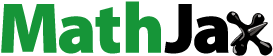
Abstract
An experiment was done to evaluate the effects of diet nutrients density on performance and egg quality in laying hens during the post-peak production phase of the first laying cycle under subtropical climate. A total of 768, 49-weeks-old Hy-Line-W36 laying hens were assigned in a completely randomised design with six treatments, eight replicates, and 16 birds each. Experimental treatments were given the diet with nutrients density recommended by the Hy-Line-W36 guide for their age and level of production (100%) or were given 92, 94, 96, 98, and 102% of that. During the experimental period, the average ambient temperature and humidity were 27.41 ± 2.54 °C and 35 ± 5%, respectively. By increasing diet nutrients density significantly improved egg production (EP), egg weight (EW), egg mass (EM), feed conversion ratio (FCR). Also, egg crude protein and yolk ether extract composition, egg special gravity and relative shell weight significantly increased as increased diet nutrient density. Average bird’s daily feed intake (FI), eggshell thickness, Haugh unit and solid percentage did not affect by diet nutrients density. By linear broken-line models, the diet nutrients density for optimised EP, EM, and FCR were estimated at 97.93, 97.57, and 100% of strain recommendation, respectively. It is concluded, laying hens during the post-peak production phase of the first laying cycle and under subtropical climate was not able to adjust FI with diet dilution. Nutrient requirements varied to what productive parameter was taken for optimisation. Optimum FCR was achieved when the diet was formulated to followed strain recommendations.
Hy-Line-W36 laying hens during the post-peak production phase of the first laying cycle and under subtropical climate cannot adjust their feed intake with dietary nutrients dilution.
HIGHLIGHTS
Introduction
The primary objective of poultry production is to achieve a desirable performance by considering the environmental issues related to intensive poultry operations (Rama Rao et al. Citation2014; Rehman et al. Citation2018). Since feed express the significant cost of production (Almeida et al. Citation2019), poultry producers often interested in feeding diets with low nutrients level to reduce feed cost (Alagawany et al. Citation2020), while it may not appear to supply sufficient energy and nutrients if birds could not adjust feed intake with diet dilution (Alagawany et al. Citation2014; dePersio et al. Citation2015; Alagawany et al. Citation2020). It is critical to adjust the diet nutrient density to the actual feed intake of the bird to ensure sufficient consumption of nutrients (Leeson and Summers Citation2009). Modern laying strains will presently have a small appetite and cannot accurately alter feed intakes according to dietary nutrients density (Jalal et al. Citation2007; dePersio et al. Citation2015). The genetic potential of highly efficient birds, such as the Hy-Line-W36, may be compromised by a diet designed for a bird that can eat more (mHy-Line Citation2016). Therefore, a higher density diet is required for a more efficient bird to achieve production potential. Against the above hypothesis, there were many reports assumed laying hens can regulate their feed intake to maintain energy and nutrients intake following requirements (Leeson et al. Citation2001; Wu et al. Citation2007). However, this ability may be affected by age and thermal conditions (Rama Rao et al. Citation2014).
The concept of phase feeding is based on the fact as birds get older, their feed intake increases, while egg mass output decreases (Saleh, Ahmed, et al. Citation2019). Therefore, laying hens should be provided sufficient nutrients by adjusting the diet density with feed intake in order to meet their requirements and to allow them to reach their genetic potential (Leeson and Summers Citation2009). On the other hand, the commercial poultry industry faces a significant challenge during summers with severe heat. High ambient temperature is very disruptive for layers; it reduces feed intake, egg production, egg weight, egg mass, and feed efficiency (Sterling et al. Citation2003; Lin et al. Citation2004; Mashaly et al. Citation2004; Saleh, Kirrella, et al. Citation2019). Under hot weather, improved production should be possible through modifications of diet composition to compensate for the low feed consumption (Renaudeau et al. Citation2012; Saleh, Eltantawy, et al. Citation2019). Furthermore, the majority of the studies have been conducted in temperate conditions. In the event that the requirement of nutrients depends on ambient temperature, too (Peguri and Coon Citation1991). The recommendations generated in temperate environments may not be suitable to apply laying hens reared in a hot climate (Rama Rao et al. Citation2014).
Given this background, the present study was conducted to evaluate the effect of dietary nutrients density on production performance and egg quality of Hy-Line-W36 laying hens during the post-peak production phase of the first laying cycle (49–60 weeks of age) under a subtropical climate. Additionally, we hypothesised that it is possible to estimate the ME and nutrients requirement of Hy-Line-W36 laying hens under subtropical climate.
