Abstract
Early-life thermal conditioning (TC) in broiler chickens has long-lasting impacts on later life. Therefore, this study aimed to clarify the molecular mechanism by which TC impacts the hepatic expression heat shock proteins (hsps), antioxidant enzymes, NADPH oxidase 4 (NOX4), glucocorticoid receptor (GR), and DNA methyltransferases genes in broiler chicks at five weeks of age. Two hundred forty one-day-old male broiler chicks of Cobb 500 were allocated into four equal experimental groups. The first group was under the optimal brooding conditions (control), whereas the 2nd, 3rd, and 4th groups were exposed to TC at 39 ± 1 °C for six hours on the third-, fifth- or seventh-day post-hatch, respectively. At 35 days of age, all chicks of four experimental groups were subjected to heat challenge by exposure to 36 ± 1 °C for six hours. Results showed that broilers exposed to TC on the fifth-day post-hatch when exposed to acute heat stress at five weeks of age had the lowest plasma CORT level, the lowest expression (p < .05) for NOX4, GR, SOD, SOD2, and CAT among all groups. The same trend was observed for hsp70, hsp90A, hsp90B, hsp60, and hspA9. In conclusion, early thermal conditioning on the fifth-day post-hatch may improve thermotolerance by decreasing NOX4 and GR expressions, leading to reduced heat shock proteins and antioxidant enzyme gene expression subsequently reduced oxidative stress.
Thermal conditioning is a promising approach to mitigate heat stress in broiler chicks.
The optimal age to perform thermal conditioning is a controversial issue.
Heat shock proteins gene expression and plasma corticosterone are good indicators for thermotolerance acquisition.
Thermal conditioning on the fifth-day post-hatch reduced oxidative stress at five weeks of age.
Highlights
Introduction
Heat stress has attracted significant attention from all interested in poultry production, either scientists or producers, for decades. It is considered one of the most substantial challenges that face poultry production associated with economic losses and negative impacts related to growth performance (Rajkumar et al. Citation2011; Xie et al. Citation2015; Akbarian et al. Citation2016). Broiler chicks are more vulnerable to negative impacts caused by heat stress than other domestic animals (Mitchell et al. Citation2005). The eukaryotic alleviate heat stress by introducing heat shock proteins (hsps) (Barna et al. Citation2018; Perini et al. Citation2020). Hsps are a group of proteins classified based on their molecular weight in hsp 100, hsp 90, hsp70, hsp60, and small hsp families (Belhadj Slimen et al. Citation2016). Heat stress induces the synthesis of hsps to prevent protein misfolding and aggregation, renature misfolded proteins, remove unstable proteins, etc. (Zilaee et al. Citation2014).
Heat stress also induces oxidative stress characterised by the excessive production of reactive oxygen species (ROS) and surpasses antioxidant capacity in broiler chicks (Akbarian et al. Citation2016). Heat stress increases ROS production by introducing NADPH oxidase 4 (NOX4) expression in avian muscle cells (Kikusato et al. Citation2015). To overcome the detrimental consequences of heat stress, the antioxidant defence system, including superoxide dismutase (SOD), superoxide dismutase 2 (SOD2), and Catalase (CAT), activates to maintain redox (antioxidant/prooxidant) balance and control free radical (Surai et al. Citation2019). It has been confirmed that post-hatch thermal conditioning (TC) induces thermotolerance at later life through epigenetic memory (Tzschentke et al. Citation2004; Yahav Citation2009; Tanizawa et al. Citation2014; Vinoth et al. Citation2016). The induced epigenetic adaptation that has a long-lasting impact might be due to ambient temperature changes during a critical phase in developing the thermoregulation system during the postnatal period (Tzschentke et al. Citation2004). Therefore, determining these critical stages (optimal age to perform thermal conditioning) is controversial (Halevy Citation2020). DNA methylation, well-characterised epigenetic modifications, plays a significant role in controlling gene expression (Li and Zhang Citation2014; Wan et al. Citation2015) .
Although a plethora of research has been reported that early thermal conditioning promotes broiler chickens' resistance to heat stress, the molecular mechanisms governing thermal conditioning are not clearly understood. This study aimed to evaluate the effects of TC on hepatic gene expression later in life at five weeks old of broiler chickens.
Materials and methods
Animals and experimental design
Two hundred forty male broiler chicks (1 day old) of Cobb 500 were obtained from a local commercial hatchery. Chicks weighed, on average 45.5 ± 0.5g, were randomly assigned to four experimental groups (60 chicks each). Chicks of each group were divided into five replicates. In the first group (CON.0), six birds were rapidly decapitated, and blood was collected in heparinised tubes. The liver was dissected and snap-frozen in liquid nitrogen and stored at −80 °C until further gene expression analysis. The remained birds were reared under the ambient temperature throughout the experimental period. The second group was subjected to thermal conditioning (TC) at 39 °C and 48%±2 RH for 6 hours on the third day of age, six birds decapitated and collected samples (blood and liver) as (TC3) and its relative control sampled (blood and liver) as (C3). The third and fourth groups (TC5 and TC7) were subjected to thermal conditioning on the fifth and seventh day of age, and their relative controls (C5 and C7) were sampled, respectively. Exposed groups were transferred to the heating chamber to maintain the environmental conditions and perform thermal conditioning. The control group (all unconditioned birds) was kept under 30 ± 2 °C and 55%±2 RH during the first week, and then gradually decreased by 0.5 °C/day until 25 ± 1 °C was reached, and persistent till the end of the experimental period. Broiler chicks were fed ad-libitum, and drinking water was free access throughout the experimental period. Broiler chicks were reared on wire cages. All cages were equipped with feeding hoppers made of galvanised steel and automatic drinkers (nipples). The birds were provided 24 hours light on the first day of placement and then adjusted to 23 hrs lighting regime up to the marketable age (35 days). Chicks were fed a commercial starter diet for the first 21 days of age, followed by a pelleted grower-finisher diet from 22 to 35 days of age, to meet the Cobb 500 strain recommendations.
At the end of the experiment that lasted five weeks, all chicks from control and thermal conditioned groups were exposed to heat challenge at 36 °C for 6 hours, resulting in four groups, control exposed (CE), 3-day conditioned exposed (TCE3), 5-day conditioned exposed (TCE5), and 7-day conditioned exposed (TCE7).
