isotopes can be used to estimate the proportion of each dietary component, which is useful to reconstruct the diet and assess preference and digestibility. Isotopic fractionation between diet and faeces is minimal, allowing for accurate predictions. Fractionation occurs because lignin is more depleted than more soluble compounds such as cellulose and sugars, resulting in more depleted values for faeces. However, because the relative content of lignin in the diet is lesser than other compounds, the fractionation is not large. In this study, fractionation was significantly different only for one replacement level (50% inclusion of both grass and legume). Adding digestibility to the predicting models, did not increase their precision.
The models used in this study were capable of predicting, with precision, the components of sheep’s diet when consuming C4 grass-legume mixed diets. Since our results indicate that adding different variable to models did not increase the precision, it is suitable to use models using only faecal 13C without the need to incorporate additional variables.
Disclosure statement
No potential conflict of interest was reported by the author(s).
References
- Alves AR, Beelen PMG, Medeiros AN. d, Gonzaga Neto S, Beelen RN. 2011. Consumo e digestibilidade do feno de sabiá por caprinos e ovinos suplementados com polietilenoglicol. Rev Caatinga. 24:52–157.
- Apolinário VX, Dubeux JC, Jr, Lira MA, Ferreira RL, Mello AC, Santos MV, Sampaio EVSB, Muir JP. 2015. Tree legumes provide marketable wood and add nitrogen in warm‐climate silvopasture systems. Agron J. 107(5):1915–1921.
- Azevedo EB, Poli CHEC, David DB, Amaral GA, Fonseca L, Carvalho PCF, Fischer V, Morris ST. 2014. Use of faecal components as markers to estimate intake and digestibility of grazing sheep. Livest Sci. 165:42–50.
- Ballentine DC, Macko SA, Turekian VC. 1998. Variability of stable carbon isotopic compositions in individual fatty acids from combustion of C 4 and C 3 plants: implications for biomass burning. Chem Geol. 152(1-2):151–161.
- Cantalapiedra-Hijar G, Dewhurst RJ, Cheng L, Cabrita ARJ, Fonseca AJM, Nozière P, Makowski D, Fouillet H, Ortigues-Marty I. 2018. Nitrogen isotopic fractionation as a biomarker for nitrogen use efficiency in ruminants: a meta-analysis. Animal. 12(9):1827–1837.
- Cantalapiedra-Hijar G, Ortigues-Marty I, Sepchat B, Agabriel J, Huneau JF, Fouillet H. 2015. Diet-animal fractionation of nitrogen stable isotopes reflects the efficiency of nitrogen assimilation in ruminants. Br J Nutr. 113(7):1158–1169.
- Corriale MJ, Loponte D. 2015. Use of stable carbon isotope ratio for foraging behavior analysis of capybara (Hydrochoerus hydrochaeris) from Esteros del Iberá, Argentina. Mamm Biol . 80(2):73–80.
- Costa SBDM. 2015. Potencial produtivo de capim-braquiária [desempenho de bovinos e digestibilidade de leguminosas em sistemas silvipastoris. Thesis ]. of UFRPE. http://www.ppgz.ufrpe.br/sites/ww4.ppgz.ufrpe.br/files/documentos/suellen_brandao_de_miranda_costa_0.pdf. (Accessed 14 July 2020).
- Fernandez I, Mahieu N, Cadisch G. 2003. Carbon isotopic fractionation during decomposition of plant materials of different quality. Glob Biogeochem Cycles. 17(3):1075.
- Franco MO, Detmann E, de Campos Valadares Filho S, Batista ED, de Almeida Rufino LM, Barbosa MM, Lopes AR. 2016. Intake, digestibility, and rumen and metabolic characteristics of cattle fed low-quality tropical forage and supplemented with nitrogen and different levels of starch. Asian-Australas J Anim Sci. 30(6):797–803.
- Fry B. 2006. Stable isotope ecology. Life Sciences Ecology. Library of Congress Control Number 2005937044. New York, NY, Springer, 2006. 308 p.
- Lima ICAR, Lira MA, Mello ACL, Santos MVF, Freitas EV, Ferreira RLC. 2008. Avaliação de sabiazeiro (Mimosa caesalpiniaefolia Benth.) quanto a acúleos e preferência por bovinos. Agraria. 3(3):289–294.
- Macedo R, Tarré RM, Ferreira E, Rezende C. d P, Pereira JM, Cadisch G, Rouws JRC, Alves BJR, Urquiaga S, Boddey RM. 2010. Forage intake and botanical composition of feed for cattle fed Brachiaria/legume mixtures. Sci Agric (Piracicaba, Braz).). 67(4):384–392.
- Martins MB, Ducatti C, Martins CL, Denadai JC, Natel AS, Souza-Kruliski CR, Sartori MMP. 2012. Stable isotopes for determining carbon turnover in sheep feces and blood. Livest Sci. 149(1-2):137–142.
- Muir JP, Pitman WD, Foster JL. 2011. Sustainable, low-input, warm-season, grass–legume grassland mixtures: mission (nearly) impossible? Grass Forage Sci. 66(3):301–315.
- Norman HC, Wilmot MG, Thomas DT, Masters DG, Revell DK. 2009. Stable carbon isotopes accurately predict diet selection by sheep fed mixtures of c3 annual pastures and saltbush or c4 perennial grasses. Livest Sci. 121(2-3):162–172.
- O'Leary, M.H. 1988. Carbon isotopes in photosynthesis. BioScience 38:328–336.
- Oltjen JW, Gunter SA. 2015. Managing the herbage utilisation and intake by cattle grazing rangelands. Anim Prod Sci. 55(3):397–410.
- Pereira Neto JD, Batista Dubeux JC, Ramos de Carvalho FF, Ferreira dos Santos MV, Ruiz-Moreno M, da Silva Santos ER. 2019. Tracing back sheep diet composition feeding grass-legume mixtures using fecal δ13C. Small Ruminant Res. 175:7–14.
- Ramirez MA. 2010. Consumo e digestibilidade aparente de fenos de Brachiaria Decumbens, stapf cultivar brasiliski cortados em tres diferentes idades. Dissertação de mestrado, USP, https://repositorio.ufmg.br/handle/1843/BUOS-8D9FT6. acessado 14/7/2020 as 14:38
- SAS – Statistical Analysis Sistem Institute 2000. General linear Model: 8.2. vol. 716. Cary, NC: SAS Institute.
- Silva AC, Souza AP, Ducatti C, Leonel S. 2011. Renovação do carbono-13 em figueiras ‘roxo de Valinhos. Rev Bras Frutic. 33(2):660–665.
- Sniffen CJ, O'Connor JD, Van Soest PJ, Fox DG, Russell JB. 1992. A net carbohydrate and protein system for evaluating cattle diets: II. Carbohydrate and protein availability. J Anim Sci. 70(11):3562–3577.
- Undi M, Wilson C, Ominski KH, Wittenberg KM. 2008. Comparison of techniques for estimation offorage dry matter intake by grazing beef cattle. Can J Anim Sci. 88(4):693–701.
- Vieira E. d L, Carvalho F. F R d, Batista ÂMV, Ferreira RLC, Santos M. V F d, Lira M. d A, Silva M. J d, Silva E. M B d. 2005. Composição química de forrageiras e seletividade de bovinos em bosque-de-Sabiá (Mimosa caesalpiniifolia Benth.) nos períodos chuvoso e seco. R Bras Zootec. 34(5):1505–1511.