Materials and methods
Feedstuffs analysis
Prior to the experiment, samples of the main ingredients, (corn: DM, 88.0%; CP, 7.5%; wheat bran: DM, 88.1%; CP, 14.8% and soybean meal: DM, 89.5%; CP, 42.0%) were analysed by the methods described (AOAC Citation2002) and (corn: ME, 3373 kcal/kg; dig Lys, 0.21%; dig Met, 0.15%; dig SAAs, 0.30%; dig. Thr, 0.23%, wheat bran: ME, 1300 kcal/kg; dig Lys, 0.43%; dig Met, 0.17%; dig SAAs, 0.39%; dig. Thr, 0.35%, and soybean meal: ME, 2420 kcal/kg; dig Lys, 2.25%; dig Met, 0.52%; dig SAAs, 1.03%; dig Thr, 1.39%) were measured by NIR through Evonik Co. (Evonik Nutrition& Care GmbH) agent in Tehran, Iran. These values were used for experimental diets formulation.
Birds, housing and diets
Considering that the aim of this study was to determine the effects of dietary nutrient density in a subtropical summer climate on layer performance, so the trial was conducted in a closed house with proper ventilation conditions during the summer season (July, August, and September), considered as oppressively hot months in the northeast of Iran. The experiment was done at the Poultry Research Farm, Ferdowsi University of Mashhad, Mashhad, Iran (59° 26′ 30′′ E longitude, 36° 25′ 18′′ N latitude, and Elevation 1083 m), by using a total of 768, 46-week-old Hy-Line-W36 laying hens. The birds were individually weighed and randomly assigned in a completely randomised design with six treatments, eight replicates, and 16 each. The birds were housed four per cage (40 cm × 45 cm wire-bottomed cage, corresponding to 450 cm2 per hen), and every four adjacent cages contained 16 birds served as an experimental unit. Each cage was equipped with a feeder and a nipple drinker to provide birds with free access to feed and water. The house temperature and relative humidity were recorded through the experimental period. The incandescent lights were used to provide 16 L:8 D cycle.
During the 3-week-adaptation period, birds fed an industry-type diet, daily feed intake, and egg production were recorded, which were 100 g/bird and 86%, respectively. Six experimental diets were formulated on a least-cost basis using corn, soybean meal, wheat bran, and soybean oil to give the daily metabolisable energy (ME), nutrients (CP, Ca, available Phosphorus, Na, dig Lys, dig SAAs, and dig Thr) corresponding to strain (mHy-Line Citation2016) recommendation for their age and level of production (100%) or were given 92, 94, 96, 98 or 102% of that amount (Table ). Birds were fed experimental diets for 12 weeks. All birds had free access to mash feed and water throughout the experiment.
Table 1. Ingredients and nutrients composition of experimental diets.
Production performance
All hens were weighed at the beginning and end of the experimental period. Egg production (number and weight) and mortality were recorded daily, whereas performance traits were calculated and compiled at every 28 days intervals (49–52, 53–56, 57–60 weeks). For egg quality traits, six eggs/replicate (48 eggs/treatment) from eggs laid during the three consecutive days at the end of each period (26–28 days) were randomly selected and transported to Egg Quality Laboratory in Ferdowsi University of Mashhad, Mashhad, Iran.
Daily energy and nutrient consumption
The average daily energy and nutrients intake were calculated by using feed consumption information and experimental diets analysed composition.
Statistical analysis
All data were analysed for normality using SAS 9.1 software through the Univariate plot normal procedure (SAS Citation2004). The data were analysed by using the General Linear Model procedure, with a one-way model and dietary nutrient density as independent variables. Orthogonal polynomials for linear and quadratic responses to diet nutrients density were calculated to explore the relationships between dietary nutrients level as independent variables and the respective traits as dependent variables. The dietary nutrients density for maximum response in performance variables, that is R2 was significant, were predicted using the single-slope broken-line regression model using the nonlinear modelling option in SAS with the dietary nutrients density as the independent variable (Robbins et al. Citation2006; Ghavi et al. Citation2020; Zarghi et al. Citation2020). The iterative procedure makes repeated estimates for coefficients and minimises residual error until the best-fit lines are achieved:
Where: X = Independent variable, R = Requirement, Y = Dependent variable, L = Theoretical maximum, I = 1 (if X < R) or I = 0 (if X > R), and U = Constant rate. The coefficient of determination (R2) was determined as follows: R2 = 1 − (Residual sum of squares/Corrected total sum of squares).
Results
The average ambient temperatures were 27.41 ± 2.54 °C with an average relative humidity of 35 ± 5% during the experimental period (Figure ).
Egg production performance
The results for egg production performance indices are presented in Table . There was a considerable positive reaction to increasing diet nutrients density on hen-day egg production (EP), egg mass (EM), and feed conversion ratio (FCR) either during any particular 28 days period or over the entire experiment (linear effect, p < .01) and egg weight during the first 28 days period and whole experimental period (linear effect, p < .05). The results showed, birds fed the diet containing 102% nutrient density as strain recommendation performed the highest EP and EM, which were 13.88% and 16.55% higher than those fed diet containing 92% nutrient density as strain recommendation, respectively. The feed conversion ratio was the lowest in the birds fed the diet with 100% nutrient density as strain recommendation, and it was improved 13.11 than that fed diet with 92% nutrient density. The feed intake (FI) was not affected (p > .05) by dietary nutrients density during the different phases and over the entire experiment.
