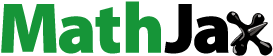
Abstract
This study was conducted to determine the effects of grower diet (d11-24) digestible sulphur amino acids (SAAs) levels on performance traits, blood metabolites, and immune responses to Newcastle disease (ND) and avian influenza (AI) vaccination in the broiler chickens. Additionally, digestible SAAs requirements were determined based on nutrient dose-response data. A total of 360, 11‐day‐old male Ross-308 broiler chicks were randomly allocated to six dietary treatments, graded levels of supplementary DL-methionine (+0.7 g/kg), but cystine (0.29%) was equal across groups, lead to levels of 0.59, 0.66, 0.73, 0.8, 0.87, and 0.94% dietary digestible SAAs, with six replicates and ten birds each. All birds were vaccinated with inactivated H9N2/ND and live-ND on days 5 and 11, respectively. Increasing dietary digestible SAAs level improved live body weight (LBW), weight gain (WG), feed efficiency (FE) with linear trends, and improved carcass yield, relative breast weight (RBW), and immune responses with quadratic trends. On day 24, a quadratic increase in blood serum albumin concentration was concomitant with decreasing triglyceride, whole cholesterol, low‐density-lipoprotein cholesterol, creatinine, and alkaline phosphatase concentration with increasing dietary digestible SAAs levels. Based on broken‐line regression models, the LBW, WG, FE, RBW, and immunity against ND and AI vaccination were optimised when dietary digestible SAAs levels were 0.73, 0.69, 0.78, 0.86, 0.73, and 0.72%, respectively. It is concluded, the digestible SAAs requirements of broilers vary according to what production parameter is taken considered for optimisation. The best grower diet digestible SAAs concentration achieved 0.86% for support performance, breast yield, and immune function.
The present data highlight that a better breast meat yield could be obtained by supplementing DL-Met, which raised dietary digestible sulphur amino acids level to 0.86%.
Highlights
Introduction
Body weights of commercial meat-type chickens will increase 55–60 fold by six weeks after hatching. A large part of weight gain is the tissue with protein content. Thus, adequate amino acid nutrition is vital to the successful feeding program for meat-type chicken (Zarghi et al. Citation2020b). Additionally, balanced dietary amino acid concentration in diet plays a primary role in improving growth performance, bird welfare, and diminish environmental pollution by poultry manure (Zarghi et al. Citation2020a).
Methionine (Met) is an essential amino acid that plays many roles in living systems and has a significant role in various biological processes such as protein anabolism, production of other S-containing amino acids, methyl donor, a precursor of intermediates in metabolic pathways, and synthesis of polyamines (Faridi et al. Citation2016; Ghavi et al. Citation2020). Broiler diets formulated on the corn-soybean meal basis are deficient in Met + cystine (Cys) which are referred to as S-containing amino acids (Goulart et al. Citation2011; Faridi et al. Citation2016). Various researchers have been reported a positive effect on broiler’s liveability, feed efficiency, growth performance (Rehman et al. Citation2019; Ghavi et al. Citation2020), and immunity via Met supplementation of corn-soybean meal diets (Swain and Johri Citation2000). A sufficient metabolic supply of sulphur amino acids (SAAs) from diet and tissue protein breakdown is necessary for the synthesis of proteins and peptides implicated in the normal functioning of the immune system (Grimble Citation2006).
The estimation of SAAs requirement is a vital key to providing appropriate nutrition in poultry (Akbari Moghaddam Kakhki et al. Citation2016). There are many previous studies conducted to determine the nutrients requirement of broilers. Due to the improvement in the growth rate that happened in recent years, it is necessary to update these requirements (Faridi et al. Citation2016). It has been suggested that Ross-308 broilers, for target live weight 1.7–2.4 kg, digestible Met and digestible SAAs (Met + Cys) requirements as: 0.51, 0.47, and 45%; and 0.95, 0.87, and 0.83%, during the starter (d1–10), grower (d11–24) and finisher (d25-market) periods, respectively (Aviagen Citation2019). Ranging 0.3–0.9% of Met concentration in the grower diet of broiler chickens has been reported (Jankowski et al. Citation2014; Rehman et al. Citation2019). Broiler chickens need 0.38% Met in the grower diets for the optimised growth performance, but a higher rate of Met is necessary for raise breast yield and immunity (Kalinowski et al. Citation2003; Jankowski et al. Citation2014; Rehman et al. Citation2019). Kalinowski et al. (Citation2003) studied the effect of 0.32, 0.38, 0.44, and 0.50% DL-Met level with a constant level of L-Cys (0.40%) on slow and fast-growing broilers from 3 to 6 weeks of age, fed a steam pelleted and crumbled corn-soybean meal diet, and observed that weight gain (WG) was not affected but feed efficiency (FE) improved with the highest level of methionine.
