Abstract
Sperm glycocalyx and plasma membrane undergo outstanding modifications during fertilization. However, it is unclear how in vitro capacitation time and acrosome reaction affect the specific location of boar sperm glycoconjugates. This study aimed to identify lectin binding patterns and to describe the sequential changes during different in vitro capacitation times (1 and 4 h) and acrosome reaction in boar spermatozoa. With Aleuria aurantia agglutinin (AAA), most uncapacitated cells were labelled in the postacrosomal region. Nevertheless, after 1 h of in vitro capacitation and the acrosome reaction, most AAA binding sites were in the acrosomal region. With Concanavalin A (ConA), most sperm were labeled in the postacrosomal region before and after capacitation. After the acrosome reaction induction, this pattern changed to a highly stained acrosomal and postacrosomal regions. Peanut agglutinin (PNA) binding sites were in the acrosomal region in uncapacitated and capacitated sperm. In acrosome reacted sperm after 4h capacitation, the most frequent pattern showed remaining positive labeling in the central area of the head. With Pisum sativum agglutinin (PSA), most uncapacitated cells showed a postacrosomal region staining. Nevertheless, faint stained all over the head and highly acrosomal region labelling was observed in the major part of capacitated and acrosome reacted sperm respectively. With Wheat germ agglutinin (WGA), the most representative pattern in uncapacitated, capacitated and acrosome reacted sperm was labelled in the acrosomal region. Regarding capacitation time, the most significant changes in the most representative pattern were observed in acrosome reacted spermatozoa after 4 h of in vitro capacitation.
Lectins allow detailed descriptions of eight different populations in boar sperm.
Changes in lectin binding patterns during capacitation are time dependent.
Lectin binding patterns significantly change after 4 h of in vitro capacitation.
Highlights
Introduction
Mammalian spermatozoa are unable to fertilise an oocyte when ejaculated. They must undergo epididymal maturation, capacitation and acrosome reaction to achieve oocyte fertilisation (Okabe Citation2013). Sperm capacitation was first reported independently by Chang (Citation1951) and Austin (Citation1952). It is a gradual and multi-step process that occurs during the sperm sequential journey along the female genital tract in vivo (Yanagimachi Citation1994; Gervasi and Visconti Citation2016; Stival et al. Citation2016). However, this process has also been successfully reproduced in vitro by incubation in a chemically defined medium in many species (Edwards Citation1969; Rath et al. Citation1999; Albarracín et al. Citation2004; Agarwal et al. Citation2017).
Capacitation appears to be necessary for posterior acrosome reaction and sperm-egg fusion (Inoue et al. Citation2005; Bianchi et al. Citation2014; Cuasnicú et al. Citation2016). In particular, acrosome reaction is characterised as multiple fusions of the plasma membrane with the outer acrosomal membrane and the release of acrosomal contents including hydrolytic enzymes by exocytosis that facilitates the sperm to penetrate the zona pellucida (Okabe Citation2014; Hirose et al. Citation2020). Since the acrosome reaction is an essential step in the fertilisation process and an indicator of sperm capacitation in mammals, the ability of a sperm population to undergo the acrosome reaction might be correlated to its fertilising ability (Xu et al. Citation2018).
The glycocalyx is the primary interface between both spermatozoa and their surrounding environment. Many studies suggest that this sugar-layer plays an important role in sperm transport, gamete recognition and interaction in mammals (Diekman Citation2003; Tecle and Gagneux Citation2015; Suarez Citation2016). For instance, glycocalyx protects sperm against female immunity (Yudin et al. Citation2005; Wigby et al. Citation2019), participates in the formation of the sperm reservoir (Kadirvel et al. Citation2012; Machado et al. Citation2014; Silva et al. Citation2017) or masks molecules involved in fertilisation and passage through the uterotubal junction (Nakanishi et al. Citation2004).
Although several methodologies have been employed to study mammalian gamete glycoconjugates, lectin histochemistry has allowed to evaluate the composition and distribution of glycoconjugates in different mammalian species (Farlin et al. Citation1992; Schroter Citation1999; Gómez-Torres et al. Citation2012; Fàbrega et al. Citation2012). Thereby, it has been observed that the sperm surface undergoes modification, redistribution or loss of specific membrane components during epididymal maturation, sperm capacitation or acrosome reaction (Bawa et al. Citation1993; Jiménez et al. Citation2003; Baker et al. Citation2004). In addition, lectin labelling could be useful to identify and select different sperm subpopulations (Ravid et al. Citation1990; Herrera et al. Citation2002).
In the porcine species, several studies with lectins have allowed to determine the presence of N-acetylglucosamine and/or sialic acid and mannose residues in the sperm head using Wheat germ agglutinin (WGA) and Concanavalin A (Con A), respectively (Vázquez et al. Citation1996; Jiménez et al. Citation2003). On the other hand, the fluorescence microscopy has allowed to know where lectin binding sites are located in boar sperm glycocalyx and their main location changes during different physiological processes (Vázquez et al. Citation1996).
The ability of lectins in the identification and selection of sperm populations has enabled the evaluation of the fertilising ability in boars. Accordingly, Pisum sativum agglutinin (PSA) has been used to evaluate sperm fertilising ability and acrosome reaction in this species (Herrera et al. Citation2002). Moreover, WGA binding was significantly lower in ejaculated, capacitated and acrosome reacted sperm in subfertile boars than in fertile boars using flow cytometry (Jiménez et al. Citation2002). Considering the large number of spermatozoa used in artificial insemination (IA) in this species (Roca et al. Citation2006; Funahashi Citation2015), the development of techniques to identify and select different sperm populations is required to reduce the number of cells used and the cost.