Table 2. Effect of diet nutrients density on egg production, egg weight, and egg mass of laying hens during the post-peak production phase of the first laying cycle under subtropical summer conditionsa.
The effect of dietary nutrient density on live body weight is reported in Table . Diet nutrients density linearly effect (p < .05) live body weight at the end and bodyweight difference between the initial and end of the experimental period. The average live body weight at 60 weeks of age was increased by increasing diet nutrients density. The average live body weight was highest in the birds fed the diet with 102% nutrient density as strain recommendation, and it was absolutely 78 g higher than a fed diet with 92% nutrient density as strain recommendation (1711 g vs. 1633 g LBW).
Table 3. Effect of diet nutrients density on feed intake, feed efficiency, and body weight of laying hens during the post-peak production phase of the first laying cycle under subtropical summer conditionsa.
Significant linear responses due to increasing dietary ME and nutrients density is observed for economic performance (Table ). Egg income, feed cost, and economic benefit (EB) linearly effect (p < .001), by increasing diet ME and nutrients density for either during any particular 28 days period or over the entire experiment.
Table 4. Effect of diet nutrients density on economic performance of laying hens during the post-peak production phase of the first laying cycle under subtropical summer conditionsa.
Daily ME and nutrients intake
The daily consumption of ME, CP, Ca, available Phosphorus, Na, dig Lys, dig Met, dig SAAs, and dig Thr per bird/day was computed using the daily feed intake data, and the analysed diets ME and nutrients composition are shown in Table . The results showed by increasing dietary nutrients density significantly and linearly (p < .01) increased bird’s daily energy and nutrients intake either during any particular 28 days period or over the entire experiment. The birds that fed a diet with 96% or over nutrients density as strain recommendation showed daily ME and nutrients consumption equal to strain requirements recommended for the post-peak production phase of the first laying cycle (mHy-Line Citation2016).
Table 5. Calculated daily absolute intakes of ME and selected nutrients in the laying hens during the post-peak production phase of the first laying cycle under subtropical summer conditionsa.
Egg weight and shell quality
The effect of dietary nutrient density on egg quality traits sampled at the end of any particular 28 days period (52, 56, and 60 weeks of age) are shown in Table . As dietary nutrients density increased, yolk and albumen weight, egg special gravity, and egg relative shell weight linearly increased (p < .01). Shell thickness and haugh unit showed a non-significant response to increasing dietary nutrients concentration.
Table 6. Effect of diet nutrients density on egg quality of laying hens during the post-peak production phase of the first laying cycle under subtropical summer conditionsa.
The egg composition sampled at the end of the experimental period (at 60 weeks of age) is given in Table . Yolk ether extract and yolk, albumen, and whole crude protein composition linearly increased (p < .001) as dietary nutrients density increased. But yolk, albumen, and whole solid and whole ether extract showed non-significant responses to increasing dietary nutrients density (p > .05).
Table 7. Effect of diet nutrients density on egg composition of laying hens during the post-peak production phase of the first laying cycle under subtropical summer conditionsa.
Discussion
The thermo-neutral zone of the laying hens is generally between 18–25 °C. The environmental condition includes average ambient temperatures and average relative humidity in this experiment were 24–34 °C and 35 ± 5%, respectively. This result shows that the birds were reared under a subtropical climate, heat stress can profoundly affect the productivity of a flock. At environmental temperatures above 33 °C, high mortality and large production losses are readily evident, but at less extreme temperatures, heat stress is often overlooked as a cause for poor growth or subtle losses in egg production and shell quality (Lin et al. Citation2004; Renaudeau et al. Citation2012).
Egg production performance
With regards to the results obtained from this experiment, Hy-line-W36 laying hens were not able to express feed intake relative with distinction in dietary nutrients density during 49–60 weeks of age under subtropical conditions. But significant linear improvement due to an increase in dietary nutrients density as observed for EP, EM, and FCR (Table ). Breeding programs in layers for higher feed efficiency have been associated with reduced body size (Thiruvenkadan et al. Citation2010; Leenstra et al. Citation2016), this propelled to low feed consumption-ability (dePersio et al. Citation2015). Hence, present strains of laying hens have a limited gastrointestinal tract volume to increase feed intake adjusted with diet dilution (Leenstra et al. Citation2016). Against our and above results, some researchers (Harms et al. Citation2000; Wu et al. Citation2005; Wu et al. Citation2007; Rama Rao et al. Citation2014) reported, hens would directly alter their feed consumption in light of nutrient density increments or diminishes. Harms et al. (Citation2000) reported Hy-Line W-36 hens responded by eating significantly more feed that was low in energy and less feed that was high in energy. Usually, birds consume feed to meet their energy requirement (Golian and Maurice Citation1992). Feed intake decreased linearly as dietary energy increased (Veldkamp et al. Citation2005).