Given this background, the present study was undertaken to evaluate the effects of graded levels of dietary digestible SAAs on performance, blood metabolites, liver functional enzymes, and immune response to Newcastle disease (ND) and avian influenza (AI) vaccination in broilers. Additionally, we hypothesised that it is possible to find an optimal inclusion level of digestible SAAs for broilers during the grower period (11-24 days of age) that allows them to reach maximum growth performance, carcass yield, and immune.
Materials and methods
The current study was done at the Poultry Research Station and the Animal Nutrition Laboratory, Ferdowsi University of Mashhad, Mashhad, Iran (Approval Number: 356/493/2019).
Birds, housing, experimental design, and diets
A total of 432, one‐day‐old male Ross-308 broiler chicks were randomly distributed to 36 experimental unit (floor pens, 1 × 1 m2, equipped with hanging pan feeder and nipple waterer and covered with wood shavings) with 12 birds each. Chicks fed a typical starter diet formulated to meet Ross-308 recommendations (Aviagen Citation2019) for the first ten days of age (starter period). On day 11, with removing heavier and lighter birds, ten birds with the highest uniformity were fixed in each experimental unit and assigned to six dietary treatments, six replicates/treatment. The experimental unit bird’s mean weight was 251 ± 5.85 g, and the difference between treatments was not significant.
A completely randomised design (CRD) experiment was run to comprise of six graded levels of supplementary DL-Met (+0.7 g/kg), while diets Cys (0.29%) was equal, correspond to six levels of digestible SAAs included 0.59, 0.66, 0.73, 0.80, 0.87, and 0.94%. Before to the start experiment, sample of main ingredients that used in feed formulation, corn (data on as-fed basis: DM, 91.83%; GE, 4121 kcal/kg; CP, 7.85%; Met, 0.17%; Cys, 0.18%; EE, 4.5%; CF, 2.3; and ash, 1.2%) and soybean meal (data on as-fed basis: DM, 92.52%; GE, 4414 kcal/kg; CP, 45.22%; Met, 0.628%; Cys, 0.68%; EE, 3.4%; CF, 5.8; and ash, 6.5%) were analysed by the methods described (AOAC Citation2002), and corn (data on as-fed basis: ME, 3498 kcal/kg; digestible Met, 0.16%; digestible SAAs, 0.32; digestible Lys, 0.22%; and digestible Thr, 0.24%) and soybean meal (data on as-fed basis: ME, 2405 kcal/kg; digestible Met, 0.57, digestible SAAs, 1.09; digestible Lys, 2.48; and digestible Thr, 1.46%) were measured by NIR through Evonik (Evonik Nutrition& Care GmbH, agent in Tehran, Iran). The values were used in a least‐cost equation for the formulation basal diet by user-friendly feed formulation done again (UFFDA, 1992) software, University of Georgia, Athens, GA, United States. A basal grower (d11-24) diet was formulated to meet or exceed Ross recommendations except for digestible SAAs (Table ). The experimental diets were provided in a way that a batch of basal diet was made and then divided into six equal portions, and a DL-Met Amino Acid feed‐grade supplement (Met AMINO, >99%, Evonik Degussa GmbH, Hanau-Wolfgang, Germany; ME = 5020 kcal/kg; CP = 58.1%; dig Met = 99%;) was added at the rate of 0.0, 0.7, 1.4, 2.1, 2.8 and 3.5 g/kg to the top of each portion at the expense of filler (corn starch) and mixed to provide six dietary treatments. The supplemental DL-Met and analysed nutrient composition in experimental diets are shown in Table . Birds fed for 14 days (during 11-24 days of age). All birds had free access to mash feed and water throughout the experiment period. Birds and housing facilities were inspected three times daily.
Table 1. Ingredients and nutrients composition of the basal dieta.
Table 2. DL-methionine supplementation and determined nutrients composition in experimental dietsa.
