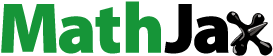
Abstract
Natural antioxidants play an important role in maintaining and improving poultry’s well-being, survival and productive and reproductive performance. Pyocyanin, a secondary blue redox-active metabolite, is one of these natural antioxidants that exert several biological activities to improve birds’ performance. This study estimated the effect of dietary supplementation with pyocyanin powder (PP) on broiler’s growth, carcase and serum properties, meat quality and microbial load activity. A total of 180 1-week-old unsexed chicks were randomly allocated into three groups. The first group served as a control (C) and fed the basal diet, while the second and third groups (P75 and P150) were fed the basal diet supplemented with 75.0 and 150.0 mg PP/kg, respectively. Results showed that overall body weight gain (BWG) was improved (p<.05) in P75 compared to P150 and control groups. Feed intake and feed conversion ratio (FCR) of chicks fed the diet containing PP levels were not altered during all experimental periods compared to the control. Dietary treatments did not affect all carcase traits, blood biochemical parameters and meat sensory characteristics at five weeks of age. Antioxidative status was improved by pyocyanin supplementation as serum malondialdehyde (MDA) was reduced while GST and GSH levels were elevated in P75 and P150 compared to the control. Dietary PP supplements increased (p<.05) the pH, lightness and redness of pectoralis major muscle and reduced (p<.05) the yellowness of the muscle and its contents of thiobarbituric acid and total volatile basic nitrogen. The PP addition showed antibacterial and antifungal activities against several pathogenic bacteria and mycotoxigenic fungi. It could be concluded that pyocyanin can be introduced as a natural, functional and phytogenic feed additive to boost broiler’s growth, improve their meat quality, produce nutritious meat products and reduce pathogenic bacteria without adverse impacts on their productivity.
This work investigated the effect of pyocyanin powder on broiler chickens.
The addition of pyocyanin at 75.0 mg/kg diet improved Body weight gain (BWG) and meat quality characteristics.
Pyocyanin exerted antibacterial and antifungal activity against several pathogenic bacteria.
Pyocyanin could be used as a natural and functional additive to poultry diets.
Highlights
Introduction
Verified data and findings have shown that phytogenic feed additives influence the development and health of animals and birds. These additives act as antioxidants that play an important role in the performance of animal wellbeing, survival, maintenance, productive and reproductive activities (Abd El-Hack et al. Citation2019; Alagawany et al. Citation2019). Dietary additives of phytogenic origin are materials obtained from plants used in poultry feeding in the poultry industry to increase productivity and reproductive efficiency and improve growth and immunity (Abd El-Hack, Abdelnour, Taha, et al. Citation2020). Herbal additives used in poultry feeds already have beneficial effects on poultry feeding, such as improved appetite and feed consumption, enhancement of endogenous digestive enzyme secretion, induction of immune response, antioxidant, antibacterial, antihelminthic and antiviral properties (Shewita and Taha Citation2019; Arif et al. Citation2019; Ibrahim et al. Citation2020). The beneficial effects of phytogenic additives on retention, intestinal microflora, gut health, minimised susceptibility to diseases, increased immune efficiency, and improved carcase yield and quality in poultry also have been shown by several reports (Gado et al. Citation2019; Abd El-Hack, Abdelnour, Taha, et al. Citation2020; Abd El-Hack, Alagawany, Shaheen, et al. Citation2020; Abdel-Moneim, Sabic, et al. Citation2020; Abo Ghanima, Elsadek, et al. Citation2020).
Pyocyanin is a secondary blue redox-active metabolite and a large family member of phenazines, tricyclic compounds. At the late stationary stage, they are secreted and provided the medium with a characteristic blue colour. It can be quickly extracted from the culture medium due to its solubility in chloroform. Pyocyanin ‘5-methyl-1-hydroxy phenazine’ can undergo a complex oxidation–reduction reaction (Ran et al. Citation2003). Many phenazine compounds isolated from bacteria have a broad range of antimicrobial activity, antitumor, antimalaria and antiparasitic activities (Laursen and Nielsen Citation2004). The antimicrobial activity of phenazine has been used as a biocontrol agent against certain food spoilage and pathogenic bacteria and fungi in sustainable agriculture (Kim Citation2000; Aunchaleeb et al. Citation2009).
These compounds are sufficient for inhibiting microbes (Giddens et al. Citation2003). Pyocyanin is isolated from Pseudomonas spp., it was used as antioxidants (Rada et al. Citation2013; Laxmi and Bhat Citation2016) and anticancer agents (Hassani et al. Citation2012). Pyocyanin has shown antibacterial and antifungal activity against many pathogenic bacteria, and mycotoxigenic fungi are also available for antioxidant and anticancer activity against cancer cell lines. As a result, for these activities, they can be used in food industries, such as food preservation and pharmaceutical applications (Marrez and Haitham Citation2020). We hypothesised that, with the aforementioned biological properties of pyocyanin, it can be used as a potential growth promoter in poultry industry. Therefore, the present study assessed the growth-promoting potential of dietary increasing levels of pyocyanin powder (PP) on performance, carcase characteristics, serum metabolites, meat quality and microbial enumeration of broiler chickens.
Materials and methods
Production, purification and characterisation of pyocyanin pigment
Soil samples were collected from the rhizosphere in Zagazig city, Sharika governorate, Egypt. The samples were transferred to the microbiological laboratory in sterilised bags. Pour plates technique was used for the isolation process: 10 g of soil samples were homogenised in 90 mL of sterilised peptone water to obtain 10−1 dilution, then serial dilutions were carried out from this dilution till 10−7. An aliquot of 0.1 mL of each dilution was spread on the surface of plate count agar (PCA) plates and incubated for three days at 37 °C (Barakat et al. Citation2015). The blue-greenish colonies were isolated and purified on cetrimide medium and preserved on cetrimide agar slants at 4 °C. The selected colonies were primarily identified by morphological and biochemical tests in Bergy Manual (Brenner et al. Citation2005; Uğur et al. Citation2012), then confirmed by MALDI-TOF spectroscopy.
Pseudomonas aeruginosa isolate was grown at King’s medium broth and incubated for 2–3 d at 35 °C for the extraction of pigment. The pigment’s colour shift to a blue-greenish indicates the pigment production (Mahon et al. Citation2007). An aliquot of 5 mL of bacterial culture was centrifuged at (10,000 rpm/15 min) and 3 mL of chloroform was applied to the supernatant and mixed until the blue colour was restored. The blue colour was converted to red-pink by adding 1 mL of 0.2N HCl, the absorbance of the acidic solution was measured at 520 nm and applied in the following equation, pyocyanin concentration (µg/mL)=Abs 520* 17.072 (Essar et al. Citation1990). The minimum volume of 0.1M NaOH was added to the pink solution to retain the blue-green colour and then chloroform was added again. The chloroform was evaporated and the PP was obtained (Raji El Feghali and Nawas Citation2018). The phytochemical profile of pyocyanin powder was identified by the GC-mass spectrum (Abdel-Shafi et al. Citation2019; Saad et al. Citation2020).