Feed consumption is particularly dependent on environmental temperature, body maintenance requirements and feed ME level (Sterling et al. Citation2003). Above the thermo-neutral zone, the feed consumption diminishes (Renaudeau et al. Citation2012). The decreased feed consumption and low laying rate are the adverse effects of heat stress (Sterling et al. Citation2003; Lin et al. Citation2004; Mashaly et al. Citation2004). On the other hand, at temperatures above the thermo-neutral zone, the bird has to expend energy to maintain normal body temperature and metabolic activities. This diverts nutrients away from egg production, resulting in performance loss. Therefore, it is important to rebalance the diet for nutrients according to the birds’ productivity demand and the observed feed intake (mHy-Line Citation2016). Our result in this experiment showed, as diet nutrients density increased, hen-day egg production and daily egg mass linearly increased from 73.63 to 83.85% and 45.67 to 53.23 g/bird, resulting in a 13.88 and 16.55% improvement of EP and EM, respectively. Similarly, increasing diet nutrients density linearly decreased FCR from 2.243 to 1.957, resulting in a 12.75% improvement in feed conversion ratio (Table ). According to our observation, Wu et al. (Citation2007) reported that increasing dietary energy and nutrient density led to improve FCR 9.9%. The statement by Leeson and Summers (Citation2009) that high dense diets can improve feed efficiency agrees with the results seen in our study (Leeson and Summers Citation2009). Leeson et al. (Citation2001) similarly reported a decline in nutrient density showed a decline in feed efficiency. Previous research showed that hens would linearly adjust their feed intake in response to diet nutrient density by eating significantly more feed that was low in energy and less feed that was high in energy.
Our observation in this experiment showed increased dietary nutrients density resulting in a 2.22% superior average egg weight during the whole of the experimental period (63.46 vs. 62.08 g/egg in the birds fed a diet with highest against lowest nutrients level). However, the dietary nutrients density effect on EW during the first 4 weeks of the experimental period (49–52 weeks of age) was more efficient than the third 4 weeks of the experimental period (57–60 weeks of age). According to our result, Wu et al. (Citation2007) reported increasing both diet energy and nutrients (amino acids, Ca, and available P) led to higher egg weight during the first phase of egg-laying in the eight commercial leghorn strains. Egg size can be influenced by the amount of energy, crude protein, amino acids, linoleic acid, and total fat in the diet and the intake of these nutrients by the hen (Leeson and Summers Citation2009). Levels of these nutrients can be manipulated at specific focuses in the lay cycle to egg weight increase, decrease, or keep steady. It is reported, laying hens tend to lay larger eggs when they are fed diets with high levels of protein (dePersio et al. Citation2015; Almeida et al. Citation2019), sulphur amino acids (Alagawany and Abou Kasem Citation2014; Akbari Moghaddam Kakhki et al. Citation2016b), lysine (Alagawany and Abou Kasem Citation2014; Akbari Moghaddam Kakhki et al. et al. Citation2016a), ME (Leeson and Summers Citation2009; dePersio et al. Citation2015), and the amount of ME that is harvested by the bird (Morris Citation2004; Wu et al. Citation2007). Leeson et al. (Citation2001) also saw a trend in reduced egg size when diet nutrient density was reduced.
Heat stress can depress egg weight (Mashaly et al. Citation2004). High environmental temperature above the thermo-neutral zone has a depressing effect on the bird’s feed intake. The result can be a shortfall in nutrients like protein (amino acids) and energy, which will decrease egg weight. It is common to see decreased egg size as a consequence of heat stress (Sterling et al. Citation2003). Appropriate adjustments in feed formulation to match the actual bird feed intake and mitigation of heat stress conditions can minimise this depression of egg size.
Our result in this experiment showed economic benefit (EB), egg income minus feed cost, increased linearly with increasing dietary energy and nutrient density (Table ). Because feed ingredient prices and egg prices often vary, there can be no fixed ideal dietary ME and nutrients density for optimal EB. Poultry producers may need to apply some economic feeding and management attention to determine the diets for optimal profits. While low energy and nutrients diet apparently are more affordable, if hens cannot alter their feed admission with dietary ME and nutrient density, it may not guarantee optimal egg production. It has been indicated that the increased efficiency of birds fed a high-density diet can offset the higher cost of feed (Roland et al. Citation1998).