Liver samples collection
Six chicks from CON.0, thermal conditioned groups (TC3, TC5, and TC7) and their respective control groups (C3, C5, and C7) were decapitated, plasma samples collected and stored at −20 °C, liver samples were removed, immediately dissected, snap-frozen in liquid nitrogen, and stored at −80 °C until further gene expression studies. At the end of the experiment, six birds from each group (CE, TCE3, TCE5, and TCE7) were slaughtered, plasma samples collected and stored at −20 °C, and liver samples were removed, immediately dissected, snap-frozen in liquid nitrogen, and stored at −80 °C until further gene expression studies.
Assessment of corticosterone (CORT) hormone
Blood samples were collected at slaughter time in heparinised tubes, centrifuged at 4,000 xg for 10 min at 4 °C. Collected plasma was stored at −20 °C until determination. The plasma corticosterone level was determined by The IBL Corticosterone Enzyme Immunoassay Kit, IBL International GmbH (Catalog NO. RE52211).
Total RNA isolation and real-time quantitative RT-PCR
Total RNA was extracted from quick-frozen liver samples using TRIzol® reagent (Invitrogen Life Technologies, Palo Alto, CA, USA) according to the manufacturer instructions. After homogenising the sample with TRIzol® Reagent, then chloroform is added, RNA is precipitated with isopropanol then treated with DNase I, RNase-free (Thermo Fisher Scientific, Cat. #EN0521). The total RNA was purified by RNeasy mini kit (Qiagen, Valencia, CA, USA). The RNA quality and quantity were determined using agarose gel electrophoresis and NanoDrop 1000 (Thermo Scientific, Wilmington, DE, USA). Four micrograms of total RNA from fresh-frozen liver were converted into cDNA using cDNA Revert Aid First Strand cDNA Synthesis Kit (Thermo Fisher Scientific, Cat. # K1621) according to the manufacturer instructions. The cDNA reactions were diluted using a 10-fold dilution factor using DNase/RNase-Free water.
Specific primer pairs for the mRNA of the selected genes (Table ) were designed using Primer-BLAST software (www.ncbi.nlm.nih.gov/tools/primer-blast). We tested different annealing temperatures to optimise each pair of primers using conventional PCR to exclude the presence of unspecific products or primer dimer synthesis; then, the PCR products were analysed by 2% agarose gel electrophoresis. Each qPCR reaction had a final volume of 15 µL of the reaction mixture, which consisted of 7.5 µL 2X SYBR Green PCR master mix kit (Thermo Fisher Scientific, Cat. #4344463), DNase/RNase-Free water, 0.3 μM forward and reverse specific primers for each gene (Table ) and 2 µL of cDNA template. The qPCR analyses were performed in the Rotor-Gene Q system (Qiagen, Hilden, Germany). Thermal cycling profiles were initiated by one denaturation step of 10 min at 95 °C, followed by 40 cycles with the following parameters: denaturation at 95 °C for 30 sec, annealing at the specific annealing temperature of each primer pair (Table ) for 15 sec and extension at 72 °C for 30 sec. Fluorescence data were acquired at the end of the extension step. Dissociation protocol with a gradient (0.5 °C every 30 sec.) from 65 °C to 95 °C was used to investigate the specificity of the qPCR and the presence of primer dimers. We used Chicken glyceraldehyde 3-phosphate dehydrogenase (GAPDH) and β-actin as internal controls. Dissociation curves were constructed to validate the quality of data at the end of amplification. All qRT-PCR experiments were performed in triplicate, and the values of the average cycle threshold (Ct) were determined and Delta-Ct scores for gene transcripts in each sample were normalised using Delta-Ct scores for GAPDH/β-actin and expressed as the fold change in gene expression using the equation, 2−ΔΔCT.
Table 1. A list of the primer’s sequences and annealing temperature (Tm) used in the present study.
Statistical analyses
Statistical differences between each group before and after the 2nd heat exposure were assessed by a one-way analysis of variance (ANOVA) using the SAS 8.0 software (SAS Institute Inc., Cary, NC, USA). Comparisons between the control group's mean values and those of each experimental group were performed using the Duncan test for multiple comparisons. P values of less than 0.05 were regarded as statistically significant.
Results
Stress hormone (corticosterone)
There were significant increases among the experimental groups considering plasma corticosterone (CORT) levels in comparison with the control group (p < .05; Table ). The fifth-day conditioned group was the lowest level compared to other conditioned groups (the third and seventh-day groups) at the time of post-conditioning. Moreover, TCE5 showed the lowest significant value at the end of the experimental period (35 days of age (DOA)). There was a reduction in corticosterone by 54% in the TCE5 group compared to the control exposed group (CE).
Table 2. Effect of thermal conditioning (TC) on plasma corticosterone (CORT) levels (ng/mL) of broiler chicks.
mRNA expression of hepatic hsp70, hsp60, hsp90B and hsp90A
Hepatic hsp70, hsp60, hsp90B, and hsp90A mRNA expression of broiler chicks exposed to thermal conditioning (TC) at 3, 5- and 7-day post-hatch and re-exposed to acute heat stress at five weeks of age are presented in Figure )). Early thermal conditioning increased mRNA expression of hsp70 significantly by 147, 60, and 428%, hsp60 by 91, 156, and 195%, hsp90B by 114, 90, and 195% in the third, the fifth, and the seventh day, respectively, compared to non-exposed groups. Moreover, hsp90A mRNA expression increased significantly by 44 and 70% on the third and seventh days groups (TC3 and TC7), respectively. However, no significant changes on the fifth day group (TC5) due to thermal conditioning compared to its relative control (C5). There were age-related gene expression changes; hsp70 and hsp90A expression increased with age, but hsp60 and hsp90B expression decreased with age.
Figure 1. Effect of thermal conditioning (TC) on mRNA expression of hsp70, hsp60, hsp90B and hsp90A in the liver of broiler chicks. Con.0: Control post-hatch; C3: control on 3 day of age (DOA); TC3: thermal conditioning on 3 DOA; C5: control on 5 DOA; TC5: thermal conditioning on 5 DOA; C7: control on 7 DOA; TC7: thermal conditioning on 7 DOA; CE: control at 35 DOA; TCE3: 3-day conditioned exposed at 35 DOA; TCE5: 5-day conditioned exposed at 35 DOA; TCE7: 7-day conditioned exposed at 35 DOA.