In this way, different lectin binding sites have been reported in boar sperm (Vázquez et al. Citation1996; Jiménez et al. Citation2003). However, most studies describe a general labelling distribution in the most obvious domains using fluorescence microscopy or quantify labelling intensities by flow cytometry. Moreover, the optimal in vitro incubation time required for boar sperm capacitation and its impact on different structural and functional biomarkers is still discussed. Recently, it has showed a significant increase in the number of capacitated spermatozoa after 4 h of incubation in a capacitation medium (Ded et al. Citation2019).
Considering the above, a better understanding of how the boar sperm surface is accurately modified during sperm capacitation and acrosome reaction is convenient. The present study aimed to characterise the porcine sperm glycocalyx and its sequential changes produced after different times of in vitro capacitation and the acrosome reaction using five lectins by fluorescence microscopy.
Materials and methods
Sperm preparation
The ejaculates were obtained from a private company. There were no specific ethical approvals necessary to perform this research because there were non-production animal manipulations that were needed to conduct this study. Five fertility tested boars were included in this report, obtaining an ejaculate from each individual. Sperm-rich fraction was immediately transported to laboratory within 1 h. Percoll treatment consisted of layering a 0.5 mL layout of semen on a discontinuous 45–90% (v/v) Percoll gradient as previously described (Parrish et al. Citation1995) and centrifuging at 700 g for 30 min without brake. Sperm collected from the bottom of the 90% fraction were washed in TALP medium by centrifugation at 700 g for 10 min. Finally, the resultant pellet was resuspended in phosphate-buffered saline without calcium or magnesium (PBS, Biowest, Nuaillé, France) to rinse a final concentration of 20·106 cells mL−1.
Sperm in vitro capacitation and acrosome reaction induction
Percoll recovered sperm was capacitated in vitro for 1 (CS1) and 4 h (CS4) (since start of Percoll treatment) by incubation at 37 °C 5% CO2 in TALP medium (Rath et al. Citation1999) composed of: 114.06 mM NaCl, 3.2 mM KCl, 8 mM C6H10CaO6·5H2O, 0.5 mM MgCl2 6H2O, 0.35 mM NaH2PO4, 25.07 mM NaHCO3, 10 mM NaC3H5O3, 1.1 mM Na pyruvate, 5 mM glucose, 2 mM caffeine, 3 mg mL−1 bovine serum albumin (BSA) (fraction V), 1 mg mL−1 PVA and 0.17 mM kanamycin sulphate. All those reagents were purchased to Sigma (Sigma-Aldrich®, Saint Louis, MO) and were embryo and/or cell culture tested.
To evaluate the success of sperm in vitro capacitation, we determined the immunolocalization of tyrosine phosphorylation in sperm plasma membrane proteins.
The location of tyrosine phosphorylation protein in boar was analysed by indirect immunofluorescence modified from Tardif et al. (Citation2001). Uncapacitated, 1 h capacitated and 4 h capacitated paraformaldehyde-fixed sperm suspensions were deposited on a coverslip. When dry, the samples were washed three times in PBS and permeabilized in absolute ethanol for 1 min. After washing, cells were blocked with 2% (w/v) BSA-PBS for 30 min. Then, coverslips were incubated for 1 h with anti-phosphotyrosine antibodies (4G10, Milipore, Temecula, CA) at 1:100 dilution in room temperature and with a secondary anti-mouse IgG antibody conjugated to Cyanine ™3 (Jackson ImmunoResearch, Ely, UK) at a 1:300 dilution for 1 h in the dark. Slides were rinsed with PBS between both incubations. Finally, coverslips were washed again three times with PBS and subsequently mounted with Vectashield H-1200 with 4′,6-diamidino-2 phenylindole (DAPI, Vector Laboratories, Burlingame, CA). Negative control experiments were performed omitting the primary antibody.
The capacitation status was determined observing the different tyrosine phosphorylation patterns (Luño et al. Citation2013). Only the pattern considered as ‘high capacitated’ was represented in order to simplify tyrosine phosphorylation results. This pattern is characterised by presenting fluorescence in the equatorial subsegment and acrosomal region (Figure ). At least 200 cells were evaluated for each in vitro physiological condition. Leica TCS-SP2 confocal microscopy (Leica Microsystems GmBH, Wetzlar, Germany), and Leica Confocal Software helped obtain images.
Figure 1. Evaluation of sperm capacitation status and acrosome reaction induction. (A) 4G10 phosphorylation patterns. Note the signal in the equatorial subsegment and acrosomal region (white arrow), pattern considered as ‘high capacitated’. (B) PNA-label. Spermatozoa with fluorescence in the acrosomal region was considered not reacted (−) and those with faint labelling in the equatorial segment were regarded as acrosome reacted (+).
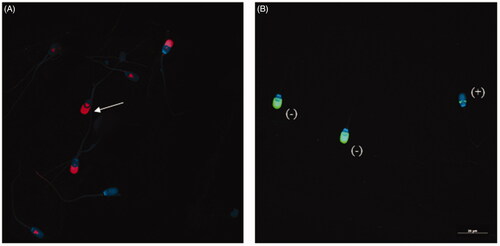
Acrosome reaction was induced by incubation of 5·106 spermatozoa with 1 µM calcium ionophore A23187 (Sigma-Aldrich, Saint Louis, MO) for 15 min (Ashworth et al. Citation1995). Two replicates were made for each sample, one at 1 h (ARS1) and the other at 4 h (ARS4) of incubation in capacitation medium.