Egg quality traits
Our results in this experiment indicate by increasing diet nutrients density yolk, and albumen weight linearly increased from 17.08 to 17.93 g and from 35.61 to 39.84 g, respectively (Table ). According to us, reported Models and Hisex® laying hens treatments, which feeds contained high crude protein levels, generated higher albumen weight compared with the other treatments (Almeida et al. Citation2019). Many studies have shown that significant increases in egg weight due to increasing dietary nutrient concentration (Sohail et al. Citation2003; Wu et al. Citation2005) is mostly due to increased yolk weight (Sell et al. Citation1987; Wu et al. Citation2005). High albumen weight is also linked to dietary energy level, favouring protein deposition (Morris Citation2004; Wu et al. Citation2007; Leeson and Summers Citation2009). Similarly, it was concluded that increasing dietary energy improved protein utilisation in broilers (Reginatto et al. Citation2000). Our result in this experiment showed, increasing dietary nutrients levels led to increasing bird’s energy and nutrients intake such as protein and essential amino acids (Table ). This agrees with the reported increasing bird amino acid intake led to significantly increasing albumen weight (Prochaska et al. Citation1996; Shafer et al. Citation1998; Novak et al. Citation2004). The increase of albumen weight might be partially attributed to the improved nutrient utilisation with increased dietary nutrients levels. Hens can use available exogenous fat as lipids for egg yolk formation (Sell et al. Citation1987). Hens can deposit dietary lipids into the egg yolk and change the composition of yolk lipids (Scheideler and Froning Citation1996).
Our results in this experiment shown, egg special gravity and relative shell weight linearly increased (p < .01) by increasing dietary nutrients density (Table ). Heat-stressed laying flocks often lay eggs with thinner, weaker eggshells because of an acid/base disturbance occurring in the blood as a result of panting (Lin et al. Citation2004; Renaudeau et al. Citation2012). Above the thermo-neutral zone, the efficiency of sensible heat loss mechanisms diminishes. At this point, the evaporation of water from the respiratory tract becomes the major heat loss mechanism of the bird (Lin et al. Citation2004; Renaudeau et al. Citation2012). As birds hyperventilate to lose body heat, there is excessive loss of CO2 gas from their lungs and blood. Lower CO2 in the blood causes blood pH to elevate or become more alkaline (Lin et al. Citation2004). The higher blood pH reduces the activity of the enzyme carbonic anhydrase, resulting in reduced calcium and carbonate ions transferred from blood to the shell gland (Scanes Citation2014), which may be responsible for the decreased shell thickness. Another contributing factor to thin eggshells is reduced intake of calcium and nutrients as feed consumption drops (Renaudeau et al. Citation2012). Therefore, closely monitor the feed consumption of the flock during subtropical weather. It is important to rebalance the diet for other critical nutrients, particularly amino acids, calcium, sodium and phosphorous, according to the birds’ productivity demand and the observed feed intake.
Dry matter of yolk, albumen, and the whole egg liquid were not influenced by dietary nutrient density. Whereas, yolk ether extract and crude protein contents of yolk, albumen, and whole egg significant and quadratic changed with increasing diet ME, and nutrient concentration (Table ). Our result in this experiment approved other research (Novak et al. Citation2004; Wu et al. Citation2007). By increasing dietary nutrients concentration, a greater proportion of nutrients will be utilised, the elevation of amino acid concentrations in plasma causes an increase in insulin secretion by the pancreas. Two functions of insulin are elevation in amino acid uptake and protein synthesis (Prochaska et al. Citation1996; Akbari Moghaddam Kakhki et al. Citation2016b).
Estimated ME and nutrient requirements
In this study, we investigated the diet nutrients density on egg performance, egg quality, and economic benefit traits in laying hens during the post-peak production phase of the first laying cycle under subtropical weather. A critical goal of this study was to estimate the ME and nutrients requirement of Hy-Line-W36 laying hens under subtropical conditions. The optimisation model was solved using the NLIN program SAS 9.1 procedure. Fitted broken-line plots for the egg performance traits as a function of diet nutrients density (% of strain recommendation) are shown in Figures . The break-points occurred at 97.62 ± 1.02%, p < .001, R2 = 0.58 for EP (Max 81.5% hen-day); 99.54 ± 2.92%, p < .011, R2 = 0.18 for EW (Max 63.67 g/Egg); 97.20 ± 0.99%, p < .001, R2 = 0.51 for EM (Max 51.66 g/bird/day), and 100 ± 1.01%, p < .001, R2 = 0.66 for FCR (Min 1.957) with linear broken line models.
Figure 2. Fitted broken-line plot of hen-day egg production of Hy-line-W36 laying hens during the post-peak production phase of the first laying cycle under subtropical summer conditions as a function of diet nutrients density (% of strain recommendation). The break point occurred at 97.62 ± 1.02, p < 0.001, R2 = 0.58 with linear broken line model.
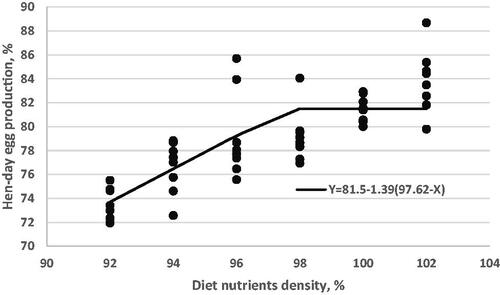
Figure 3. Fitted broken-line plot of average egg weight of Hy-line-W36 laying hens during the post-peak production phase of the first laying cycle under subtropical summer conditions as a function of diet nutrients density (% of strain recommendation). The break point occurred at 99.54 ± 2.92, p < 0.011, R2 = 0.18 with linear broken line model.