Microbial activity
Antibacterial activity of pyocyanin pigment
Well-diffusion assay was used to determine the pyocyanin antibacterial activity against three types of G+ bacteria (Staphylococcus aureus, Bacillus cereus and Listeria monocytogenes) and three types of G− bacteria (Salmonella enterica, Klebsiella pneumoniae and Escherichia coli) strains. The tested bacteria’s pure cultures were sub-cultured in Mueller Hinton broth (MHB) and incubated at 37 °C on a shaking incubator at 200 rpm for 24 h. Each culture was spread uniformly onto individual Mueller Hinton Agar (MHA) plates using the spread plate method. Wells (6 mm diameter) were punched in MHA with sterilised cork, then each well was loaded with 70 µL of pigment concentration of 10, 20, 30, 40 and 50 µg/mL dissolved in DMSO, the wells in MHA plates loaded with DMSO as a control. The MHA Plates were incubated for 24 h at 37 °C and pigment sensitivity was observed by diameter of inhibition zones around the wells (Saha et al. Citation2008). The minimum inhibition concentration (MIC) was the lowest concentration of pyocyanin that inhibited that bacterial growth and was estimated by microdilution broth; according to Murray et al. (Citation1995) the MIC defined as the lowest concentration of pyocyanin that inhibits the bacterial growth, and determined as follows, 100 µL of pyocyanin concentrations of 10, 20, 30, 40 and 50 μg mL−1 was added to test tubes containing 9 mL of MHB inoculated with 100 μL of the bacterial inoculum then incubated at 37 °C for 24 h. Turbidity (visible growth) was measured after 24 h at 600 nm using the JENWAY-England 6405 UV/VIS Spectrophotometer. The experiments were all done in triplicate. The control, was MHB tubes inoculated with bacterial inoculum. The minimum bactericidal concentration (MBC) was the lowest concentration of pyocyanin that killed the bacteria and was determined by pour plate method as follow 100 µL of MIC tubes was spared on new MHA plates then the bacterial growth was observed after incubation at 37 °C for 24 h.
Antifungal activity
Disc assay was used to determine the antifungal activity of pyocyanin concentrations (10, 20, 30, 40 and 50 µg/mL dissolved in DMSO) against six fungi strains (Aspergillus niger, Aspergillus flavus, Candida albicans, Candida gelberta, Penicillium solitum and Penicillium crustosum). An aliquot of 100 μL of the fungal suspension was spread on sabouraud dextrose agar (SDA, Candida). On potato dextrose agar (PDA, other fungi) plates, then discs 6 mm saturated with each concentration of pyocyanin were put on the sides of SDA for Candida and PDA plates for fungi. The SDA plates were incubated at 30 °C for 24 h and PDA plates were at 28 °C for 5 d, then the resultant inhibition zones were measured (mm). The MIC and MFC of pyocyanin were estimated by microdilution broth, and pour plate method, respectively (Moghadam et al. Citation2019).
Experimental design and diets
The present investigation was carried out at Poultry Research Farm, Poultry Department, Faculty of Agriculture, Zagazig University, Zagazig, Egypt. According to the Local Experimental Animal Care Committee, all experimental procedures were carried out and approved by the ethics of the Poultry Department's institutional committee, Faculty of Agriculture, Zagazig University, Zagazig, Egypt. A total of 180 Ross-308 unsexed broiler chicks one week old with an initial body weight of 130.22 ± 0.22 g were housed in a floor litter and used in a full randomised design trial with three treatments; 60 chicks in six replicates per group and replicate dimensions were 40 cm high × 100 cm width × 100 cm length. Dietary treatments were as follows: the first group served as a control (C) and fed the basal diet, while second and third groups (P75 and P150) were fed the basal diet supplemented with 75.0 and 150.0 mg PP/kg, respectively. Broilers were fed the basal diets in pellet form from 1 to 3 weeks (starter) and from 3 to 5 weeks (finisher). Feed and freshwater were provided ad-libitum during the experimental period. Chicks were housed under the same hygienic, rearing, and environmental conditions. The basal diets were formulated to meet the requirements of the broiler strain (Table ).
Table 1. Composition and chemical analysis of the basal diets as fed.
Growth performance and blood biochemical traits
Birds were weighed individually at weekly intervals, and the period and cumulative average daily feed intake (ADFI) were recorded. Body weight gain (BWG) and feed conversion ratio (FCR) were calculated from these data. For carcase evaluation, six birds with an average body weight around the mean treatment were randomly selected and slaughter weight (SW) was recorded. The carcases were weighed, and the liver, gizzard, heart and abdominal fat weights were registered and proportioned to the SW. Dressing percentage was calculated as weight of carcase plus the weight of giblets)/live body weight × 100 according to Abo Ghanima, Abd El-Hack, et al. (Citation2020). Blood samples were collected from sacrificed broiler chicks in clean, sterile tubes and left to coagulate. As mentioned in (Sitohy et al. Citation2013; Abdelnour, Swelum, et al. Citation2020; Abdelnour, El-Saadony, et al. Citation2020), serum samples were isolated from blood samples, then stored at −20 °C until analysis. In addition to the activity of alanine aminotransferase (ALT) and aspartate aminotransferase (AST), the serum content of urea, creatinine, total protein and albumin has been estimated using commercial diagnostic kits offered by Bio Diagnostic Co. (Giza, Egypt). From the following equation, globulin was calculated: Globulin = total protein - albumin.
The oxidative stress markers were estimated in the blood, including the lipid peroxidation malondialdehyde (MDA), glutathione peroxidase (GPx), glutathione-S-transferase (GST), glutathione reduced (GSH) using kits generated by Merck (KGaA, Darmstadt, Germany).
Meat quality
The 2-thiobarbituric acid test (TBA)
The determination of lipid oxidation in pectoralis muscle was tested according to Fernandez-Lopez et al. (Citation2005) The 2-thiobarbituric acid (TBA) numbers were determined as MDA/kg sample according to the following equation: TBA number (kg)=absorbance at 538 nm × 7.8. The pH of meat samples was measured using a digital pH metre.
Total volatile bases nitrogen (TVBN)
Total volatile bases nitrogen (TVBN) was calculated according to methods defined by Goulas and Kontominas (Citation2005). In brief, a total of 10 g meat samples was mixed with 50 mL of distilled water. The mixture was quantitatively transferred with 200 mL of distilled water into a 500 mL round bottom flask and distilled after 2 g of MgO and one drop of silicone to avoid foaming. A 250 mL Erlenmeyer flask containing 25 mL of 3% aqueous solution of boric acid, 0.04 mL of methyl red and methylene blue combined as markers for the titration of ammonia was used as the distillate receiver. The distillation continued until a final distillate volume of 125 mL was obtained. When the distilled absolute volatile basic nitrogen is rendered alkaline, the boric acid solution turned green (TVBN). This was titrated with 0.1N hydrochloric acid solutions that were aqueous. Total neutralisation was achieved when, after adding a further drop of hydrochloric acid, the distillate colour turned pink. From the amount (v) of hydrochloric acid added and its concentration (C), the quantity of TVBN in mg/100 g of meat was determined as follows: % mg TVBN= (V × C × 14 × 100)/10.
Colour evaluation
According to the tristimulus colour system, the colour of the samples was determined using a method defined by Francis and Clydesdale (Citation1975) (Hunter Lab Colour Flex, Ez, USA). In terms of lightness (L*), redness (a*) and yellowness colour (b*), was expressed. The standardisation was achieved by calibrating the system with a pink standard plate (L= +70.9, a= +22.4 and b= +8.2). An average of three readings from the same position was the Hunter value.
Sensory evaluation
All samples were sensory evaluated for their properties. The panel consisted by rating the above quality attributes using the following rating scale: 5 = Excellent, 4 = good, 3 = below good above fair, 2 = fair and 1 = very poor. Rating of 3 and 2 indicated that the products were of marginal quality, whereas the rating of 1 (poor) and below indicated that the consumers might not accept the product.