Vaccine inoculation protocol
On day 5, all birds were inoculated with a commercial inactivated ND and AI vaccine (H9N2/ND 0.3 ml/bird, Razi Vaccine & Serum Research Institute, Iran) by subcutaneous route in the back of the neck. Additionally, on day 11, all birds were inoculated by oral route with a commercial live-ND vaccine (Avinew® NeO, Boehringer Ingelheim, Lyon, France). The content of the 1000 dose of ND vaccine vial was reconstituted in 1000 ml distilled water, and each bird was inoculated with 1.1 ml ND virus into the lumen of the crop by oral gavage.
Growth performance trait
All pen birds were weighed at the initial and end of the experimental period (d11 and d24), and feed consumption was measured by weighing the offered and residual feeds for each experimental unite. The growth performance indices as mean live body weight (LBW), daily WG, and daily feed intake (FI) were calculated. The FE, adjusted for mortality and calculated as; WG/FI (Zarghi et al. Citation2020b).
Blood sampling
On day 24 of age, two birds from each pen (12/treatment) were randomly selected, and blood samples were collected from the brachial vein of each bird into non‐heparinized tubes. After allowing for the completion of clotting, blood samples were centrifuged at 1,900 g for 5 min at 4 °C to extract serum. Subsequently, serum samples were divided into two subsamples and frozen at −20 °C for later analysis (Zarghi et al. Citation2020a).
Blood metabolites
Serum triglyceride (TG), whole cholesterol, high‐density lipoprotein cholesterol (HDL-C), low‐density lipoprotein cholesterol (LDL-C), total fat (TF), uric acid (UA), creatinine, total protein (TP), albumin, alanine aminotransferase (ALT), aspartate aminotransferase (AST), and alkaline phosphatase (ALP) concentration were measured by kits from Pars Azmoon Company, Iran using a multi‐test automatic random‐access system auto‐analyser (Cobas Bio, Roche). Before testing, samples were removed from the freezer, thawed, and warmed to room temperature.
Immune responses
Antibody titre against ND virus was performed using commercially available HI kits (IDEXX, Labs Inc., Westbrook, Maine, USA), briefly twofold dilutions of the sera to be tested are made in 96-well plates, then a known titre of the virus is added, and the plate is incubated for 30 minutes at room temperature (Allahdo et al. Citation2018). Also, antibody titre against AI virus was performed using commercially available ELISA kits (IDEXX, Labs Inc., Westbrook, Maine, USA) according to the manufacturer’s instructions. ELISA absorbency was measured at 650 nm using an ELISA reader (Bio-Tek Instruments Inc. ELX 800; Winooski, Vermont) by standard procedures.
Carcass and visceral organs relative weight
On day 24 of age, two birds from each pen (12/treatment) after 4 hr of feed withdrawal but had free access to drinking water were chosen and decapitated. The carcasses were eviscerated manually and were obtained by removing the head, feathers, feet, and visceral organs. Whole carcasses were weighed to determine the carcass weight and then were cut according to a standard procedure (CitationTabatabaei Yazdi et al. 2017); breast (without skin), legs (thigh plus drumstick), and others (neck, wings plus back). Visceral organs, total carcass, and carcass portions were weighed by a digital weighing scale (0.001 g, model GF 400, A&D Weighing).
Statistical analysis
All data were analysed for normality using SAS 9.1 software through the Univariate procedure. Then, data were analysed using the general linear model of SAS with dietary treatment as independent variables. Linear and quadratic models were analysed to describe the relationships between digestible SAAs level and variables. In order to estimate the different optimised responses, used of linear broken-line and quadratic broken-line (QBL) models (Robbins et al. Citation2006; Pesti et al. Citation2009). The iterative procedure makes repeated estimates for coefficients and minimises residual error until the best-fit lines are achieved: one line with a slope of zero and the other with a marked slope. The two lines are fitted to the values using the following equations:
Where: Y = dependent variable, L = theoretical maximum, R = requirement, X = independent variable, I = 1 (if X < R) or I = 0 (if X > R), and U = rate constant.
To assist in choosing an appropriate model, coefficient of determination (R2), adjusted coefficient of determination (adj. R2), root means square error (RMSE), and Akaike’s information criterion (AIC) values were calculated using the following formulas.