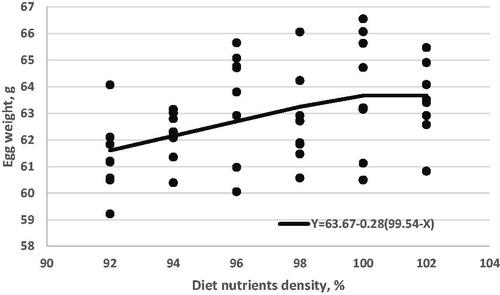
Figure 4. Fitted broken-line plot of egg mass of Hy-line-W36 laying hens during the post-peak production phase of the first laying cycle under subtropical summer conditions as a function of diet nutrients density (% of strain recommendation). The break point occurred at 97.20 ± 0.99, p < 0.001, R2 = 0.51 with linear broken line model.
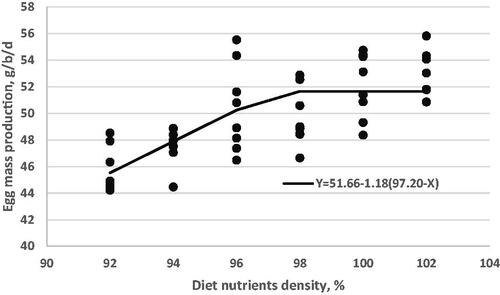
Figure 5. Fitted broken-line plot of feed conversion ratio of Hy-line-W36 laying hens during the post-peak production phase of the first laying cycle under subtropical summer conditions as a function of diet nutrients density (% of strain recommendation). The break point occurred at 100 ± 1.01, p < 0.001, R2 = 0.66 with linear broken line model.
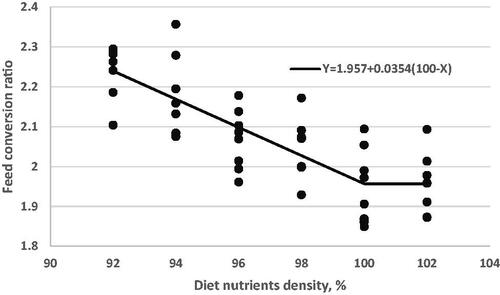
The summary of ME and nutrients (CP, Ca, available Phosphorus, Na, dig Lys, dig Met, dig SAAs, and dig Thr) requirements for the optimisation of EP, EW, EM, and FCR estimated by linear broken-line fit models are shown in Table . The present data suggest that optimum values of ME for Hy-Line-W36 laying hens reared in the subtropical weather during the post-peak production phase of the first laying cycle (49–60 weeks of age) was 286–293 kcal/kg diet. Corresponding values for optimum CP and Ca were 14.9–15.4 and 4.33–4.45 g/kg diet, respectively. Also available phosphorus, Na, dig Lys, dig SAAs, and dig Thr were 442–455, 177–182, 697–717, 589–606, and 481–495 mg/kg of diet, respectively. Overlay, our result has shown Hy-Line W-36 laying hens under subtropical conditions have a relatively limited capacity for increasing their feed intake according to diet dilution so that those not able to exhibit in feed intake relative to dietary nutrient dilution. Insufficient amino acid intake is the primary reason for productivity loss during subtropical weather. Therefore, it is important to rebalance the diet for other critical nutrients, particularly amino acids, calcium, sodium, and phosphorous according to the birds’ productivity demand and the observed feed intake.
Table 8. Estimated metabolisable energy and nutrients requirements of laying hens during the post-peak production phase of the first laying cycle under subtropical summer conditions by the linear broken-line fit model.
In agreement with our result, the scientific literature has demonstrated the beneficial effect of diets with a higher energy density and adjustments in the nutrients on production performance in laying hens (Jalal et al. Citation2007; dePersio et al. Citation2015). The modern commercial laying hens have been genetically improved for increased EP and feed efficiency. Thus, it is better to meet the nutrient requirements of birds to produce high performance (Fouad and El-Senousey Citation2014).
Conclusions
Hy-Line-W36 commercial laying hens during the post-peak production phase of the first laying cycle and under subtropical climate cannot adjust their feed intake with dietary nutrients dilution. During the post-peak production phase of the first laying cycle under subtropical climate, optimum EP, EM, FCR, and EW were observed with 97.6–100% of dietary nutrient density of strain recommendation. However, laying hens diet formulated with 97.6% of strain recommendation had the optimal egg production, but the higher dietary nutrients level of 100% was obtained for maximised feed efficiency.
Ethical approval
The experiment was conducted with the approval of the Animal Care Committee of the Ferdowsi University of Mashhad, Mashhad, Iran (Approval no: 318/441/2017).