Microbial analysis
During feeding, dietary samples supplemented with pyocyanin concentrations (75 and 150 mg/kg diet) or without pyocyanin (control) were subjected to microbial analysis at an interval of (0, 7, 14 and 21 d). An amount of 25 g of dietary sample was homogenised with 225 mL of sterile saline peptone in a stomacher bag for 30 min to obtain 10−1 dilution. Serial dilutions were done till 10−7. An aliquot of 100 µL of each dilution was spread onto specific agar media plates. The total bacterial count (TBC) was enumerated at PCA after incubation at 30 °C for 48 h. The total count of yeasts and moulds (TYMC) was assessed after incubation at 25 °C for 5 d on Rose Bengal Chloramphenicol agar. Violet Red Bile Agar (Biolife, Italy) was used to enumerate coliforms after incubation at 37 °C for 24 h. E. coli count was assessed on Tryptone Bile Glucuronide Agar at 37 °C for 24 h (Harrigen and Mccance-Margart Citation1976; Szabo et al. Citation1986).
Caecal microbial community was also evaluated; 10 g of broiler caecum samples from each treatment were collected and transferred to a 250 mL Erlenmeyer flask containing 90 mL of sterile saline peptone solution and homogenised for 30 min. Decimal serial dilutions up to 10−7 were prepared. The microbial counts were assessed on specific media (El-Saadony et al. Citation2020; Reda et al. Citation2020; Sheiha et al. Citation2020). The TBC was enumerated on PCA. Violet Red Bile Agar and Tryptone Bile Glucuronide Agar were used for counting coliform and E. coli after incubation at 37 °C for 24 h, respectively (Harrigen and Mccance-Margart Citation1976; Szabo et al. Citation1986). Black colonies on S.S. agar indicate Salmonella spp., found and the count was assessed as per (Edwards and Hilderbran Citation1976). Yeasts and moulds were enumerated by using Rose Bengal Chloramphenicol agar medium at 28 °C for 72 h (Kurtzman and Fell Citation1984). Lactic acid bacteria were counted using MRS- medium (DeMan, Rogosa-Sharpe, Oxoid, CM 361) (Argyri et al. Citation2013). The microbial counts were converted into log numbers (Ashour, El-Hack, et al. Citation2020; Elbaz et al. Citation2021; Reda et al. Citation2021).
Histological examination
Collected samples were fixed in neutral buffered formalin (10%) and managed via the routine paraffin embedding method. Briefly, tissues specimens were dehydrated in ascending grade of ethyl alcohol, cleared in two changes of xylol, embedded in paraffin blocks, microtomed into 4 μm thick sections using a microtome (Leica RM 2155, England), and stained with haematoxylin and eosin according to Suvarna et al. (Citation2018). Representative photomicrographs were captured by a digital camera (Leica EC3, Leica, Germany) connected to a microscope (Leica DM500) (Abdel-Moneim, Elbaz, et al. Citation2020).
Statistical analysis
Data were subjected to the one-way analysis of variance using the General Linear Models (GLMs) procedure of the SPSS (Citation2008). The statistical model was as follows:
where Yij = observation, μ = overall mean, Pi = a fixed effect of PP dietary supplementation, and £ijk = experimental error. Using the Student–Newman–Keuls test, the statistical significant differences between means were determined at p<.05.
Results and discussion
Isolation and identification of bacterial isolate from soil
Fifteen bacterial isolates were obtained on PCA medium; one isolate was produced blue-greenish pigment. This isolate was purified. The purified isolate was gram-negative, short rods, and non-spore-forming under a light microscope. Results showed that this bacterium resembles Pseudomonas species. Based on Bergey’s Manual recommended tests (Brenner et al. Citation2005), the comparison process revealed the examined isolate could be defined as P. aeruginosa. This identification was confirmed by MALDI TOF mass spectroscopy according to Mahon et al. (Citation2007). The recorded results indicated that the selected bacteria were 98% similar to several Pseudomonas spp., mainly P. aeruginosa DSM 50071 T HAM (Cunningham and Patel Citation2013). Thus, the local screened bacterial isolate P. aeruginosa AS6 is similar to P. aeruginosa DSM 50071 T HAM. The concentration of isolated pyocyanin was 179.65 µg/mL based on the absorbance at 520 nm
GC-MS analysis of pyocyanin
The phytochemical components identified from the PP using GC-MS analysis were valeric acid, p-Allylphenol, 3, 3′, 4′, 7-Tetramethoxyflavone, Kaempferol, Flavidin- diacetate, Phenol, 2, 4 bis (1,1dimethylethyl) 3, 7, 11,15 Tetramethyl 2 hexadecen1ol, Phytol, 1 Propene1, 2, 3 tricarboxylic acid, tributylester, 5 Hydroxy1,4 dimethoxy anthraquinone and Eicosamethylcy clodecasiloxane along with other minor phytoconstituents (Figure , Table ). Several recent studies have suggested that many of these phytochemical components exert several biological activities including antitumor, antinociceptive, antioxidant, anti-inflammatory, immune-stimulating, antifungal and antibacterial properties (Islam et al. Citation2018; Wang et al. Citation2018; Marrez and Haitham Citation2020). These biological effects of pyocyanin components might explain its beneficial impacts on broilers' performance, meat quality and physiological responses.
Table 2. List of phytochemicals identified from pyocyanin powder using GC-MS analysis.
Antimicrobial activity of pyocyanin isolated from P. aeruginosa AS6
Based on the obtained results, MHA plates loaded with DMSO (control) did not show antimicrobial activity. Pyocyanin isolated from P. aeruginosa AS6 strain has more antibacterial activity than antifungal activity with a 40% relative increase (Table ). The IDZs against bacterial strains were in the range of (13–32 mm). However, the IDZs in tested fungi in the range of (10–24 mm). The IDZs were increased (p<.05) in a concentration-dependent manner. S. aureus and K. pneumonia were the most sensitive G+. G − bacteria to pyocyanin concentration (50 µg/mL), i.e. 32 and 25 mm, respectively. Nevertheless, L. monocytogenes and S. enterica were the most resistant G+, and G − bacteria to pyocyanin concentration (50 µg/mL) with IDZs 29, and 22 mm, respectively. On the other hand, Candida species were more vulnerable than fungal species, with 30% relative increase in IDZs. The most resistant fungi to pyocyanin concentration (50 µg/mL) were A. niger with 17 mm. Pyocyanin isolated from P. aeruginosa AS6 strain remarkably inhibited (p<.05) the bacterial growth in the range of MIC (6–9 µg/mL), and no bacterial growth (MBC) in the range of 10–18 µg/mL. The MIC range (9–18 µg/mL) were inhibited the fungal growth while MFC range (18–36 µg/mL) achieve no fungal growth (Table ). Several studies were reported the antimicrobial activity of pyocyanin, where Saha et al. (Citation2008), El-Shouny et al. (Citation2011) and Marrez and Haitham (Citation2020) reported that the antimicrobial activity of P. aeruginosa strains because of its secondary metabolites pyocyanin. Pyocyanin isolated from P. aeruginosa 4B strain showed antibacterial activity against pathogenic bacteria i.e. G + bacteria (L. monocytogenes and B. cereus) and G − bacteria (Salmonella paratyphi, E. coli and K. pneumonia). Also, Rahman et al. (Citation2009) found that pyocyanin extracted from P. aeruginosa DSO-19 has antibacterial activity against S. aureus, Staphylococcus epidermis, Bacillus subtilis and Micrococcus luteus. Moreover, Sudhakar et al. (Citation2013) found that pigment isolated from P. aeruginosa SU1 has the maximum activity against E. coli, S. aureus, Proteus sp. and Klebsiella spp. Pyocyanin exhibited the highest activity against C. albicans and B. cereus with average inhibition zones diameters of 26 and 14 mm, respectively, and also found that pyocyanin revealed a weak antibacterial activity was against E. coli (11 mm), Proteus vulgaris (10 mm) and Proteus mirabilis (9 mm), with MIC values in the range of 16–64 μg mL−1 (Dahah et al. Citation2016).