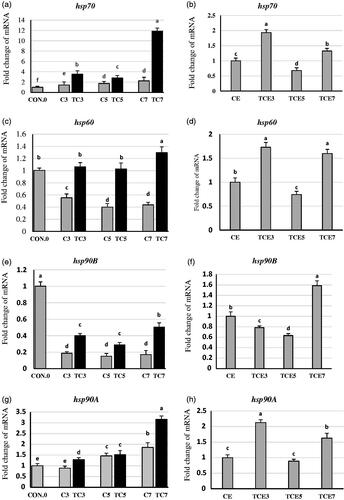
At five weeks of age (Figures ,h)), the third and seventh-day groups followed a similar expression trend related to hsp70, hsp60, and hsp90A. There were significant increases in gene expression of hsp70 by 92, 32%, hsp60 by 72, 59%, and hsp90A by 112 and 62% in the third and seventh -day groups, respectively, compared to the control exposed group (CE). In contrast, on the fifth day, there were significant decreases in mRNA expression of hsp70 and hsp60 by 32 and 25%, respectively, compared to the CE group. However, there were no significant changes in the fifth-day group related to hsp90A gene expression compared to CE. In the third and fifth days, thermal conditioned groups decreased hsp90B gene expression significantly by 21 and 36%, respectively, compared to CE. However, gene expression for hsp90B increased significantly by 58% in the seventh-day group compared to CE.
mRNA expression of mitochondrial related genes hspA9, SOD2 and NOX4
In the liver of thermally conditioned groups (Figure )), hspA9, SOD2, and NOX4 expressions were significantly increased by 192, 64, and 84%, respectively for the fifth-day group. Also, hspA9, SOD2, and NOX4 increased by 165, 203, and 65%, respectively, for the seventh-day group compared to its relative control. In contrast, there were significant declines by 85, 16, and 52% in gene expression of hspA9, SOD2, and NOX4, respectively, due to thermal conditioning on the third- day group compared with its control.
Figure 2. Effect of thermal conditioning (TC) on mRNA expression of hspA9, SOD2 and NOX4 in the liver of broiler chicks. Con.0: Control post-hatch; C3: control on 3 DOA; TC3: thermal conditioning on 3 DOA; C5: control on 5 DOA; TC5: thermal conditioning on 5 DOA; C7: control on 7 DOA; TC7: thermal conditioning on 7 DOA; CE: control at 35 DOA; TCE3: 3-day conditioned exposed at 35 DOA; TCE5: 5-day conditioned exposed at 35 DOA; TCE7: 7-day conditioned exposed at 35 DOA.
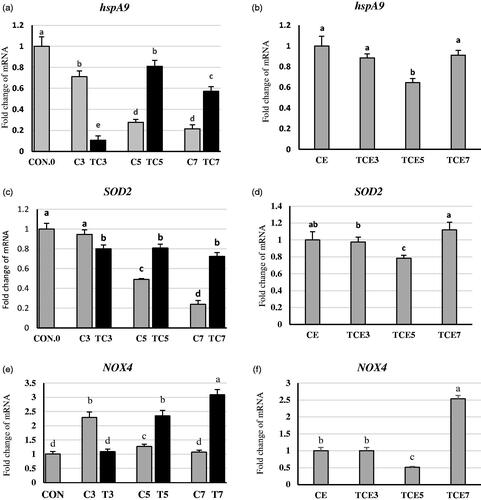
It is worthy to notice that the expression of all previous three genes declined with age. Interestingly, there were no significant gene expression changes (Figure ) for hspA9, SOD2 genes due to acute heat stress at five weeks of age in TCE3 and TCE7 compared to CE. However, in TCE5 (Figure )), there were significant decreases by 35 and 21% in mRNA expression of hspA9, SOD2, respectively, compared to CE. Concerning NOX4, there was a considerable decrease of 48% in TCE5, conversely, significantly increased by 158% in TCE7. However, no significant changes in TCE3 due to acute heat stress compared to CE.
Upregulation of hepatic CAT and SOD
TC significantly upregulated antioxidant enzymes CAT and SOD gene expression (Figure ). The increased percentage in expressions was 174, 286, and 700% in TC3, TC5, and TC7, respectively, compared to their respective CAT controls. The same trend of expressions was obtained in SOD. Thermal conditioning significantly increased gene expression of SOD by 103, 103, and 229% in TC3, TC5, and TC7, respectively, compared to their respective controls. At five weeks of age, acute heat stress significantly increased CAT and SOD mRNA expression (Figure ) by 21, 111, and by 28, 78% in TCE3 and TCE7, respectively, compared to CE. Converse results were observed in TCE5. CAT gene expression decreased but not significantly by 7% in TCE5. However, the SOD gene expression significantly decreased by 29% in TCE5 compared to CE.
Figure 3. Effect of thermal conditioning (TC) on mRNA expression of CAT and SOD in the liver of broiler chicks. Con.0: Control post-hatch; C3: control on 3 DOA; TC3: thermal conditioning on 3 DOA; C5: control on 5 DOA; TC5: thermal conditioning on 5 DOA; C7: control on 7 DOA; TC7: thermal conditioning on 7 DOA; CE: control at 35 DOA; TCE3: 3-day conditioned exposed at 35 DOA; TCE5: 5-day conditioned exposed at 35 DOA; TCE7: 7-day conditioned exposed at 35 DOA.