Based on previous studies, the effectiveness of the acrosome reaction induction was performed omitting calcium ionophore A23187 as control (Sáez-Espinosa et al. Citation2019). To differentiate between acrosome-intact and acrosome-reacted sperm, the cells were treated with FITC-labelled Peanut agglutinin (PNA), which has been described as a marker for the outer acrosome membrane (Fazeli et al. Citation1997). A total of 5 μL of acrosome reaction control were placed on a coverslip and fixed in methanol for 30 min. After drying the smear, the cells were washed three times in PBS and unspecific binding was blocked by 2% (w/v) BSA-PBS for 30 min. The smears were then incubated in the dark with PNA lectin conjugated to fluorescein-5 isothiocyanate (PSA-FITC, Sigma-Aldrich) 20 μg mL−1 for 30 min. After three washes in PBS (Biowest, Nuaillé, France), the samples were mounted with Vectashield and DAPI. DAPI helped with identification of the cell nucleus. The whole process took place at room temperature.
Sperm that showed fluorescence in the acrosomal region were considered not reacted and those with faint labelling in the equatorial segment were regarded as acrosome reacted (Figure ). At least 200 cells were evaluated for each in vitro physiological condition. Leica TCS-SP2 confocal microscopy (Leica Microsystems GmBH, Wetzlar, Germany), and Leica Confocal Software helped obtain images.
Sperm sample fixation
Sperm from groups Uncapacitated (UCS), one-hour-capacitated (CS1), four-hours-capacitated (CS4), acrosome reacted after one-hour-capacitation (ARS1) and acrosome reacted after four-hours-capacitation (ARS4) were fixed as follows: sperm was centrifuged three times for 5 min at 700 g and pellet was washed with PBS. Final resuspension was made in PBS 2% (w/v) paraformaldehyde (Electron Microscopy Sciences, Hatfield, PA) and spermatozoa were incubated for 45 min at 4 °C. Afterwards, sperm was centrifuged 5 min at 400 g and washed with PBS until lectin binding assays were made.
Lectin binding
Carbohydrates surface distribution in UCS, CS1, CS4, ARS1 and ARS4 was characterised using AAA, Con A, PNA, PSA and WGA lectins conjugated with FITC (Vector Laboratories, Burlingame, CA) (see Table for taxonomic name of source and specifity of lectins used). Fixed samples (5 µL) were deposited on a round coverslip of 12 mm. The coverslips incubated with PNA lectin were permeabilized in methanol for 30 min. Once coverslip was dried, it was rehydrated thrice with PBS for 5 min and incubated with 2% (w/v) BSA-PBS block solution for 1 h. Next, samples were incubated with lectin FITC-conjugated at a final concentration of 20 μg mL−1 for 1 h at room temperature in a dark humid chamber. Finally, samples were washed thrice in PBS for 5 min and assembled with Vectashield with DAPI. Quantification of different staining patterns (P) was performed by scoring 100 cells of each sample using a Leica TCS SP2 laser scanning confocal microscope. Added to this, negative control experiments were performed omitting the lectin.
Table 1. Carbohydrates affinity of lectins used in this study.
Statistical analysis
The Shapiro–Wilk test supported the adjustments of the percentage values to the normal curve. Statistical differences between different in vitro physiological conditions and capacitation time were tested for the different lectins using t-student distribution. Differences were statistically significant at a 95% confidence level (p < 0.05). The statistical analysis was performed by means of IBM SPSS Statistics19.0 (IBM, Armonk, NY).
Results
Evaluation of sperm capacitation and acrosome reaction induction
The distribution of tyrosine phosphorylation fluorescence patterns was significantly different (p < 0.05) among UCS, CS1 and CS4. In UCS, only the 1.86 ± 0.66% of cells showed the pattern considered as ‘highly capacitated’, while in CS1 and CS4 the percentage of this pattern was 25.64 ± 6.07% and 49.81 ± 6.79%, respectively. The percentages obtained of acrosome-reacted spermatozoa was 14.69 ± 5.41% in ARS1 controls and 21.60 ± 2.63% in ARS4 controls.
Lectin labelling patterns
We analysed all the staining patterns observed with the different lectins in UCS, CS1, CS4, ARS1 and ARS4 which correspond to different membrane domains along the sperm head (Figure ). We just considered those were present over 5% of sperm sample. Eight different lectin binding patterns were detected (Figure ) that seemed to be consistent. Lectin binding patterns observed on sperm were the following: Pattern 1 (P1): Highly stained acrosomal region (AAc) with maximum fluorescence in apical ridge (ApR); Pattern 2 (P2): Faint stained all over the head; Pattern 3 (P3): Highly stained AAc and anterior (PAcA) and posterior postacrosomal region (PAcP) with no labelling in the equatorial segment (EqS); Pattern 4 (P4): labelling in the postacrosomal region (PAcA + PAcP); Pattern 5 (P5): Highly stained AAc and faint labelling in EqS and PAcP with a non-labelled PAcA; Pattern 6 (P6): Highly stained EqS; Pattern 7 (P7): Stained AAc with maximum fluorescence at the end of AAc; Pattern 8 (P8): faint labelling in EqS. Not all lectin binding patterns were observed for all lectins (Figure ). Due to the wide variability in the number of lectin labelling patterns, the most frequent one depending on the in vitro physiological condition and the lectin used is showned in Figure .