Acknowledgements
The authors would like to thank the Poultry Research Station and Animal Nutrition Laboratory for their valuable help, critiques, and suggestions and the Iran branch of Evonik Co. for the feedstuffs amino acid analysis.
Disclosure statement
No potential conflict of interest was reported by the author(s).
Additional information
Funding
References
- Akbari Moghaddam Kakhki R, Golian A, Zarghi H. 2016a. Effect of dietary digestible lysine concentration on performance, egg quality, and blood metabolites in laying hens. J Appl Poultry Res. 25(4):506–517.
- Akbari Moghaddam Kakhki R, Golian A, Zarghi H. 2016b. Effect of digestible methionine + cystine concentration on performance, egg quality and blood metabolites in laying hens. Br Pult Sci. 57(3):403–414.
- Alagawany M, Abou Kasem DE. 2014. The combined effects of dietary lysine and methionine intake on productive performance, egg component yield, egg composition and nitrogen retention in Lohmann Brown hens. Egyptian J Nutrition and Feeds. 17(2):400–408.
- Alagawany M, El-Hindawy MM, Abd El-Hack ME, Arif M, El-Sayed SA. 2020. Influence of low-protein diet with different levels of amino acids on laying hen performance, quality and egg composition. An Acad Bras Ciênc. 92(1):e20180230.
- Alagawany M, El-Hindawy MM, Attia AI. 2014. Impact of protein and certain amino acids levels on performance of growing Japanese quails. Univers J Appl Sci. 2:105–110.
- Almeida TW, Silva AL, Saccomani AP, Muñoz JA, Silva RT, Franca NV, Faria DE, Faria Filho DE. 2019. Performance and egg quality of commercial laying hens fed diets formulated using non-linear programming. Braz J Poutl Sci. 21(3):1–10.
- AOAC. 2002. Official methods of analysis of AOAC international. 17 ed. Washington, DC, USA: Association of Official Analytical Chemists.
- dePersio S, Utterback PL, Utterback CW, Rochell SJ, O’Sullivan N, Bregendahl K, Arango J, Parsons CM, Koelkebeck KW. 2015. Effects of feeding diets varying in energy and nutrient density to Hy-Line W-36 laying hens on production performance and economics. Poult Sci. 94(2):195–206.
- Fouad AM, El-Senousey HK. 2014. Nutritional factors affecting abdominal fat deposition in poultry: a review. Asian-Australas J Anim Sci. 27(7):1057–1068.
- Ghavi S, Zarghi H, Golian A. 2020. Effect of dietary digestible sulphur amino acids level on growth performance, blood metabolites and liver functional enzymes of broilers 1–11 days of age. Ital J Anim Sci. 19(1):1439–1449.
- Golian A, Maurice DV. 1992. Dietary poultry fat and gastrointestinal transit time of feed and fat utilization in broiler chickens. Poult Sci. 71(8):1357–1363.
- Harms RH, Russell GB, Sloan DR. 2000. Performance of four strains of commercial layers with major changes in dietary energy. J Appl Poultry Res. 9(4):535–541.
- Jalal MA, Scheideler SE, Pierson EM. 2007. Strain response of laying hens to varying dietary energy levels with and without Avizyme supplementation. J Appl Poultry Res. 16(3):289–295.
- Leenstra F, Ten Napel J, Visscher J, Van Sambeek F. 2016. Layer breeding programmes in changing production environments: a historic perspective. Worlds Poult Sci J. 72(1):21–36.
- Leeson S, Summers JD. 2009. Commercial poultry nutrition. Nottingham (UK): Nottingham University Press.
- Leeson S, Summers JD, Caston LJ. 2001. Response of layers to low nutrient density diets. J Appl Poultry Res. 10(1):46–52.
- Lin H, Mertens K, Kemps B, Govaerts T, De Ketelaere B, De Baerdemaeker J, Decuypere E, Buyse J. 2004. New approach of testing the effect of heat stress on eggshell quality: mechanical and material properties of eggshell and membrane. Br Poult Sci. 45(4):476–482.
- Mashaly MM, Hendricks GL, Kalama MA, Gehad AE, Abbas AO, Patterson PH. 2004. Effect of heat stress on production parameters and immune responses of commercial laying hens. Poult Sci. 83(6):889–894.
- mHy-Line. 2016. Hy-line variety W-36 commercial management guide 2016. West Des Moines (IA): Hy-Line International.
- Morris T. 2004. Nutrition of chicks and layers. World Poultry Sci J. 60(1):5–18.
- Novak C, Yakout H, Scheideler S. 2004. The combined effects of dietary lysine and total sulfur amino acid level on egg production parameters and egg components in Dekalb Delta laying hens. Poultry Sci. 83(6):977–984.
- Peguri A, Coon C. 1991. Effect of temperature and dietary energy on layer performance. Poult Sci. 70(1):126–138.