Table 3. Antimicrobial activity of pyocyanin presented by inhibition zones diameter (IDZ) (mm).
On the side of pyocyanin’s antifungal activity, it was found that pyocyanin isolated from several P. aeruginosa species has antifungal activity against Aspergillus and Candida spp., i.e. Aspergillus fumigatus, A. niger and C. albicans (Kerr Citation1994; Kerr et al. 1999). There was clinical evidence that pyocyanin suppressed the growth of different C. albicans in patients with lung infection (Pal and Revathi Citation1998; Kaleli et al. Citation2007), and other diseases (Marrez and Haitham Citation2020). Sudhakar et al. (Citation2013) reported that pyocyanin separated from P. aeruginosa WS1 encountered phytopathogens with MIC value of 64 μg mL−1 against A. flavus and Aspergillus fumigates, and 128 μg mL−1 against Candida species.
Growth performance
The effects of supplementation with pyocyanin on broiler chicks’ growth efficiency are shown in Table . No statistical differences were found in BWG due to treatments during the starter and finisher periods, while the overall BWG was improved (p<.05) in P75 and P150 compared to the control one. ADFI and FCR were not affected by the dietary inclusion of pyocyanin. The best values of BWG and FCR were observed in P75 group. The increase in BWG may be due to the biological activities of certain pyocyanin additive compounds that enhance digestion, absorption and intestinal function. Additionally, the antioxidant, anti-inflammatory, immune-stimulating, antifungal and antibacterial properties of pyocyanin and its derivatives (Islam et al. Citation2018; Wang et al. Citation2018; Marrez and Haitham Citation2020) might explain the improvement in broilers’ growth. These findings can be used as a basis for food industries, such as food preservation and pharmaceutical applications (Marrez and Haitham Citation2020). Several studies have reported the beneficial effects of phytogenic additives on growth efficiency, retention of nutrients, gut health, intestinal microflora, decreased disease susceptibility, improved immune function and improved poultry carcase yield and quality (Abd El-Moneim et al. Citation2019; Alagawany et al. Citation2019; Khafaga et al. Citation2019; Batiha et al. Citation2020; Abo Ghanima, Elsadek, et al. Citation2020; Abo Ghanima, Bin-Jumah, et al. Citation2020; Abdel-Moneim, Shehata, Mohamed, et al. Citation2021). Marzoni et al. (Citation2014) stated that no effects on broiler chickens’ growth efficiency were achieved by supplementing a mixture of natural antioxidants. However, Abdel-Moneim, Shehata, Mohamed, et al. (Citation2021) revealed that dietary inclusion of Spirulina platensis, a source of pyocyanin, increased BWG but did not affect FI or FCR. Contrarily, Elbaz et al. (Citation2021) stated that phytogenic additives such as garlic powder can improve FI and FCR of broiler chickens.
Table 4. Effect of dietary supplementation of pyocyanin on growth performance parameters of broiler chicks.
Carcase characteristics
Table presents the effects of dietary supplementation with PP on carcase traits at the end of the experimental period. All studied carcase traits were not statistically different compared to the control birds. The examined lymphoid organs were not statistically different as well. Results showed that dietary treatment affected the intestinal length, where broilers in P75 group recorded the highest length followed by those in P150 group compared to the control birds. In agreement with our results, Marzoni et al. (Citation2014) proved that supplementing a mixture of natural antioxidants did not affect the carcase yield of broilers. Çabuk et al. (Citation2006) also reported insignificant differences in broilers' growth and internal organ weight fed a mixture of herbs. The numerical reduction in abdominal fat percentage may be attributed to pyocyanin derivatives' antioxidant properties, which decrease the activity of the cytosolic malic enzyme leading to depressed abdominal fat deposition (Ashour, Bin-Jumah, et al. Citation2020; Abdel-Moneim, Shehata, Khidr, et al. Citation2021).
Table 5. Effect of dietary supplementation of pyocyanin carcase characteristics of broiler chicks.
Blood biochemical parameters
The effect of pyocyanin supplementation on some serum biochemical parameters of broiler chicks is presented in Table . The changes in the levels of serum content of urea, creatinine, total protein and albumin and the activity of ALT and AST in broiler chicks in P75 and P150 groups were slight and insignificant when compared to the control group. Pyocyanin supplementation enhanced the antioxidative status of broiler chickens (Table ). The level of lipid peroxidation (MDA) was reduced in P75 and P150 groups compared to the control. The activity of GST and level of GSH were elevated in treated groups compared to the untreated one. GPx activity was not altered among experimental groups. Blood biochemical parameters are generally correlated to the health status of birds and are good indices of the nutritional, physiological and pathological status of the birds. The lack of differences in these biomarkers in this study indicates the health benefits of pyocyanin. Moreover, the antioxidant role of pyocyanin was noticed in the results of this study. These findings are consistent with several earlier studies which documented the antioxidant properties of pyocanin and its phytochemical components such as phytol, phenazine, valeric acid and kaempferol (Islam et al. Citation2018; Wang et al. Citation2018; Marrez and Haitham Citation2020).
Table 6. Effect of dietary supplementation of pyocyanin on serum biochemical parameters of broiler chicks.
Table 7. Effect of dietary supplementation of pyocyanin on serum oxidative stress markers of broiler chicks.
Meat quality criteria
PH
The pH directly affects meat quality characteristics, such as colour, tenderness, water holding capacity and shelf life. The pH of broiler meat is a feature of glycogen in the muscle before slaughter and glycogen's conversion rate after slaughter into lactic acid (Nasir et al. Citation2017). The chicken meat colour with a pH below 5.8 was pale, while the colour of the chicken meat with a higher pH was too dark and a great danger to human health. So, for poultry, the ideal pH is between 5.8 and 6.3 (Pearson and Gillette Citation1996). The findings obtained in Table showed that the mean pH values for the chicken breast analysed were 5.37, 5.63 and 6.00 and that there were no significant disparities between the pH values of the different levels.
Table 8. Effect of dietary supplementation of pyocyanin on pH, TBA, TVBN and colour of chicken meat samples.
TBARS
A significant factor for restricting the shelf-life of meat products is lipid oxidation. The TBARS values reflect the lipid oxidation content and are primarily used as an important indicator for evaluating meat products’ quality due to its relatively simple measurement (Zhao et al. Citation2019). As shown in Table , with rising pyocyanin concentration, TBARS values decreased in all samples. Pyocyanin's strong inhibition effect on the change in TBARS value could be due to its antioxidant activity.
TVBN
TVBN, which consists mainly of dimethylamine (DMA), trimethylamine (TMA), ammonia and other compounds, is primarily due to its activity protein-degrading microbial enzymes and non-protein nitrogen compounds (Balamatsia et al. Citation2007; Yi et al. Citation2011). TVBN values of all different levels were shown in Table . TVBN values of all samples decreased with increasing concentration of Pyrocyanin levels, which had not exceeded the limitation (20mg/100 g of meat) of the Egyptian standard. The decrease in TVBN value in level 3 might be attributed to the reduced protein breakdown due to microbial strains and proteolytic enzymes’ activity.
Meat colour
Meat colour is an important assessment criterion. Colour or visual appearance is undoubtedly one of the most important sensory characteristics that affect consumers’ acceptance of meat and meat products (Adeyemi and Sazili Citation2014). The effect of the addition of pyocyanin on the colour of broilers is shown in Table . The findings indicate an increase in L* values with an increase in pyocyanin levels. With increased concentration, the a* value of the samples increased. The increase in red colour intensity may be due to increased concentration due to the absence of fat oxidation and colour oxidation. The key factors leading to changes in the value of b* are increased lipid oxidation and the formation of MetMb (Xiong Citation2000). The primary cause of deterioration in meat quality during storage, including undesirable changes in colour parameters (L*, a* and b*) and sensory properties, are compounds developed during the oxidative degradation of lipids (Nam et al. Citation2001; Gok et al. Citation2008).