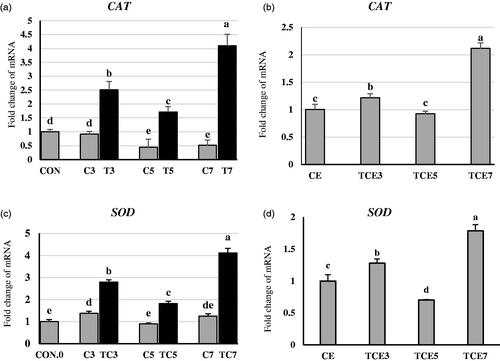
DNA methylation regulators and glucocorticoid receptor gene expression in liver
In the liver of thermally conditioned birds at days 3 and 7, DNMT1 and DNMT3A expression (Figure ) were increased by (59 and 50% for DNMT1, 38 and 58% for DNMT3A) respectively, compared to their respective controls. However, in the TC5 group, DNMT1 and DNMT3A expression were decreased by 27 and 28% respectively, compared with its control. Hepatic DMAP1 mRNA expression significantly increased by 85% in the TC5 group compared to the non-conditioned group. A converse trend was observed in the TC7 group. DMAP1 mRNA expression (Figure ) significantly decreased by 37% in comparison with the control group. However, DMAP1 showed no significant changes in the TC3 group. Concerning DNMT3B (Figure ), early thermal conditioning significantly decreased DNMT3B mRNA expression by 30 and 33% in TC3 and TC5, respectively, compared to their respective non-conditioned groups. In contrast, DNMT3B mRNA expression significantly increased by 79% in TC7 compared to control.
Figure 4. Effect of thermal conditioning (TC) on mRNA expression of DNMT1, DNM3A, DMAP1, DNMT3B, and GR in the liver of broiler chicks. Con.0: Control post-hatch; C3: control on 3 DOA; TC3: thermal conditioning on 3 DOA; C5: control on 5 DOA; TC5: thermal conditioning on 5 DOA; C7: control on 7 DOA; TC7: thermal conditioning on 7 DOA; CE: control at 35 DOA; TCE3: 3-day conditioned exposed at 35 DOA; TCE5: 5-day conditioned exposed at 35 DOA; TCE7: 7-day conditioned exposed at 35 DOA.
At five weeks of age (Figure )), all four mentioned genes showed no significant TCE3 group changes compared to the CE group. However, the TCE7 group for DNMT1, DNMT3A, DMAP1, and DNMT3B showed significant increases by 63, 35, 53, and 35%, respectively, in gene expression compared to the CE group. In contrast, the TCE5 group for DNMT1, DNMT3A, DMAP1, and DNMT3B showed significant decreases by 25, 31, 17, and 31%, respectively, in gene expression compared to the CE group.
Glucocorticoid receptor (GR) expression was increased following TC5 and TC7 (Figure ) by 120 and 153% compared to non-conditioned controls. However, there were no significant changes in TC3. Interestingly, at five weeks of age (Figure ) GR expression decreased significantly by 30% in the TCE5 group compared to the CE group. In contrast, TCE3 and TCE7 groups showed significant increases by 61 and 56% compared to the CE group.
Discussion
Corticosterone hormone responses
Expose broiler chicken to a high ambient temperature above the thermoneutral zone between (18–24 °C) in poultry for a short time is considered acute heat stress. Thermal stress (31 and 36 °C) from day 35 to 42 has impaired productive performance in broiler chickens (Quinteiro-Filho et al. Citation2010) and in rabbits (Madkour et al. Citation2020). Moreover, it has been reported that corticosterone could be used as an excellent biomarker of acute heat stress (Dou et al. Citation2019). In the current study, after exposure of all treatment groups to heat challenge (36 °C for 6 h.) at five weeks of age resulted in a reduction of corticosterone level (the gold standard stress marker) in TCE5 compared to control exposure and other heat conditioned groups (TCE3 and TCE7), suggesting mitigation of stress induced by thermal stress (Baxter et al. Citation2020). As a response to stress, the HPA axis activates and provides metabolic support by mobilising stored energy, suppressing the immune response, and potentiating numerous sympathetically mediated effects (Ulrich-Lai and Herman Citation2009; van Bodegom et al. Citation2017). The superiority of the TCE5 group birds may indicate more maturation of the hypothalamic–pituitary–adrenal (HPA) axis, leading to more resistance to stressors.
HSPS mRNA expressions
Previous studies showed that post-hatch thermal conditioning increased thermotolerance when broiler chickens were exposed to late heat stress (Zaboli et al. Citation2017; Kang et al. Citation2019). The most appropriate age to exploit and induce thermotolerance in broiler chickens was before the age of 7 days (Yahav et al. Citation2005). Yet, the molecular mechanisms are unclear (Hensch Citation2004; King et al. Citation2014). Therefore, the current study aimed to expand our understanding of the molecular mechanisms by which early thermal conditioning impacts later life through evaluating the effects of TC (39 °C for 6 hours) at different ages and subsequent AHS on hepatic gene expression for hsps, antioxidants enzymes, glucocorticoid receptor, and DNA methyltransferase.
Hsps exert a defensive role against heat stress to facilitate protein folding and refolding during stress, protect them from degradation, and maintain the structural integrity of the cells (Hao and Gu Citation2014; Lowman et al. Citation2014; Zulkifli et al. Citation2014; Cedraz et al. Citation2017; Wan et al. Citation2017). Living cells respond to environmental stressors through hsps synthesis's upregulation, leading to enhance tolerance against various stress conditions/factors (Surai Citation2015). In the same line, our current study indicated that early thermal conditioning in the first week of age upregulated hsp70, hsp60, hsp90B, and hsp90A in the liver. This increase in hsps could be a cytoprotective mechanism that further permits the cells to survive. Interestingly, the expression's magnitude was age-dependent (Blake et al. Citation1990; Gabriel et al. Citation1996). The highest expression for all genes was demonstrated at the 7-day TC. Increased hsps expression levels at 7-day might be due to old birds being more sensitive to high temperatures rather than young ones. Supporting this hypothesis, the 7-day TC group had the highest gene expression levels for NOX4 and GR genes, indicating that the group was more stressed than others.