Figure 2. (A) Different membrane domains identified in this study. ApR: apical ridge; AAc: acrosomal region; EqS: equatorial segment; PacA: anterior postacrosomal region; PacP: posterior postacrosomal region. Modified from Bruckbauer et al. (Citation2010). (B) Lectin staining patterns of boar sperm head. It is shown models of the staining pattern and the images taken by confocal microscopy (×63).
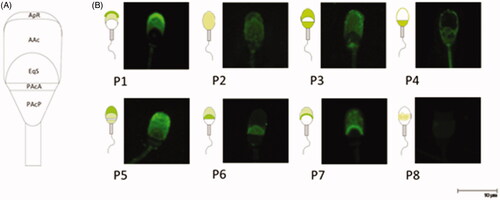
Distribution of AAA
We characterised four clearly defined patterns P1, P4, P6 and P7 with AAA lectin. Specifically, we observed that in UCS, the most representative pattern was P4 (53.47%) and in CS1 this pattern changed significantly to P1 (56.92%) (Figure ). In CS4, the most common pattern was P7 (40.41%) followed by the pattern P1 (38.85%). We also detected a significant increase of P1 after 1 and 4 h capacitation compared to uncapacitated spermatozoa. After acrosome reaction induction, the most abundant subpopulation showed P7 both after 1 (ARS1) and 4 h (ARS4) of in vitro capacitation (64.52 and 64.53%, respectively). In this way, P7 increased as the same time that P1 diminished significantly when the acrosome reaction was induced after 1 and 4 h of in vitro capacitation. No differences between 1 and 4 h of incubation in ARS and CS were observed.
Distribution of ConA
With Con A lectin, we identified a total of five different patterns: P2, P3, P4, P5 and P7 (Figure ). The most common pattern was P4 in UCS (66.14%), CS1 (51.10%) and CS4 (56.02%) (Figure ). In addition, P5 was the second most-observed pattern in UCS, CS1 and CS4. P2 appeared only in CS1 and CS4. After acrosome reaction induction, P3 was the main pattern observed in both ARS1 (68.27%) and ARS4 (57.75%), followed by P7. On the other hand, P4 and P5 did not appear in acrosome reacted spermatozoa (ARS1 and ARS4). No statistical differences between in vitro capacitation (1 h and 4 h) nor after acrosome reaction were observed.
Distribution of PNA
The PNA lectin was used for the assessment of the reacted sperm percentage. Thus, we identified only two patterns with PNA lectin: P1 and P8. All UCS and CS1 included in this study showed P1 with PNA lectin. This pattern was considered as non-reacted sperm. However, CS4 showed P1 (94.64%) and P8 (5.36%). After the acrosome reaction induction in both capacitation conditions (1 and 4 h), the most common pattern was P1 (62.43%) in ARS1 and P8 (64.33%) in ARS4 (Figure ). We detected significant differences between ARS1 (37.57%) and ARS4 (64.33%) for P8, pattern that was considered as reacted.
Distribution of PSA
With PSA lectin, we observed six different lectin patterns: P1, P2, P4, P5, P6 and P7. The most common pattern in UCS was P4 (54.20%) followed by P2 (35.88%) (Figure ). However, after in vitro capacitation this pattern changed to P2 in CS1 (67.78%) and CS4 (42.76%). P4 only appeared in UCS and CS1 while P6 did not appeared in that in vitro physiological conditions. In ARS1 the most representative pattern was P7 (51.12%) followed by P1 (43.52%) and in ARS4 was P1 (42.73%) (Figure ). In addition, after acrosome reaction induction (ARS1 and ARS4), P2 did not be observed. We observed a significant increase of P6 between ARS1 (5.36%) and ARS4 (29.11%).
Distribution of WGA
We recognised four different patterns with WGA lectin: P1, P2, P3 and P7. The main pattern in UCS was P1 (55.76%) (Figure ). After 1 h (CS1) and 4 h (CS4) of capacitation, P1 was also the most representative (66.75 and 68.39%, respectively). No statistical differences between UCS and CS1/CS4 sperm were observed with WGA lectin, except with P2, which diminished significantly after 4 h of in vitro capacitation. Neither differences between CS1 and CS4 were observed. In contrast, significant differences were obtained after acrosome reaction induction; P7 pattern was the principal pattern in ARS1 (40.22%) and ARS4 (40.80%). Moreover, P2 did not appear in acrosome reacted spermatozoa (ARS1 and ARS4) (Figure ).
Discussion
The spermatozoon is a complex cell whose surface is notably modified from the passage through the epididymis until the moment of fertilisation (Tecle and Gagneux Citation2015). The use of lectins has allowed to accurately locate glycocalyx components in the head of boar spermatozoa and to identify the changes produced during in vitro capacitation at different times. The findings obtained for tyrosine phosphorylation as a key process of sperm capacitation justify the effectiveness of the methodology used in this study. Thus, we observed a significant and progressive increase in tyrosine phosphorylation pattern considered as ‘high capacitated’ during in vitro capacitation time. Similarly, it has been described a time-dependent increase of the aforementioned pattern during in vitro capacitation, reaching almost 40% (Ded et al. Citation2019). Likewise, we have been described the accurate location of lectin binding sites after the acrosome reaction. The specific distribution of lectin binding in boar spermatozoa observed in this study provides additional evidence of the presence of regionalisation in the plasma membrane surface (Gadella Citation2017).