Sensory evaluation
The chicken samples under investigation were evaluated for sensory characters (tenderness and juiciness, taste and aroma) following the addition of three levels of pyocyanin. The mean of scores is presented in Table . The addition of pyocyanin did not have a noticeable adverse impact on the tenderness, juiciness, taste and aroma of the samples for all samples at the different levels used. All samples showed a high acceptability score for similar sensory attributes. All samples typically scored well for all sensory parameters measured. These findings demonstrate that these natural functional ingredients can be integrated into poultry products without adversely affecting the quality of products that produce nutritious meat products. Functional advantages can offer customers added value (Bech-Larsen and Scholderer Citation2007).
Table 9. Effect of dietary supplementation of pyocyanin on sensory characteristics of chicken meat samples.
Impact of pyocyanin on microbial count
Feed samples
Figure showed the effect of PP addition at two concentrations (75 and 150 mg) on the microbial count in diet during the feeding period, especially (TBC, total yeasts and moulds count (TYMC), coliform and E. coli count). Generally, the microbial count decreased (p<.05) with pyocyanin supplementations in P75 and P150 groups. However, the microbial count increased during the feeding period. P150 has relatively decreased the TBC by 21%, total yeasts and moulds by 30%, total coliform by 35%, and E. coli by 40% compared to control. These results agree with the results of Makarand et al. (Citation2007) who recorded an antimicrobial activity of the pyocyanin against B. subtilis, C. albicans and E. coli. However, others demonstrated that phenazine antibiotics’ activity is concentration dependence; therefore, when the concentration of phenazine increased, the biological activity would be increased (Stephen Citation1981). Sudhakar et al. (Citation2013) also stated that pyocyanin isolated from P. aeruginosa SU1 has the highest antibacterial activity against E. coli. The antimicrobial activity of pyocyanin may be attributable to the effect of pyocyanin’s antimicrobial active components, including phenazine. Compounds also demonstrated multiple phenazines for anti-cancer, antimalaria and antiparasitic activities (Brisbane et al. Citation1987 ; Laursen and Nielsen Citation2004).
Figure 2. Effects of dietary inclusion of pyocyanin (C: control, P75: 75 mg/kg and P150: 150 mg/kg) on total bacterial count, total yeast and mould count, coliform populations and Escherichia coli in the basal diet during feeding period of 0, 7, 14 and 21 d of birds. Different lowercase letters indicate significant differences between treatments.
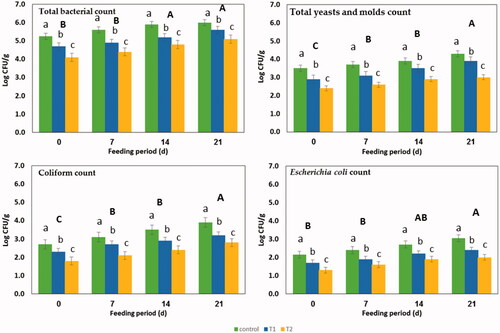
Caecal samples
By increasing the concentration of dietary pyocyanin, the microbial load in broiler caecum has enhanced TBC, total yeasts and moulds, Enterococcus spp., Coliform, E. coli and Salmonella spp. were decreased, while lactobacillus spp. count was increased (Table ). The addition of pyocyanin at 150 mg/kg prevented Salmonella spp. growth in the broiler caecum. Moreover, 75 and 150 mg pyocyanin/kg diet raised broiler caecum lactic acid bacteria count by 40% about control. The pyocyanin concentration of 150 mg/kg was more effective than the 75 mg/kg concentration. There were reductions in the overall microbial count, especially pathogenic bacteria, from the obtained results (Table ). The antimicrobial activity of pyocyanin might attributed to the fact that this pigment has developed as secondary metabolites to protect microorganisms from the harmful effect of visible and near-ultraviolet ran light rays.
Table 10. Effect of dietary supplementation of pyocyanin on caecal microbiota (Log CFU/mL) of broiler chicks.
Moreover, the antimicrobial activity of the isolated pigment against various microorganisms, e.g. C. albicans and A. fumigatus has been reported (Kerr et al. Citation1999). The author attributed this effect to phenazine and its derivatives. Phenazine is also known as tubermycin B because of its antibiotic activity against Mycobacterium tuberculosis, which causes pneumonia in susceptible patient populations and frequently fatal infections (Allen et al. Citation2005 ).
Histological examination
Ileum
The positive impacts of dietary supplementation of pyocyanin on ileal histomorphology are shown in Table . Birds fed pyocyanin in their diets had higher (p=.024) values of villus height (VH) than the untreated group. However, crypt depth (CD) and VH: CD ratio were not significantly influenced. These observations are consistent with our findings on growth performance.
Table 11. Effect of dietary supplementation of pyocyanin on ileum histomorphology of broiler chicks.
Liver
No histological alterations were noticed in control birds and those treated with pyocyanin, as normal central vein and portal area with normal hepatic parenchyma were observed (Figure ). Despite this normal hepatic structure, slight mononuclear cell permeation and dilatation of hepatoportal blood vessel in C group and slight congestion of hepatoportal blood vessel in P150 group were observed. These observations indicate that dietary levels of pyocyanin did not affect the normal structure of the hepatic parenchyma and did not exert hepatotoxicity impact.
Figure 3. (a) Effect of dietary supplementation of pyocyanin on ileum histomorphology of broiler chickens (H&E × 100). C: control group, P75: 75 mg pyocyanin/kg diet and P150: 150 mg pyocyanin/kg diet. (b) Liver of broiler chickens as affected by dietary supplementation of pyocyanin (H&E × 400) showing normal hepatic parenchyma, note the normal hepatocytes and blood sinusoids with slight dilatation of hepatoportal blood vessel in C (black arrow head), congestion of hepatoportal blood vessel in P150 (blue arrow head), blood sinusoids showing mononuclear cells permeation in C (black arrows). C: control group, P75: 75 mg pyocyanin/kg diet and P150: 150 mg pyocyanin/kg diet. (c) Spleen of broiler chickens as affected by dietary supplementation of pyocyanin (H&E × 200) showing normal lymphoid follicles with normal white and red bulbs and with slight vacuolation in C (arrows). C: control group, P75: 75 mg pyocyanin/kg diet and P150: 150 mg pyocyanin/kg diet.
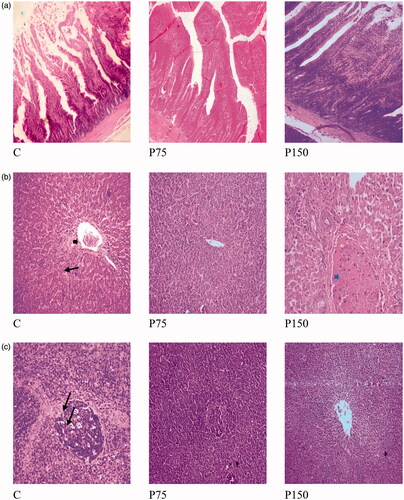
Spleen
Normal histological structure of spleen lymphoid follicles with normal white and red bulbs was noticed in all experimental groups with slight vacuolation in spleen tissue of control group (Figure ); suggesting the absence of the cytotoxic effect of pyocyanin at the studied levels.