In a recent study (Suri and Vaidya Citation2015), exposure to early life stress could lead to either stress resistance can cope with stress or enhance the susceptibility to negative consequences of stress in later life. In the current study, at five weeks of age, when all groups exposed to acute heat stress 36 °C for 6 hours, the TCE5 group exhibited the lowest levels of expression for all hsps compared to CE and other thermal conditioned groups (TCE3 and TCE7). In contrast, TCE3 and TCE7 showed higher gene expressions when compared to CE and TCE5. It has been reported that the cellular mechanisms by which thermal conditioning has a long-lasting thermotolerance effect are complex and contradicting (Rajkumar et al. Citation2017). For example, previous studies highlighted that heat tolerance was associated with the upregulation of hsp in poultry (Wang and Edens Citation1998; Al-Zghoul et al. Citation2013), while others reported that thermotolerance was related to the downregulation of hsps in broilers (Rajkumar et al. Citation2015; Vinoth et al. Citation2015; Rajkumar et al. Citation2017; Vinoth et al. Citation2018). Our findings included the two previous thoughts, wherein TCE3 and TCE7 followed the first school of thought; however, TCE5 followed the second one. Our findings showed that the thermally conditioned group on the fifth day of age downregulated NOX4 and GR by 48 and 30%, respectively, compared to CE after AHT at five weeks of age.
In contrast, TCE3 and TCE7 upregulated NOX4 and GR, indicating that TCE5 is more adapted than the other two thermally conditioned groups and the control exposed group (CE). Several studies targeted to define the optimal time period, duration, and level of heat acclimation in chickens (Moraes et al. Citation2004; Yahav et al. Citation2004; Collin et al. Citation2007; Tona et al. Citation2008). Improved thermotolerance by thermal conditioning during post-hatch resulted from changes in ambient temperature at “critical developmental phases” of the thermoregulation system that induce epigenetic adaptation that have long-lasting positive effects (Tzschentke et al. Citation2004). In the current study, the divergent heat shock responses between the thermally conditioned groups at different ages may be due to the five days of age is a critical time in the transformation of hypothalamic neuronal thermosensitivity, wherein hypothalamic neuronal warm sensitivity increased and cold sensitivity reduced (Tzschentke and Basta Citation2000). Additionally, Surai (Citation2015) mentioned that differences in hsp expression depend on the strength of stress. Such findings showed that the levels of expression for hsps on the fifth day were significantly lower than other days (third or seventh), especially in hsp70 and hsp90, indicating that birds that settled in thermal conditioning (the fifth day) on that day were less stressed than other different days.
Antioxidant enzymes and NADPH oxidase 4 responses
Thermal stress induces mitochondrial dysfunction (Akbarian et al. Citation2016). The metabolic demand and mitochondrial biogenesis increased during heat stress, so cells increase their energy expenditure and consequently increase ROS production (Mujahid et al. Citation2007; Yang et al. Citation2010). The elevation of ROS production not only through mitochondria but also through NOX4 (Kikusato et al. Citation2015). Excess ROS production induces oxidative stress associated with a modulation of NOX4 and SOD2 gene expression (Akbarian et al. Citation2016; Al-Zghoul et al. Citation2019). Consistent with previous studies, TC (39˚C for 6 hours) on 5- or 7-day post-hatch resulted in significant increases in hepatic gene expressions of NOX4, SOD2, and hspA9.
In contrast, TC on the third day of age decreased significantly all mentioned genes compared to their relatives’ control. This might be due to the lower expression of NOX4 may reduce the expression of anti-oxidative related genes like SOD2 and hspA9 (Saleh et al. Citation2020). Or maybe due to the third-day thermal conditioning group can perform a negative feedback adaptive mechanism to protect the liver from the potential damage due to heat stress. The hspA9 was initially identified as a 75 kDa protein and was categorised as one of the hsp70 families (Wadhwa et al. Citation2002). The present finding follows the previous studies stating that hspA9 prevents oxidative damage in hepatic rats by ameliorating mitochondrial function (E et al. Citation2013).
Thermal conditioning on the fifth day of age reduced NOX4, SOD2 significantly, and hspA9 gene expressions at five weeks of age compared to CE, TCE3, and TCE7 groups indicating that TC5 group has a long-lasting positive effect for reducing oxidative stress at five weeks of age through the downregulation of NOX4. A study carried out by Kikusato et al. (Citation2015) mentioned that NOX4 is the primary ROS source. So the reduction in NOX4 gene activity in the TCE5 group in our study is an indicator of alleviating oxidative stress (Al-Zghoul et al. Citation2019). Consistent with our results, NOX4 and SOD2 gene expression decreased after exposure to acute heat stress in thermally manipulated birds (Al-Zghoul et al. Citation2019; Saleh et al. Citation2020).
It has been reported that the rapid increase of ROS production under heat stress in broiler chicks (Lin et al. Citation2006; Mujahid et al. Citation2006; Yang et al. Citation2010; Del Vesco et al. Citation2017). Meanwhile, antioxidant enzymes such as CAT and SOD activity increased to eliminate or neutralise ROS's adverse effect (Cui et al. Citation2014; Hu et al. Citation2019). In the present study, exposure to high temperature (TC) upregulated CAT and SOD to cope with oxidative stress (Cui et al. Citation2014). This increase in CAT activity and SOD activity might be a protective mechanism to prevent liver damage and minimise oxidative stress during heat stress (Tan et al. Citation2010; Hao and Gu Citation2014; Rimoldi et al. Citation2015). Moreover, such findings indicated that increased ROS production due to heat stress induces antioxidant enzymes (Zhang et al. Citation2014). This upregulation of antioxidant enzymes might scavenge ROS and protect the organism from heat stress (Yang et al. Citation2010).
Interestingly, SOD and CAT were downregulated in the TCE5 group at five weeks of age, indicating that oxidative stress was alleviated in this group. Although, to best our knowledge, there are no reports investigate the effect of post-hatch thermal conditioning on antioxidant enzymes gene expression, Al-Zghoul et al. (Citation2019) and Saleh et al. (Citation2020) found that decreased SOD and CAT gene expression in thermally conditioned birds during embryogenesis after exposure to acute heat stress. It is noteworthy that such findings indicated that thermal conditioning on the fifth day of age has a long-lasting positive effect in reducing oxidative stress through decrease expressions of NOX4, SOD2, SOD, and CAT in the liver. Taking the results concerning hsps together with the results mentioned above, we suggest that early thermal conditioning on the fifth day induce thermotolerance adaptation through the downregulation of hsps and Antioxidant enzymes as well as NOX4 genes.