AAA labelling
Studies with AAA to detect terminal fucose in porcine sperm are further scarce. In contrast, most reports have used Ulex europaeus agglutinin (UEA) to study α-linked fucose in boar sperm. In this way, difficulty in locating UEA binding sites using fluorescence microscopy has been described (Vázquez et al. Citation1996). The use of flow cytometry, however, has effectively managed to detect fucose residues using UEA lectin (Jiménez et al. Citation2003).
The presence of terminal fucose in the postacrosomal region in uncapacitated cells has also been observed in mice (Baker et al. Citation2004). Nevertheless, in the present study labelling in the equatorial segment was observed in around 20% of cells in this experimental condition. This location has been described in humans using AAA lectin in uncapacitated sperm, being related to cells reacted spontaneously (Gómez-Torres et al. Citation2012).
During in vitro capacitation, we observed an increase in a pattern with faint fluorescence in the acrosomal region after 1 and 4 h of incubation. A loss of fucose detection during in vitro capacitation has also been observed both in the presence of oviductal fluid in goats (Taitzoglou et al. Citation2007) and TALP-HEPES medium in pigs (Jiménez et al. Citation2003). This pattern continued to increase after acrosome reaction induction in both experimental conditions. Thereby, it could be explained by the loss of plasma and outer acrosomal membrane after the exocytosis process and the subsequent exposure of the inner acrosomal membrane during the acrosome reaction.
Con A labelling
Mannose expression has been previously correlated with the fertilising ability in boars (Jiménez et al. Citation2002). Regarding mannose location, it has been described in the acrosomal region using Con A-HRP in uncapacitated boar spermatozoa (Töpfer-Petersen et al. Citation2009). In our study, the most uncapacitated spermatozoa fluoresced in the postacrosomal region. However, we observed a pattern with high acrosomal region labelling in more than 30% of uncapacitated cells, being our second most abundant pattern in this in vitro physiological condition. Differences in both studies could be due to the sample processing conditions.
After both capacitation experimental conditions, we identified that the most abundant pattern remained unchanged, followed by one which presented labelling in acrosomal region. Other studies observed that after 4 h of in vitro capacitation, FITC-Con A staining was concentrated on the acrosomal region of boar sperm (Jiménez et al. Citation2003). It is possible that our second most observed pattern was the identified by this group since they did not register percentages of different lectin binding patterns. Moreover, the in vitro capacitation method in both studies was different.
Cells underwent several changes after acrosome reaction induction due to most of the reacted sperm in both experimental conditions showed a pattern with fluorescence in acrosomal and postacrosomal regions. In humans, the location of Con A binding sites in the postacrosomal region has been observed in fertile subjects and subsequently related to proper sperm functionality (Bains et al. Citation1992). Another representative pattern in reacted cells showed faint fluorescence only in acrosomal region with a maximum of fluorescence at the lower part of this domain. A similar pattern labelled in the acrosomal region has been described using oocyte coculture as acrosome reaction induction method (Vázquez et al. Citation1996). This pattern could be due to the loss of membrane during acrosome reaction and probably the labelling we observed was in the inner acrosomal membrane. This fact is supported by the presence of Con A binding sites in the inner acrosomal membrane described in thin sections in boars (Töpfer-Petersen et al. Citation2009).
PNA labelling
All spermatozoa evaluated with PNA lectin in our study showed fluorescence at the acrosomal region with high intensity in the apical ridge before in vitro capacitation. Other authors also showed that peroxidase-labelled PNA lectin binds to the acrosomal region in 94.6% of boar ejaculated sperm (Vázquez et al. Citation1996). However, we observed that this percentage was progressively decreasing during in vitro capacitation until reaching less than 40% in acrosome-reacted spermatozoa after 4 h of capacitation. A similar decrease of this pattern was observed in zona pellucida-reacted spermatozoa (56%) (Vázquez et al. Citation1996).
Galactose distribution in the acrosomal region has been previously correlated with acrosome-intact boar spermatozoa (Serrano et al. Citation2001). Moreover, the high density of molecules in the apical ridge could be related to a mechanism of protection against intracellular membrane fusion (Boerke et al. Citation2008). This would explain the presence of strong fluorescence intensity in the apical ridge in most sperm analysed before of the acrosome reaction induction.
The reduction in acrosome-intact cells could be due to a decrease of galactose residues during acrosome reaction. In this way, the most common pattern after acrosome- reaction induction after 4 h of incubation in a capacitation medium showed a light fluorescence in equatorial region, which could correspond to acrosome-reacted spermatozoa. Accordingly, other authors have described percentages of around 75% of cells reacted after 4 h of in vitro capacitation, although using zona pellucida-induced acrosome reaction (Serrano et al. Citation2001). These findings corroborate the use of PNA lectin to identify and select sperm population with proper membrane integrity.
PSA labelling
Regarding PSA lectin, glycoproteins or glycolipids that carry mannose residues may migrate from the postacrosomal region in uncapacitated cells to the acrosomal region during capacitation. This reorganisation would be explained by the presence of a homogeneous pattern overall sperm head in most of 1 and 4 h-capacitated cells.