Conclusions
Dietary supplementation of pyocyanin enhanced the growth performance and carcase traits of broiler chickens. Pyocyanin exerted antibacterial, antifungal, and antioxidant properties when supplemented to broilers’ diets, particularly at 75 mg/kg. The dietary inclusion of pyocyanin diminished caecal pathogenic bacteria. Besides, pyocyanin is a healthy and economic way to enhance chicken meat products' technical and sensory properties. However, further investigations are needed to examine more dietary levels of pyocyanin with more attention to the potentially toxic effects of pyocyanin when added to broilers' diets at higher levels.
Ethical approval
The present investigation was carried out at Poultry Research Farm, Poultry Department, Faculty of Agriculture, Zagazig University, Zagazig, Egypt. According to the Local Experimental Animal Care Committee, all experimental procedures were carried out and approved by the ethics of the Poultry Department's institutional committee, Faculty of Agriculture, Zagazig University, Zagazig, Egypt.
Author contributions
Conceptualisation and methodology, E.A.A., A.E.A., M.T.E-S., A.O.O., E.T.A.S.-A. and M.E.A.E.-H. Data curation, M.E.A.E.-H., E.A.A. and A.O.O. Writing – original draft preparation, E.A.A., M.T.E-S., A.O.O., A.E.T. and M.E.A.E.-H. Writing – review and editing, M.E.A.E.-H., N.M.A., M.E.S., R.M.F. and B.A.A. All authors have read and agreed to the published version of the manuscript.
Institutional review board statement
All experimental procedures for this study were conducted following the Local Experimental Animal Care Committee and subsequently approved by the Institutional Ethics Committee, Department of Poultry, Faculty of Agriculture, Zagazig University, Zagazig, Egypt (ZU-IACUC/2/F/94/2018).
Acknowledgments
Authors express their thanks to their respective institutions.
Disclosure statement
The authors declare no conflict of interest.
Additional information
Funding
References
- Abd El-Hack ME, Abdelnour SA, Abd El-Moneim AE, Arif M, Khafaga A, Shaheen H, Samak D, Swelum AA. 2019. Putative impacts of phytogenic additives to ameliorate lead toxicity in animal feed. Environ Sci Pollut Res. 26:23209–23218.
- Abd El-Hack ME, Abdelnour SA, Taha AE, Khafaga AF, Arif M, Ayasan T, Swelum AA, Abukhalil MH, Alkahtani S, Aleya L, et al. 2020. Herbs as thermoregulatory agents in poultry: an overview. Sci Total Environ. 703:134399.
- Abd El-Hack ME, Alagawany M, Shaheen H, Samak D, Othman SI, Allam AA, Taha AE, Khafaga AF, Arif M, Osman A, et al. 2020. Ginger and its derivatives as promising alternatives to antibiotics in poultry feed. Animals, 10(3), p.452. Animals 10(3):452.
- Abd El-Moneim AE, Sabic EM, Abu-Taleb AM. 2019. Influence of dietary supplementation of irradiated or non-irradiated olive pulp on biochemical profile, antioxidant status and immune response of Japanese quails. Biol Rhythm Res. 2: 1–16.
- Abdel-Moneim AE, Elbaz AM, Khidr RE, Badri FB. 2020. Effect of in ovo inoculation of Bifidobacterium spp. on growth performance, thyroid activity, ileum histomorphometry and microbial enumeration of broilers. Probiotics Antimicro Prot. 12(3):873–882.
- Abdel-Moneim AME, Sabic E, Abu-Taleb A, Ibrahim N. 2020. Growth performance, hemato-biochemical indices, thyroid activity, antioxidant status, and immune response of growing Japanese quail fed diet with full-fat canola seeds. Trop Anim Health Product. 52:1853–1862.
- Abdel-Moneim A-ME, Shehata AM, Khidr RE, Paswan VK, Ibrahim NS, El-Ghoul AA, Aldhumri SA, Gabr SA, Mesalam NM, Elbaz AM, et al. 2021. Nutritional manipulation to combat heat stress in poultry–A comprehensive review. J Therm Biol. 98:102915.
- Abdel-Moneim A-ME, Shehata AM, Mohamed NG, Elbaz AM, Ibrahim N. 2021. Synergistic effect of Spirulina platensis and selenium nanoparticles on growth performance, serum metabolites, immune responses, and antioxidant capacity of heat-stressed broiler chickens. Biol Trace Elem Res. 1–12. https://doi.org/https://doi.org/10.1007/s12011-021-02662-w.
- Abdelnour SA, El-Saadony MT, Saghir SAM, Abd El-Hack ME, Al-Shargi OYA, Al-Gabri N, Salama A. 2020. Mitigating negative impacts of heat stress in growing rabbits via dietary prodigiosin supplementation. Livest Sci. 240:104220.
- Abdelnour SA, Swelum AA, Salama A, Al-Ghadi MQ, Qattan SYA, Abd El-Hack ME, Khafaga AF, Alhimaidi AR, Almutairi BO, Ammari AA. 2020. The beneficial impacts of dietary phycocyanin supplementation on growing rabbits under high ambient temperature. Ital J Anim Sci. 19(1):1046–1056.
- Abdel-Shafi S, Al-Mohammadi A-R, Sitohy M, Mosa B, Ismaiel A, Enan G, Osman A. 2019. Antimicrobial activity and chemical constitution of the crude, phenolic-rich extracts of Hibiscus sabdariffa, Brassica oleracea and Beta vulgaris. Molecules. 24(23):4280.
- Abo Ghanima MM, Abd El-Hack ME, Othman SI, Taha AE, Allam AA, Abdel-Moneim A-ME. 2020. Impact of different rearing systems on growth, carcass traits, oxidative stress biomarkers and humoral immunity of broilers exposed to heat stress. Poultr Sci. 99(6):3070–3078.
- Abo Ghanima MM, Bin-Jumah M, Abdel-Moneim A-ME, Khafaga AF, Abd El-Hack ME, Allam AA, El-Kasrawy NI. 2020. Impacts of strain variation on response to heat stress and boldo extract supplementation to broiler chickens. Animals. 10(1):24.
- Abo Ghanima MM, Elsadek MF, Taha AE, Abd El-Hack ME, Mohamed E, Alagawany M, Ahmed BM, Elshafie MM, El-Sabrout K. 2020. Effect of housing system and rosemary and cinnamon essential oils on layers performance, egg quality, haematological traits, blood chemistry, immunity, and antioxidant. Animals. 10(2):245.
- Adeyemi KD, Sazili AQ. 2014. Efficacy of carcass electrical stimulation in meat quality enhancement: a review. Asian-Australas J Anim Sci. 27:447–456.
- Alagawany M, Elnesr SS, Farag MR, Abd El-Hack ME, Khafaga AF, Taha AE, Tiwari R, Yatoo MI, Bhatt P, Marappan G, et al. 2019. Use of licorice (Glycyrrhiza glabra) herb as a feed additive in poultry: current knowledge and prospects. Animals 9(8):536.
- Allen L, Dockrell DH, Pattery T, Lee DG, Cornelis P, Hellewell PG, Whyte MK. 2005. Pyocyanin production by Pseudomonas aeruginosa induces neutrophil apoptosis and impairs neutrophil-mediated host defenses in vivo. J Immunol. 174(6):3643–3649.
- Argyri AA, Zoumpopoulou G, Karatizas KA, Tsakalidou E, Nychas GJE, Paganou EZ, Tassou CC. 2013. Selection of potential probiotic lactic acid bacteria from fermented olives by in vitro tests. Food Microbiol. 33:282–291.
- Arif M, Hayat Z, Abd El-Hack ME, Saeed M, Imran HM, Alowaimer AN, Saadeldin IM, Taha AE, Swelum AA. 2019. Impacts of supplementing broiler diets with a powder mixture of black cumin, Moringa and chicory seeds. SA J Sci. 49(3):564–572.