DNA methyltransferases response
It has been reported that early post-hatch thermal conditioning induces DNA methylation changes that are involved in thermotolerance adaptation to heat stress at later life (Vinoth et al. Citation2018; Cramer et al. Citation2019). There is a scarce of information about the effect of early thermal conditioning on DNA methyltransferases DNMTs. We found one study tried to distinguish between resilience and vulnerable chicks after exposure to early thermal conditioning (Cramer et al. Citation2019). They found that decrease the activity of DNMT in resilience chicks, on the contrary, increases the activity of DNMT in vulnerable chicks. Moreover, Bartlett et al. (Citation2019) reported that in response to environmental stress, GR acts to reshape epigenetic changes. Kang et al. (Citation2017) found that early life nutritional stress-induced epigenetic changes in hepatic GR expression through passive demethylation. In the current study, we found that TC on the fifth day of age caused downregulation of all DNMTs and GR, indicating stress resilience. However, the other two (TC3 and TC7) thermally groups are stress vulnerable. Exposing broiler chicks during early age on the third or fifth day of age is an effective way to induce thermotolerance in later life (Yossifoff et al. Citation2008; Kisliouk and Meiri Citation2009). However, determining the best day to perform thermal conditioning is a controversial issue because it depends on time and strength.
Conclusions
The presented findings showed that early thermal conditioning leads to future resilience or vulnerability to heat stress at later life, wherein TC3 and TC7 lead to make birds sensitive as indicated by the overexpression of hsps and antioxidant enzymes as well as NOX4 and that supported by upregulation of DNMTs at five weeks old. On the contrary, TC5 leads to make birds resilient as indicated by a reduction of corticosterone hormone, downregulation of hsps, antioxidant enzymes, NOX4, and DNMTs at five weeks old.
It is concluded, according to the results, the best timing for thermal conditioning at 39˚C for 6 hours was the fifth day of age because it has long-lasting positive impacts through decreased the CORT level and gene expression of NOX4 and subsequently reduced oxidative stress.
Animal welfare statement
The authors confirm that they have followed EU standards for the protection of animals used for scientific purposes.
Ethical approval
The experimental design and all the research protocols were approved by the Medical Research Ethics Committee (MREC) of the National Research Centre with ethical approval code 20/107.
Acknowledgments
This paper is based upon work supported by Science, Technology & Innovation Funding Authority (STDF) under grant ((STDF - RSG #34824).
Disclosure statement
No potential conflict of interest was reported by the author(s).
Additional information
Funding
References
- Akbarian A, Michiels J, Degroote J, Majdeddin M, Golian A, De Smet S. 2016. Association between heat stress and oxidative stress in poultry; mitochondrial dysfunction and dietary interventions with phytochemicals. J Anim Sci Biotechnol. 7(1):37.
- Al-Zghoul M-B, Al-Zhgoul M-B, Dalab AES, Ababneh MM, Jawasreh KI, Al Busadah KA, Ismail ZB. 2013. Thermal manipulation during chicken embryogenesis results in enhanced Hsp70 gene expression and the acquisition of thermotolerance. Res Vet Sci. 95(2):502–507.
- Al-Zghoul M, Sukker H, Ababneh M. 2019. Effect of thermal manipulation of broilers embryos on the response to heat-induced oxidative stress. Poult Sci. 98(2):991–1001.
- Barna J, Csermely P, Vellai T. 2018. Roles of heat shock factor 1 beyond the heat shock response. Cell Mol Life Sci. 75(16):2897–2916.
- Bartlett AA, Lapp HE, Hunter RG. 2019. Epigenetic mechanisms of the glucocorticoid receptor. Trends Endocrinol Metab. 30(11):807–818.
- Baxter MFA, Greene ES, Kidd MT, Tellez-Isaias G, Orlowski S, Dridi S. 2020. Water amino acid-chelated trace mineral supplementation decreases circulating and intestinal HSP70 and proinflammatory cytokine gene expression in heat-stressed broiler chickens. J Anim Sci. 98(3):skaa049.
- Belhadj Slimen I, Najar T, Ghram A, Abdrrabba M. 2016. Heat stress effects on livestock: molecular, cellular and metabolic aspects, a review. J Anim Physiol Anim Nutr. 100(3):401–412.
- Blake MJ, Nowak TS, Jr, Holbrook NJ. 1990. In vivo hyperthermia induces expression of HSP70 mRNA in brain regions controlling the neuroendocrine response to stress. Brain Res Mol Brain Res. 8(1):89–92.
- Cedraz H, Gromboni JGG, Garcia AAP, Farias Filho RV, Souza TM, Oliveira ERd, Oliveira EBd, Nascimento CSd, Meneghetti C, Wenceslau AA. 2017. Heat stress induces expression of HSP genes in genetically divergent chickens. PLoS One. 12(10):e0186083
- Collin A, Berri C, Tesseraud S, Rodon FR, Skiba-Cassy S, Crochet S, Duclos MJ, Rideau N, Tona K, Buyse J, et al. 2007. Effects of thermal manipulation during early and late embryogenesis on thermotolerance and breast muscle characteristics in broiler chickens. Poult Sci. 86(5):795–800.
- Cramer T, Rosenberg T, Kisliouk T, Meiri N. 2019. Early-life epigenetic changes along the corticotropin-releasing hormone (CRH) gene influence resilience or vulnerability to heat stress later in life. Mol Psychiatry. 24(7):1013–1026.
- Cui Y-t, Liu B, Xie J, Xu P, Habte-Tsion H-M, Zhang Y-Y. 2014. The effect of emodin on cytotoxicity, apoptosis and antioxidant capacity in the hepatic cells of grass carp (Ctenopharyngodon idellus). Fish Shellfish Immunol. 38(1):74–79.
- Del Vesco A, Khatlab A, Goes E, Utsunomiya K, Vieira J, Neto AO, Gasparino E. 2017. Age-related oxidative stress and antioxidant capacity in heat-stressed broilers. Animal: Int J Anim Biosci. 11(10):1783–1790.
- Dou J, Montanholi YR, Wang Z, Li Z, Yu Y, Martell JE, Wang YJ, Wang Y. 2019. Corticosterone tissue-specific response in Sprague Dawley rats under acute heat stress. J Therm Biol. 81:12–19.