- Prochaska JF, Carey JB, Shafer DJ. 1996. The effect of L-lysine intake on egg component yield and composition in laying hens. Poult Sci. 75(10):1268–1277.
- Rama Rao S, Ravindran V, Raju M, Srilatha T, Panda A. 2014. Effect of different concentrations of metabolisable energy and protein on performance of White Leghorn layers in a tropical climate. Br Poult Sci. 55(4):532–539.
- Reginatto MF, Ribeiro AML, Penz AM Jr, Kessler AM, Krabbe EL. 2000. Effect of energy, energy: protein ratio and growing phase on the performance and carcass composition of broilers. Rev Bras Cienc Avic. 2(3):229–237.
- Rehman ZU, Kamran J, Abd El-Hack ME, Alagawany M, Bhatti SA, Ahmad G, Saleem A, Ullah Z, Yameen RMK, Ding C. 2018. Influence of low-protein and low-amino acid diets with different sources of protease on performance, carcasses and nitrogen retention of broiler chickens. Anim Prod Sci. 58(9):1625–1631.
- Renaudeau D, Collin A, Yahav S, De Basilio V, Gourdine JL, Collier RJ. 2012. Adaptation to hot climate and strategies to alleviate heat stress in livestock production. Animal. 6(5):707–728.
- Robbins KR, Saxton AM, Southern LL. 2006. Estimation of nutrient requirements using broken-line regression analysis. J Anim Sci. 84:155–165.
- Roland DA, Bryant MM, Zhang JX, Roland DA, Rao SK, Self J. 1998. Econometric feeding and management. 1. Maximizing profits in Hy-Line W-36 hens by optimizing total amino acid intake and environmental temperature. J Appl Poultry Res. 7(4):403–411.
- Saleh A, Ahmed E, Ebeid T. 2019. The impact of phytoestrogen source supplementation on reproductive performance, plasma profile, yolk fatty acids and antioxidative status in aged laying hens. Reprod Dom Anim. 54(6):846–854.
- Saleh AA, Kirrella AA, Dawood MAO, Ebeid TA. 2019. Effect of dietary inclusion of cumin seed oil on the performance, egg quality, immune response and ovarian development in laying hens under high ambient temperature. J Anim Physiol Anim Nutr. 103(6):1810–1817.
- Saleh AA, Eltantawy SM, Gawish EM, Younis HH, Abd El-Moneim KA, Abd El A AE, Ebeid TA. 2019. Impact of dietary organic mineral supplementation on reproductive performance, egg quality characteristics, lipid oxidation, ovarian follicular development, and immune response in laying hens under high ambient temperature. Biol Trace Elem Res. 195:506–514.
- SAS. 2004. SAS/STAT users guide. Release 9.1. Cary (NC): SAS Institute.
- Scanes CG. 2014. Sturkie’s avian physiology. Amsterdam (The Netherlands): Elsevier Science.
- Scheideler SE, Froning GW. 1996. The combined influence of dietary flaxseed variety, level, form, and storage conditions on egg production and composition among vitamin E-supplemented hens. Poult Sci. 75(10):1221–1226.
- Sell J, Angel CR, Escribano F. 1987. Influence of supplemental fat on weights of eggs and yolks during early egg production. Poult Sci. 66(11):1807–1812.
- Shafer DJ, Carey JB, Prochaska JF, Sams AR. 1998. Dietary methionine intake effects on egg component yield, composition, functionality, and texture profile analysis. Poult Sci. 77(7):1056–1062.
- Sohail SS, Bryant MM, Roland DA. Sr 2003. Influence of dietary fat on economic returns of commercial Leghorns. J Appl Poultry Res. 12(3):356–361.
- Sterling K, Bell D, Pesti G, Aggrey S. 2003. Relationships among strain, performance, and environmental temperature in commercial laying hens. J Appl Poultry Res. 12(1):85–91.
- Thiruvenkadan AK, Panneerselvam S, Prabakaran R. 2010. Layer breeding strategies: an overview. World Poultry Sci J. 66(3):477–502.
- Veldkamp T, Kwakkel RP, Ferket PR, Verstegen MWA. 2005. Growth responses to dietary energy and lysine at high and low ambient temperature in male turkeys. Poult Sci. 84(2):273–282.
- Wu G, Bryant MM, Gunawardana P, Roland DA, Sr. 2007. Effect of nutrient density on performance, egg components, egg solids, egg quality, and profits in eight commercial leghorn strains during phase one. Poult Sci. 86(4):691–697.
- Wu G, Bryant MM, Voitle RA, Roland DA, Sr. 2005. Effect of dietary energy on performance and egg composition of Bovans White and Dekalb White hens during phase I. Poult Sci. 84(10):1610–1615.
- Zarghi H, Golian A, Nikbakhtzade M. 2020. Effect of dietary digestible lysine level on growth performance, blood metabolites and meat quality of broilers 23–38 days of age. J Anim Physiol Anim Nutr. 104(1):156–165.