- Ashour EA, El-Hack MEA, Shafi ME, Alghamdi WY, Taha AE, Swelum AA, Tufarelli V, Mulla ZS, El-Ghareeb WR, El-Saadony MT. 2020. Impacts of green coffee powder supplementation on growth performance, carcass characteristics, blood indices, meat quality and gut microbial load in broilers. Agriculture. 10(10):457.
- Ashour EA, Bin-Jumah M, Abou Sayed-Ahmed ET, Osman AO, Taha AE, Momenah MA, Allam AA, Swelum AA, Abd El-Hack ME. 2020. Effects of dried okra fruit (Abelmoschus esculentus L.) powder on growth, carcass characteristics, blood indices, and meat quality of stored broiler meat. Poultr Sci. 99:3060–3069.
- Aunchaleeb N, Sukanga A, Chanokporn P, Paweena P, Saksit C. 2009. Synthesis, isolation of phenazine derivatives and their antimicrobial activity. Walailak J Sci and Tech. 6(1):79–91.
- Balamatsia CC, Patsias A, Kontominas MG, Savvaidis I. 2007. Possible role of volatile amines as quality-indicating metabolites in modified atmosphere-packaged chicken fillets: correlation with microbiological and sensory attributes. Food Chem. 104(4):1622–1628.
- Barakat KM, Mattar MZ, Sabae SZ, Darwesh OM, Hassan SH. 2015. Production and characterization of bioactive pyocyanin pigment by marine Pseudomonas aeruginosa OSh1. Res J Pharm Biol Chem Sci. 6:933–943.
- Batiha GE, Magdy Beshbishy A, G Wasef L, Elewa YH, A Al-Sagan A, Abd El-Hack ME, Mohamed E, Taha AE, M Abd-Elhakim Y, Prasad Devkota H. 2020. Chemical constituents and pharmacological activities of garlic (Allium sativum L.): a review. Nutrients. 12(3):872.
- Bech-Larsen T, Scholderer J. 2007. Functional foods in Europe: consumer research, market experiences and regulatory aspects. Trends Food Sci Technol. 18(4):231–234.
- Brenner J, Kreig R, Stanley T. 2005. Bergey’s manual of systematic bacteriology. The probacteria, part A. Introductory essay. New York (NY): Springer; p. 27.
- Brisbane PG, Janik LJ, Tate ME, Warren RF. 1987. Revised structure for the phenazine antibiotic from Pseudomonas fluorescens 2-79 (NRRL B-15132). Antimicrob Agents Chemother. 31(12):1967–1971.
- Çabuk M, Bozkurt M, Alçiçek A, Akbaþ Y, Küçükyýlmaz K. 2006. Effect of a herbal essential oil mixture on growth and internal organ weight of broilers from young and old breeder flocks. SA J Sci. 36(2):135–141.
- Cunningham SA, Patel R. 2013. Importance of using Bruker’s security-relevant library for biotyper identification of Burkholderia pseudomallei, Brucella Species, and Francisella tularensis. J Clin Microbiol. 51:1639–1640. PMID: 23447635.
- Dahah H, Djibaoui R, Saïd N. 2016. Antimicrobial, antioxidant and hemolytic effects of Pyocyanin produced by Pseudomonas aeruginosa isolated from saline soil of Mina river. Algeria Int J Biosci. 9(5):134–143.
- Edwards EA, Hilderbrand RL. 1976. Method for identifying Salmonella and Shigella directly from the primary isolation plate by coagglutination of protein A-containing staphylococci sensitized with specific antibody. J Clin Microbiol. 3(3):339–343.
- Elbaz AM, Ibrahim NS, Shehata AM, Mohamed NG, Abdel-Moneim AME. 2021. Impact of multi-strain probiotic, citric acid, garlic powder or their combinations on performance, ileal histomorphometry, microbial enumeration and humoral immunity of broiler chickens. Trop Anim Health Prod. 53(1):10.
- El-Saadony MT, Elsadek MF, Mohamed AS, Taha AE, Ahmed BM, Saad AM. 2020. Effects of chemical and natural additives on cucumber juice’s quality, shelf life, and safety. Foods. 9(5):639.
- El-Shouny WA, Al-Baidani ARH, Hamza WT. 2011. Antimicrobial activity of pyocyanin produced by Pseudomonas aeruginosa isolated from surgical wound-infections. Int J Pharm Med Sci. 1(1):01–07.
- Essar D, Eberly L, Hadero A, Crawfort I. 1990. Identification and characterization of genes for second anthranilate synthase in Pseudomonas aeroginosa: interchaneability of the two anthranilate synthases and evolutionary implications. J Bacteriol. 172(2):884–900.
- Fernandez-Lopez J, Zhi N, Aleson-Carbonell L, Pérez-Alvarez JA, Kuri V. 2005. Antioxidant and antibacterial activities of natural extracts: application in beef meatballs. Meat Sci. 69(3):371–380.
- Francis FJ, Clydesdale FM. 1975. Food colorimetry: theory and applications. Westport (CT): AVI Publishing Co. Inc.
- Gado AR, Ellakany HF, Elbestawy AR, Abd El-Hack ME, Khafaga AF, Taha AE, Arif M, Mahgoub SA. 2019. Herbal medicine additives as powerful agents to control and prevent avian influenza virus in poultry–a review. Ann Anim Sci. 19(4):905–935.
- Giddens S, Houliston G, Mahanty H. 2003. The influence of antibiotic production and pre-emptive colonization on the population dynamics of Pantoea agglomerans (Erwinia herbicola) Eh1087 and Erwinia amylovora in planta. Environ Microbiol. 5(10):1016–1021.
- Gok V, Obuz E, Akkaya L. 2008. Effect of packaging method and storage time on the chemical, microbiological, and sensory properties of Turkish pastirma – A dry cured beef product. Meat Sci. 80(2):335–344.
- Goulas AE, Kontominas MG. 2005. Effect of salting and smoking-method on the keeping quality of chub mackerel (Scomber japonicus): biochemical and sensory attributes. Food Chem. 93(3):511–520.
- Harrigen WF, Mccance-Margart E. 1976. Laboratory methods in food and dairy microbiology. London: Academic Press; p. 1–115.
- Hassani HH, Hasan HM, Al-Saadi A, Ali AM, Muhammad MH. 2012. A comparative study on cytotoxicity and apoptotic activity of pyocyanin produced by wild type and mutant strains of Pseudomonas aeruginosa. Eur J Exp Biol. 2(5):1389–1394.
- Ibrahim N, Sabic E, Abu-Taleb A, Abdel-Moneim A. 2020. Effect of dietary supplementation of full-fat canola seeds on productive performance, blood metabolites and antioxidant status of laying Japanese quails. Braz J Poult Sci. 22(1):1–10.
- Islam MT, Ali ES, Uddin SJ, Shaw S, Islam MA, Ahmed MI, Shill MC, Karmakar UK, Yarla NS, Khan IN. 2018. Phytol: a review of biomedical activities. Food Chem Toxicol. 121:82–94.
- Kaleli I, Cevahir N, Demir M, Yildrim U, Sahin R. 2007. Anticandidal activity of Pseudomonas aeruginosa strains isolated from clinical specimens. Mycoses. 50(1):74–78.
- Kerr JR. 1994. Suppression of fungal growth exhibited by Pseudomonas aeruginosa. J Clin Microbiol. 32(2):525–527.
- Kerr JR, Taylor GW, Rutman A, Hoiby N, Cole PJ, Wilson R. 1999. Pseudomonas aeruginosa pyocyanin and 1-hydroxyphenazine inhibit fungal growth. J Clin Pathol. 52(5):385–387.