- E Q, Liu X, Liu Y, Liu W, Zuo J. 2013. Over-expression of GRP75 inhibits liver injury induced by oxidative damage. Acta Biochimica et Biophysica Sinica (Shanghai). 45(2):129–134.
- Gabriel J, Ferro J, Stefani R, Ferro M, Gomes S, Macari M. 1996. Effect of acute heat stress on heat shock protein 70 messenger RNA and on heat shock protein expression in the liver of broilers. Br Poult Sci. 37(2):443–449.
- Halevy O. 2020. Timing is everything-the high sensitivity of avian satellite cells to thermal conditions during embryonic and posthatch periods. Front Physiol. 11:235.
- Hao Y, Gu X. 2014. Effects of heat shock protein 90 expression on pectoralis major oxidation in broilers exposed to acute heat stress. Poult Sci. 93(11):2709–2717.
- Hensch TK. 2004. Critical period regulation. Annu Rev Neurosci. 27:549–579.
- Hu R, He Y, Arowolo MA, Wu S, He J. 2019. Polyphenols as potential attenuators of heat stress in poultry production. Antioxidants (Basel). 8(3):67.
- Kang D, Park J, Shim K. 2019. Heat treatment at an early age has effects on the resistance to chronic heat stress on broilers. Animals (Basel). 9(12):1022.
- Kang SW, Madkour M, Kuenzel WJ. 2017. Tissue-specific expression of DNA methyltransferases involved in early-life nutritional stress of chicken. Gallus gallus. Front Genet. 8:204.
- Kikusato M, Yoshida H, Furukawa K, Toyomizu M. 2015. Effect of heat stress-induced production of mitochondrial reactive oxygen species on NADPH oxidase and heme oxygenase-1 mRNA levels in avian muscle cells. J Therm Biol. 52:8–13.
- King EC, Pattwell SS, Glatt CE, Lee FS. 2014. Sensitive periods in fear learning and memory. Stress. 17(1):13–21.
- Kisliouk T, Meiri N. 2009. A critical role for dynamic changes in histone H3 methylation at the Bdnf promoter during postnatal thermotolerance acquisition. Eur J Neurosci. 30(10):1909–1922.
- Li E, Zhang Y. 2014. DNA methylation in mammals. Cold Spring Harb Perspect Biol. 6(5):a019133.
- Lin H, Decuypere E, Buyse J. 2006. Acute heat stress induces oxidative stress in broiler chickens. Comp Biochem Physiol A Mol Integr Physiol. 144(1):11–17.
- Lowman ZS, Edens FW, Ashwell CM, Nolin SJ. 2014. Actigen® influence on the gene expression of heat shock proteins in Ross 708 broiler chickens. Int J Poult Sci. 13(2):114–123.
- Madkour M, Aboelenin MM, Younis E, Mohamed MA, Hassan H, Alagawany M, Shourrap M. 2020. Hepatic acute-phase response, antioxidant biomarkers and DNA fragmentation of two rabbit breeds subjected to acute heat stress. Ital J Anim Sci. 19(1):1558–1566.
- Mitchell M, Sandercock D, MacLeod MG, Hunter RR. 2005. Thermoregulatory and metabolic heat production responses during acute heat stress in genetically improved broiler chickens. Int Poult Sci Forum Annual Meeting.
- Moraes V, Malheiros R, Bruggeman V, Collin A, Tona K, Van As P, Onagbesan OM, Buyse J, Decuypere E, Macari M. 2004. The effect of timing of thermal conditioning during incubation on embryo physiological parameters and its relationship to thermotolerance in adult broiler chickens. J Therm Biol. 29(1):55–61.
- Mujahid A, Sato K, Akiba Y, Toyomizu M. 2006. Acute heat stress stimulates mitochondrial superoxide production in broiler skeletal muscle, possibly via downregulation of uncoupling protein content. Poult Sci. 85(7):1259–1265.
- Mujahid A, Akiba Y, Warden CH, Toyomizu M. 2007. Sequential changes in superoxide production, anion carriers and substrate oxidation in skeletal muscle mitochondria of heat-stressed chickens. FEBS Lett. 581(18):3461–3467.
- Perini F, Cendron F, Rovelli G, Castellini C, Cassandro M, Lasagna E. 2020. Emerging genetic tools to investigate molecular pathways related to heat stress in chickens: a review. Animals. 11(1):46.
- Quinteiro-Filho WM, Ribeiro A, Ferraz-de-Paula V, Pinheiro ML, Sakai M, Sá LRM, Ferreira AJP, Palermo-Neto J. 2010. Heat stress impairs performance parameters, induces intestinal injury, and decreases macrophage activity in broiler chickens. Poult Sci. 89(9):1905–1914.
- Rajkumar U, Reddy M, Rao S, Radhika K, Shanmugam M. 2011. Evaluation of growth, carcass, immune response and stress parameters in naked neck chicken and their normal siblings under tropical winter and summer temperatures. Asian Australas J Anim Sci. 24(4):509–516.
- Rajkumar U, Vinoth A, Shanmugam M, Rajaravindra K, Rama Rao S. 2015. Effect of embryonic thermal exposure on heat shock proteins (HSPs) gene expression and serum T3 concentration in two broiler populations. Anim Biotechnol. 26(4):260–267.
- Rajkumar U, Vinoth A, Shanmugam M, Rajaravindra K, Rama Rao S. 2017. Effect of increased incubation temperature on Hsp 90 and 60 gene expressions in coloured broiler chickens. J Appl Anim Res. 45(1):298–303.
- Rimoldi S, Lasagna E, Sarti FM, Marelli SP, Cozzi MC, Bernardini G, Terova G. 2015. Expression profile of six stress-related genes and productive performances of fast and slow growing broiler strains reared under heat stress conditions. Meta Gene. 6:17–25.
- Saleh KM, Tarkhan AH, Al-Zghoul MB. 2020. Embryonic thermal manipulation affects the antioxidant response to post-hatch thermal exposure in broiler chickens. Animals (Basel). 10(1):126.
- Surai P. 2015. Antioxidant systems in poultry biology: heat shock proteins. J Sci. 5(12):1188–1222.
- Surai PF, Kochish II, Fisinin VI, Kidd MT. 2019. Antioxidant defence systems and oxidative stress in poultry biology: An update. Antioxidants (Basel). 8(7):235.