On the other hand, we observed that the most common lectin binding pattern in acrosome reacted spermatozoa changed after both capacitation times. After 1 h of incubation and the subsequent induction of the acrosome reaction, most abundant pattern presented maximum fluorescence in the lower part of the acrosomal region. However, after 4 h of incubation, most of the spermatozoa showed a maximum fluorescence in the apical ridge. In this way, the changes observed after acrosome reaction induction were time dependent.
We also observed a significant increase of a pattern which showed faint fluorescence labelling in the acrosomal region and higher fluorescence labelling in the equatorial segment in acrosome reacted cells after 4 h of in vitro capacitation. According to these findings we could consider PSA lectin as an acrosomal status indicator (Berger Citation1990). Herrera et al. (Citation2002) explained that sperm without acrosomal FITC-PSA fluorescence or with only FITC-PSA equatorial segment fluorescence were considered acrosome-reacted using progesterone such as acrosome reaction inductor. Thus, they obtained percentages of induced acrosome reaction from 5 to 20% after 4 h of incubation. These results are similar to those obtained with our P6 pattern, which showed fluorescence in the equatorial segment in about 30% of acrosome-reacted after 4 h of incubation cells. The differences in the percentage of acrosome-reacted cells with these authors were probably related to the acrosome reaction induction method.
WGA labelling
N-Acetylglucosamine and/or sialic acid residues on the sperm membrane are indicators of sperm fertilising ability. In this way, reproductive problems have been associated with a decrease in the aforementioned residues in boar sperm surface (Jiménez et al. Citation2002).
In our study, WGA lectin is specially located in the acrosomal region in uncapacitated spermatozoa. In this experimental condition, we also observed that the sum of the percentage of P1 and P3, which showed fluorescence in acrosomal region, was around 90%. Other studies have also suggested that over 90% of ejaculated sperm showed the main concentration of binding sites in the acrosomal region (Vázquez et al. Citation1996). The second most observed pattern in uncapacitated sperm presented faint fluorescence in the whole head in the 30% of cells. Likewise, Jiménez et al. (Citation2003) concluded that fresh boar spermatozoa showed a considerable number of N-acetylglucosamine and sialic acid residues over all plasma membrane. However, they did not distinguish different patterns, so it is possible that they were describing our second most observed pattern in uncapacitated cells.
WGA binding sites also remained in the acrosomal region after 1 and 4 h of in vitro capacitation in most cells of our study. According to our findings, WGA HRP-conjugated labelling pattern did not change during the in vitro capacitation in boar spermatozoa (Vázquez et al. Citation1996). We also observed that a pattern which showed faint labelling throughout the head was significantly lower in 1-h-capacitated sperm in comparison with uncapacitated sperm. This pattern suggests the release of sialic acid residues in acrosomal region, which is an important event in capacitation for the subsequent ZP recognition (Lassalle and Testart Citation1994).
After acrosome reaction in both capacitation times, we observed that most cells showed a pattern with weak fluorescence intensity in the acrosomal region, probably associated with the decrease in WGA binding sites after the acrosome reaction (Jiménez et al. Citation2003). Specifically, this pattern change may be due to the elimination of sialic acid, which acts as a protective cover for the sperm (Lassalle and Testart Citation1994) or could be due to the loss of plasma and acrosomal outer membrane during acrosome reaction. Perhaps WGA binding sites in these acrosome-reacted spermatozoa are in the inner acrosomal membrane and are involved in recognition processes.
As described above, we tested the effect of different capacitation time (1 and 4 h) on lectin binding patterns. It is known that sperm capacitation and membrane fusion are time-dependent processes (de Jonge Citation2017). We especially observed significant differences in acrosome reacted spermatozoa after 4 h of in vitro capacitation (Figures and ). This is according to other authors that consider over 4 h of incubation to acquire the capacitated status (Ded et al. Citation2019) and fertilising ability (Imai et al. Citation1979).
Conclusions
The use of lectins has allowed to describe different lectin binding patterns, reporting the presence of sperm subpopulations in boars. On the other hand, we have determined in a precise way the sequential kinetics of surface carbohydrates during in vitro capacitation and acrosome reaction and the effect of incubation time in a capacitating medium.
Disclosure statement
No potential conflicts of interest were reported by the author(s).
Additional information
Funding
References
- Agarwal S, Kharche SD, Bhatiya AK. 2017. Study of TALP and TRIS citrate medium on caprine sperm capacitation and subsequent in vitro embryo production. Iran J Vet Res. 18(4):275–278.
- Albarracín JL, Mogas T, Palomo MJ, Peña A, Rigau T, Rodríguez-Gil JE. 2004. In vitro capacitation and acrosome reaction of dog spermatozoa can be feasibly attained in a defined medium without glucose. Reprod Domest Anim. 39(3):129–135.
- Ashworth PJC, Harrison RAP, Miller NGA, Plummer JM, Watson PF. 1995. Flow cytometric detection of bicarbonate-induced changes in lectin binding in boar and ram sperm populations . Mol Reprod Dev. 40(2):164–176.
- Austin CR. 1952. The capacitation of the mammalian sperm. Nature. 170(4321):326.
- Bains H, Sehgal S, Bawa SR. 1992. Human sperm surface mapping with lectins. Acta Anat (Basel). 145(3):207–211.
- Baker SS, Thomas M, Thaler CD. 2004. Sperm membrane dynamics assessed by changes in lectin fluorescence before and after capacitation. J Androl. 25(5):744–751.
- Bawa SR, Pabst MA, Werner G, Bains HK. 1993. Capacitated and acrosome reacted spermatozoa of goat (Capra indicus): a fluorescence and electron microscopic study. Andrologia. 25(3):123–135.