- Khafaga AF, Abd El-Hack ME, Taha AE, Elnesr SS, Alagawany M. 2019. The potential modulatory role of herbal additives against Cd toxicity in human, animal, and poultry: a review. Environ Sci Pollut Res. 26(5):4588–4604.
- Kim K. 2000. Phenazine-1-carboxylic acid resistance in phenazine-1- carboxilic acid producing Bacillus sp. B-6. J Biochem Mol Biol. 33:332–336.
- Kurtzman C, Fell JW. 1984. The yeasts, a taxonomic study3. Amsterdam, The Netherlands: Elsevier Science Publishers.
- Laursen J, Nielsen J. 2004. Phenazine natural products: Biosynthesis, synthetic analogue and biological activity. Chem Rev. 104(3):1663–1685.
- Laxmi MS, Bhat G. 2016. Characterization of pyocyanin with radical scavenging and antibiofilm properties isolated from Pseudomonas aeruginosa Strain BTRY 1. 3 Biotech. 6(1):27.
- Mahon C, Lehman D, Manuselis G. 2007. Textbook of diagnostic microbiology. 3rd ed. Amsterdam, The Netherlands: Elsevier; p. 508.
- Makarand R Rane, Prashant D Sarode, Bhushan L Chaudhari and Sudhir B Chincholkar (2007). Detection, isolation and identification of phenazine -1- carboxylic acid produced by biocontrol strain of Pseudomonas aeruginosa. Journal of Scientific & Industrial Research 66, 627–631.
- Marrez DA, Haitham SM. 2020. Biological activity and applications of pyocyanin produced by Pseudomonas aeruginosa. Open Acc J Bio Sci. 1: 140–144.
- Marzoni M, Chiarini R, Castillo A, Romboli I, De Marco M, Schiavone A. 2014. Effects of dietary natural antioxidant supplementation on broiler chicken and Muscovy duck meat quality. Anim Sci Papers Rep. 32:359–368.
- Moghadam ZA, Hosseini H, Hadian Z, Asgari B, Mirmoghtadaie L, Mohammadi A, Shamloo E, Javadi NHS. 2019. Evaluation of the antifungal activity of cinnamon, clove, thymes, zataria multiflora, cumin and caraway essential oils against Ochratoxigenic Aspergillus ochraceus. J Pharm Res Int. 26:1–16.
- Murray PR, Baron EJ, Pfaller MA, Tenover FC, Yolk RH. 1995. Manual of Clinical Microbiology, 6th ed. Mosby Year Book, London.
- Nasir A, Aasima R, Faneshwar K, Vijay S, Vivek S. 2017. Determinants of broiler chicken meat quality and factors affecting them: a review. J Food Sci Technol. 54(10):2997–3009.
- Nam TG. 2011. Lipid peroxidation and its toxicological implications. Toxicol Res. 27(1):1–6.
- Pal R, Revathi R. 1998. Susceptibility of yeasts to Pseudomonas aeruginosa. Indian J Med Microbiol. 16(2):72–74.
- Pearson AM, Gillette TA. 1996. Processed meats. 3rd ed. New York Albany, Bonn, Boston, London.
- Rada B, Jendrysik MA, Pang L, Hayes CP, Yoo D. 2013. Pyocyanin enhanced neutrophil extracellular trap formation requires the NADPH oxidase. PLoS One. 8(1):e54205.
- Rahman PK, Pasirayi G, Auger V, Ali Z. 2009. Development of simple and low-cost micro-bioreactor for high throughput bioprocessing. Biotechnol Lett. 31(2):209–214.
- Raji El Feghali P, Nawas T. 2018. Extraction and purification of pyocyanin: a simpler and more reliable method. MOJ Toxicol. 4(6):417–422.
- Ran HM, Hassett DJ, Lau GW. 2003. Human targets of Pseudomonas aeruginosa pyocyanin. Proc Natl Acad Sci USA. 100(24):14315–14320.
- Reda FM, El-Saadony MT, Elnesr SS, Alagawany M, Tufarelli V. 2020. Effect of dietary supplementation of biological curcumin nanoparticles on growth and carcass traits, antioxidant status, immunity and caecal microbiota of Japanese quails. Animals 10(5):754.
- Reda FM, El-Saadony MT, El-Rayes TK, Attia AI, El-Sayed SAA, Ahmed SYA, Madkour M, Alagawany M. 2021. Use of biological nano zinc as a feed additive in quail nutrition: biosynthesis, antimicrobial activity and its effect on growth, feed utilisation, blood metabolites and intestinal microbiota. Ital J Anim Sci. 20(1):324–335.
- Szabo RA, Todd CD, Jean A. 1986. Method to isolate Escherichia coll 0157.H7 from food. J Food Protect. 49(10):768–772.
- Saad AM, Mohamed AS, Ramadan MF. 2020. Storage and heat processing affect flavors of cucumber juice enriched with plant extracts. Int J Veg Sci. 1: 1–11.
- Saha S, Thavasi R, Jayalakshmi S. 2008. Phenazine pigments from Pseudomonas aeruginosa and their application as antibacterial agent and food colourants. Res J Microbiol. 3(3):122–128.
- Sheiha AM, Abdelnour SA, Abd El-Hack ME, Khafaga AF, Metwally KA, Ajarem JS, Maodaa SN, Allam AA, El-Saadony MT. 2020. Effects of dietary biological or chemical-synthesized nano-selenium supplementation on growing rabbits exposed to thermal stress. Animals 10(3):430.
- Shewita RS, Taha AE. 2019. Influence of dietary supplementation of ginger powder at different levels on growth performance, haematological profiles, slaughter traits and gut morphometry of broiler chickens. SA J Sci. 48(6):997–1008.
- Sitohy M, Osman A, Gharib A, Chobert JM, Haertlé T. 2013. Preliminary assessment of potential toxicity of methylated soybean protein and methylated β-lactoglobulin in male Wistar rats. Food Chem Toxicol. 59:618–625.
- SP SS 2008. Statistical Package for Social Sciences Program. Version 17 for Windows, SP SS Inc., Chicago.
- Stephen J. 1981. Anthrax toxin. Pharmacol Ther. 12(3):501–513.
- Sudhakar T, Karpagam S, Shiyama S. 2013. Antifungal efficacy of pyocyanin produced from bioindicators of nosocomial hazards. Int J ChemTech Res. 5:1101–1106.
- Suvarna, Kim S., Christopher Layton, and John D. Bancroft, eds. Bancroft's theory and practice of histological techniques E-Book. Elsevier Health Sciences, 2018.
- Uğur A, Ceylan Ö, Aslım B. 2012. Characterization of Pseudomonas spp. from seawater of the southwest coast of Turkey. J Biol Environ Sci. 6(16):25–33.
- Wang J, Fang X, Ge L, Cao F, Zhao L, Wang Z, Xiao W. 2018. Antitumor, antioxidant and anti-inflammatory activities of kaempferol and its corresponding glycosides and the enzymatic preparation of kaempferol. PLoS One. 13:e0197563.
- Xiong YL. 2000. Protein oxidation and implications for muscle food quality. In: Decker E, Faustman C, Lopez-Bote CJ, editors. Antioxidants in muscle foods: nutritional strategies to improve quality. New York (NY): John Wiley and Sons; p. 85–111.
- Yi S, Li J, Zhu J, Lin Y, Fu L, Chen W, Li X. 2011. Effect of tea polyphenols on microbiological and biochemical quality of collichthys fish ball. J Sci Food Agric. 91(9):1591–1597.
- Zhao S, Li N, Li Z, He H, Zhao Y, Zhu M, Wang Z, Kang Z, Ma H. 2019. Shelf life of fresh chilled pork as affected by antimicrobial intervention with nisin, tea polyphenols, chitosan, and their combination. Int J Food Prop. 22(1):1047–1063.