- Suri D, Vaidya VA. 2015. The adaptive and maladaptive continuum of stress responses - a hippocampal perspective. Rev Neurosci. 26(4):415–442.
- Tan G-Y, Yang L, Fu Y-Q, Feng J-H, Zhang M-H. 2010. Effects of different acute high ambient temperatures on function of hepatic mitochondrial respiration, antioxidative enzymes, and oxidative injury in broiler chickens. Poult Sci. 89(1):115–122.
- Tanizawa H, Shiraishi J-i, Kawakami S-I, Tsudzuki M, Bungo T. 2014. Effect of short-term thermal conditioning on physiological and behavioral responses to subsequent acute heat exposure in chicks. J Poult Sci. 51(1):80–86.
- Tona K, Onagbesan O, Bruggeman VCA, Berri C, Duclos MJ, Tesseraud S, Buyse J, Decuypere E, Yahav S. 2008. Effects of heat conditioning at d 16 to 18 of incubation or during early broiler rearing on embryo physiology, post-hatch growth performance and heat tolerance. Archiv Für Geflügelkunde Sonderheft. 72(2):75–83.
- Tzschentke B, Basta D. 2000. Development of hypothalamic neuronal thermosensitivity in birds during the perinatal period. J Therm Biol. 25(1-2):119–123.
- Tzschentke B, Basta D, Janke O, Maier I. 2004. Characteristics of early development of body functions and epigenetic adaptation to the environment in poultry: focused on development of central nervous mechanisms. Avian Poul Biolog Rev. 15(3):107–118.
- Ulrich-Lai YM, Herman JP. 2009. Neural regulation of endocrine and autonomic stress responses. Nat Rev Neurosci. 10(6):397–409.
- van Bodegom M, Homberg JR, Henckens MJAG. 2017. Modulation of the hypothalamic-pituitary-adrenal axis by early life stress exposure. Front Cell Neurosci. 11:87
- Vinoth A, Thirunalasundari T, Tharian JA, Shanmugam M, Rajkumar U. 2015. Effect of thermal manipulation during embryogenesis on liver heat shock protein expression in chronic heat stressed colored broiler chickens. J Therm Biol. 53:162–171.
- Vinoth A, Thirunalasundari T, Shanmugam M, Rajkumar U. 2016. Effect of early age thermal conditioning on expression of heat shock proteins in liver tissue and biochemical stress indicators in colored broiler chicken. Euro J Exp Bio (Basel). 6:53–63.
- Vinoth A, Thirunalasundari T, Shanmugam M, Uthrakumar A, Suji S, Rajkumar U. 2018. Evaluation of DNA methylation and mRNA expression of heat shock proteins in thermal manipulated chicken. Cell Stress Chaperones. 23(2):235–252.
- Wadhwa R, Taira K, Kaul SC. 2002. An Hsp70 family chaperone, mortalin/mthsp70/PBP74/Grp75: what, when, and where? Cell Stress Chaper. 7(3):309–316.
- Wan J, Oliver VF, Wang G, Zhu H, Zack DJ, Merbs SL, Qian J. 2015. Characterization of tissue-specific differential DNA methylation suggests distinct modes of positive and negative gene expression regulation. BMC Genomics. 16:49
- Wan Y, Ma C, Wei P, Fang Q, Guo X, Zhou B, Jiang R. 2017. Dynamic expression of HSP90B1 mRNA in the hypothalamus of two Chinese chicken breeds under heat stress and association analysis with a SNP in Huainan chickens. Czech J Anim Sci. 62(No. 2):82–87.
- Wang S, Edens F. 1998. Heat conditioning induces heat shock proteins in broiler chickens and turkey poults. Poult Sci. 77(11):1636–1645.
- Xie J, Tang L, Lu L, Zhang L, Lin X, Liu HC, Odle J, Luo X. 2015. Effects of acute and chronic heat stress on plasma metabolites, hormones and oxidant status in restrictedly fed broiler breeders. Poult Sci. 94(7):1635–1644.
- Yahav S, Collin A, Shinder D, Picard M. 2004. Thermal manipulations during broiler chick embryogenesis: effects of timing and temperature. Poult Sci. 83(12):1959–1963.
- Yahav S, Shinder D, Tanny J, Cohen S. 2005. Sensible heat loss: the broiler's paradox. World's. Poult Sci J. 61(3):419–434.
- Yahav S. 2009. Alleviating heat stress in domestic fowl: different strategies. World Poult Sci J. 65(4):719–732.
- Yang L, Tan G-Y, Fu Y-Q, Feng J-H, Zhang M-H. 2010. Effects of acute heat stress and subsequent stress removal on function of hepatic mitochondrial respiration, ROS production and lipid peroxidation in broiler chickens. Comp Biochem Physiol C Toxicol Pharmacol. 151(2):204–208.
- Yossifoff M, Kisliouk T, Meiri N. 2008. Dynamic changes in DNA methylation during thermal control establishment affect CREB binding to the brain-derived neurotrophic factor promoter . Eur J Neurosci. 28(11):2267–2277.
- Zaboli GR, Rahimi S, Shariatmadari F, Torshizi MAK, Baghbanzadeh A, Mehri M. 2017. Thermal manipulation during Pre and Post-Hatch on thermotolerance of male broiler chickens exposed to chronic heat stress. Poult Sci. 96(2):478–485.
- Zhang GH, Liu H, Wang JJ, Wang ZY. 2014. Effects of thermal stress on lipid peroxidation and antioxidant enzyme activities of the predatory mite, Neoseiulus cucumeris (Acari: Phytoseiidae). Exp Appl Acarol. 64(1):73–85.
- Zilaee M, Ferns GA, Ghayour-Mobarhan M. 2014. Heat shock proteins and cardiovascular disease. Adv Clin Chem. 64:73–115.
- Zulkifli I, Najafi P, Nurfarahin A, Soleimani A, Kumari S, Aryani AA, O'Reilly EL, Eckersall PD. 2014. Acute phase proteins, interleukin 6, and heat shock protein 70 in broiler chickens administered with corticosterone. Poult Sci. 93(12):3112–3118.