- Berger T. 1990. Pisum sativum agglutinin used as an acrosomal stain of porcine and caprine sperm. Theriogenology. 33(3):689–695.
- Bianchi E, Doe B, Goulding D, Wright GJ. 2014. Juno is the egg Izumo receptor and is essential for mammalian fertilization. Nature. 508(7497):483–487.
- Boerke A, Tsai PS, Garcia-Gil N, Brewis IA, Gadella BM. 2008. Capacitation-dependent reorganization of microdomains in the apical sperm head plasma membrane: functional relationship with zona binding and the zona-induced acrosome reaction. Theriogenology. 70(8):1188–1196.
- Bruckbauer A, Dunne PD, James P, Howes E, Zhou D, Jones R, Klenerman D. 2010. Selective diffusion barriers separate membrane compartments. Biophys J. 99(1):L1–L3.
- Chang MC. 1951. Fertilizing capacity of spermatozoa deposited into the fallopian tubes. Nature. 168(4277):697–698.
- Cuasnicú PS, Da Ros VG, Weigel Muñoz M, Cohen DJ. 2016. Acrosome reaction as a preparation for gamete fusion, in: Molecular reproduction and development. Reprod Biol Endocrinol. 220:159–172.
- de Jonge C. 2017. Biological basis for human capacitation-revisited. Hum Reprod Update. 23(3):289–299.
- Ded L, Dostalova P, Zatecka E, Dorosh A, Komrskova K, Peknicova J. 2019. Fluorescent analysis of boar sperm capacitation process in vitro. Reprod Biol Endocrinol. 17(1):109.
- Diekman AB. 2003. Glycoconjugates in sperm function and gamete interactions: how much sugar does it take to sweet-talk the egg? Cell Mol Life Sci. 60(2):298–308.
- Edwards RG, Bavister BD, Steptoe PC. 1969. Early stages of fertilization in vitro of human oocytes maturated in vitro. Nature. 221(5181):632–178.
- Fàbrega A, Puigmulé M, Dacheux JL, Bonet S, Pinart E. 2012. Glycocalyx characterisation and glycoprotein expression of Sus domesticus epididymal sperm surface samples. Reprod Fertil Dev. 24(4):619–630.
- Farlin ME, Jasko DJ, Graham JK, Squires EL. 1992. Assessment of Pisum sativum agglutinin in identifying acrosomal damage in stallion spermatozoa. Mol Reprod Dev. 32(1):23–27.
- Fazeli A, Hage WJ, Cheng FP, Voorhout WF, Marks A, Bevers MM, Colenbrander B. 1997. Acrosome-intact boar spermatozoa initiate binding to the homologous *Zona pellucida in vitro. Biol Reprod. 56(2):430–438.
- Funahashi H. 2015. Methods for improving in vitro and in vivo boar sperm fertility. *Reprod Domest Anim. 50:40–47.
- Gadella BM. 2017. Reproductive tract modifications of the boar sperm surface. Mol Reprod Dev. 84(9):822–831.
- Gervasi MG, Visconti PE. 2016. Chang’s meaning of capacitation: a molecular perspective. Mol Reprod Dev. 83(10):860–874.
- Gómez-Torres MJ, Avilés M, Girela JL, Murcia V, Fernández-Colom PJ, Romeu A, De Juan J. 2012. Characterization of the lectin binding pattern in human spermatozoa after swim-up selection. Histol Histopathol. 27(12):1621–1628.
- Herrera J, Fierro R, Zayas H, Conejo J, Jiménez I, García A, Betancourt M. 2002. Acrosome reaction in fertile and subfertile boar sperm. Arch Androl. 48(2):133–139.
- Hirose M, Honda A, Fulka H, Tamura-Nakano M, Matoba S, Tomishima T, Mochida K, Hasegawa A, Nagashima K, Inoue K, et al. 2020. Acrosin is essential for sperm penetration through the *Zona pellucida in hamsters. Proc Natl Acad Sci U S A. 117(5):2513–2518.
- Imai H, Niwa K, Iritani A. 1979. Time requirement for capacitation of boar spermatozoa assessed by their ability to penetrate the zona-free hamster egg. J Reprod Fertil. 56(2):489–492.
- Inoue N, Ikawa M, Isotani A, Okabe M. 2005. The immunoglobulin superfamily protein Izumo is required for sperm to fuse with eggs. Nature. 434(7030):234–238.
- Jiménez I, González-Márquez H, Ortiz R, Betancourt M, Herrera J, Fierro R. 2002. Expression of lectin receptors on the membrane surface of sperm of fertile and subfertile boars by flow cytometry. Arch Androl. 48(2):159–166.
- Jiménez I, González-Márquez H, Ortiz R, Herrera JA, Garcı́a A, Betancourt M, Fierro R. 2003. Changes in the distribution of lectin receptors during capacitation and acrosome reaction in boar spermatozoa. Theriogenology. 59(5–6):1171–1180.
- Kadirvel G, Machado SA, Korneli C, Collins E, Miller P, Bess KN, Aoki K, Tiemeyer M, Bovin N, Miller DJ. 2012. Porcine sperm bind to specific 6-sialylated biantennary glycans to form the oviduct reservoir. Biol Reprod. 87:147.
- Lassalle B, Testart J. 1994. Human Zona pellucida recognition associated with removal of sialic acid from human sperm surface. J Reprod Fertil. 101(3):703–711.
- Luño V, López-Úbeda R, García-Vázquez FA, Gil L, Matás C. 2013. Boar sperm tyrosine phosphorylation patterns in the presence of oviductal epithelial cells: in vitro, ex vivo, and in vivo models. Reproduction. 146(4):315–324.
- Machado SA, Kadirvel G, Daigneault BW, Korneli C, Miller P, Bovin N, Miller DJ. 2014. LewisX-containing glycans on the porcine oviductal epithelium contribute to formation of the sperm reservoir. Biol Reprod. 91:1–9.
- Nakanishi T, Isotani A, Yamaguchi R, Ikawa M, Baba T, Suarez SS, Okabe M. 2004. Selective passage through the uterotubal junction of sperm from a mixed population produced by chimeras of calmegin-knockout and wild-type male mice. Biol Reprod. 71(3):959–965.
- Okabe M. 2013. The cell biology of mammalian fertilization. Development. 140(22):4471–4479.
- Okabe M. 2014. Mechanism of fertilization: a modern view. Exp Anim. 63(4):357–365.
- Parrish JJ, Krogenaes A, Susko-Parrish J. 1995. Effect of bovine sperm separation by either swim-up or Percoll method on success of in vitro fertilization and early embryonic development. Theriogenology. 44(6):859–869.
- Rath D, Long CR, Dobrinsky JR, Welch GR, Schreier LL, Johnson LA. 1999. In vitro production of sexed embryos for gender preselection: high-speed sorting of X-chromosome-bearing sperm to produce pigs after embryo transfer. J Anim Sci. 77(12):3346–3352.
- Ravid A, Sagiv M, Bartoov B, Eltes F, Novogrodsky A, Allalouf D, Levinsky H, Singer R. 1990. Separation of sub-populations of sperm with higher fertility potential from normal and pathological semen by peanut agglutinin. Andrologia. 22(3):225–230.
- Roca J, Vázquez JM, Gil MA, Cuello C, Parrilla I, Martínez EA. 2006. Challenges in pig artificial insemination. Reprod Domest Anim. 41(s2):43–53.
- Sáez-Espinosa P, Huerta-Retamal N, Robles-Gómez L, Avilés M, Aizpurua J, Velasco I, Romero A, Gómez-Torres MJ. 2019. Influence of in vitro capacitation time on structural and functional human sperm parameters. Asian J Androl. 21:1–7.
- Schroter S, Osterhoff C, McArdle W, Ivell R. 1999. The glycocalyx of the sperm surface. Hum Reprod Update. 5(4):302–313.
- Serrano H, Diaz-Esparza L, García-Suárez D. 2001. Pig sperm membrane integrity evaluated by lectin labeling. Arch Androl. 47(1):59–65.
- Silva E, Frost D, Li L, Bovin N, Miller DJ. 2017. Lactadherin is a candidate oviduct Lewis X trisaccharide receptor on porcine spermatozoa. Andrology. 5(3):589–597.
- Stival C, Puga Molina L del C, Paudel B, Buffone MG, Visconti PE, Krapf D. 2016. Sperm capacitation and acrosome reaction in mammalian sperm. In: Buffone MG, editors. Sperm acrosome biogenesis and function during fertilization, advances in anatomy, embryology and cell biology. Cham: Springer International Publishing; p. 93–106.
- Suarez SS. 2016. Mammalian sperm interactions with the female reproductive tract. Cell Tissue Res. 363(1):185–194.
- Taitzoglou IA, Kokoli AN, Killian GJ. 2007. Modifications of surface carbohydrates on bovine spermatozoa mediated by oviductal fluid: a flow cytometric study using lectins. Int J Androl. 30(2):108–114.
- Tardif S, Dubé C, Chevalier S, Bailey JL. 2001. Capacitation is associated with tyrosine phosphorylation and tyrosine kinase-like activity of pig sperm proteins. Biol Reprod. 65(3):784–792.
- Tecle E, Gagneux P. 2015. Sugar-coated sperm: unraveling the functions of the mammalian sperm glycocalyx. Mol Reprod Dev. 82(9):635–650.
- Töpfer-Petersen E, Januschke E, Schmoeckel C, Schill WB. 2009. Ultrastructural localization of lectin binding sites of the acrosomal membrane system of boar spermatozoa. Andrologia. 16(6):539–547.
- Vázquez JM, Martínez E, Pastor LM, Roca J, Matas C, Calvo A. 1996. Lectin histochemistry during in vitro capacitation and acrosome reaction in boar spermatozoa: new lectins for evaluating acrosomal status of boar spermatozoa. Acta Histochem. 98(1):93–100.
- Wigby S, Suarez SS, Lazzaro BP, Pizzari T, Wolfner MF. 2019. Sperm success and immunity. In: Current topics in developmental biology. New York, NY: Elsevier Inc.; p. 287–313.
- Xu F, Guo G, Zhu W, Fan L. 2018. Human sperm acrosome function assays are predictive of fertilization rate in vitro: a retrospective cohort study and meta-analysis. Reprod Biol Endocrinol. 16:1–29.
- Yanagimachi R. 1994. Fertility of mammalian spermatozoa: its development and relativity. Zygote. 2(4):371–372.
- Yudin AI, Treece CA, Tollner TL, Overstreet JW, Cherr GN. 2005. The carbohydrate structure of DEFB126, the major component of the cynomolgus macaque sperm plasma membrane glycocalyx. J Membr Biol. 207(3):119–129.