Where: yt = observed and predicted values, ŷt = predicted values, N = number of observations. P = k + 1; k is the number of parameters.
The exploration of the models according to the fit (quality of prediction) was done using the adj. R2, RMSE, and AIC criterion, a smaller numerical value of RMSE, AIC, and higher value of adj. R2 indicates a better fit when comparing models.
Results
Growth performance
Responses to dietary digestible SAAs level were significant (linear effect, p<.05) for LBW at 24 days of age and WG and FE during the grower period (11–24 days of age). Live body weight, WG, and FE improved as dietary digestible SAAs level increased (Table ). Birds fed diet contain 0.73 and 0.80% digestible SAAs, performed the highest LBW, FE, and WG, which were 21.75, 16.11, and 22.08%, higher than those fed non-Met supplemented diet (digestible SAAs = 0.59%), respectively. Feed intake was not affected by digestible SAAs level (p>.05). The relative carcass (p<.05) and breast weight improved (p<.01) as increased dietary digestible SAAs level by a quadratic trend. However, supplemental DL-Met did not affect (p>.05) on legs (thigh & drumstick), frame (carcass without breast and legs), liver, heart, gastrointestinal tract, and dressing (cover) relative weight (Table ).
Table 3. Effect of dietary digestible sulphur amino acids (DSAAs) level on growth performance, and daily DSAAs intake in male Ross-308 broilers during grower period (d11-24 of age)a.
Table 4. Effect of dietary digestible sulphur amino acids (DSAAs) level on carcass and cut relative weight (g/100 g of live body weight) in male Ross-308 broilers on day 24a.
Blood biochemistry and immune responses
The results for blood metabolites and immunity responses are shown in Table . Responses to dietary digestible SAAs level were significant (quadratic effect, p<.05) for blood metabolites and liver function enzymes, also ND and AI vaccination response were significant with quadratic trends (p<.01). The highest blood serum alkaline phosphatase (ALP) concentration was observed in the birds fed the non-supplemented diet (digestible SAAs = 0.59%). By increasing dietary digestible SAAs level up to 0.73%, ALP concentration decreased, but over that, increased. The blood serum ALT and AST concentration did not affect by dietary digestible SAAs level (p>.05). Regression analyses showed significant and quadratic effects (p<.01) due to dietary digestible SAAs concentrations for TG, cholesterol, LDL-C, and creatinine. Immune response to ND and AI vaccination was affected by dietary digestible SAAs level significant and quadratic (p<.01). The partial correlation coefficients and probability values between growth performance traits with blood serum metabolites and liver functional enzymes on day 24 have shown in Table . Live body weight had a significant and negative correlation with blood serum TG (-0.41), LDL-C (-0.34), and liver enzymes; ALT (-0.5), AST (-0.42), and ALP (-0.51) concentration. Breast relative weight had a negative correlation with liver function enzymes, ALT (-0.43) and ALP (-0.32) concentration, and a positive correlation with blood serum total protein (0.32) and albumin (0.34) concentration. Besides, FE showed a significant and negative correlation with TG (-0.3), cholesterol (-0.35), LDL-C (-0.4), UA (-0.39), and ALP (-0.32).
Table 5. Effect of dietary digestible sulphur amino acids (DSAAs) level on blood metabolites and immune responses (antibody titre) to Newcastle disease, and avian influenza vaccination in male Ross-308 broilers on day 24a.
Table 6. Partial correlation coefficients (above) and probability values (below) between growth performance traits with blood serum constituent of male Ross-308 broilers on day 24.
Estimated digestible sulphur amino acids requirements
A critical goal of this study was to estimate the digestible SAAs requirement of Ross-308 broilers during the grower (d11–24) period. The optimisation model was solved using the NLIN program SAS 9.1 procedure. Fitted broken-line models for the growth performance traits, relative breast weight, and immunity as a function of dietary digestible SAAs concentration are shown in Table . However, the FI responses did not fit in the linear and quadratic broken-line models to optimised dietary digestible SAAs level for FI. Based on the highest adj. R2 and the lowest RMSE and AIC, linear broken-line fit models were selected to estimate digestible SAAs requirements for live body weight (Figure ) and weight gain (Figure ). Similarly, a quadratic broken-line fit model was achieved to estimate the digestible SAAs requirement for feed efficiency (Figure ), relative breast weight (Figure ), and immune responses to ND (Figure ) and AI (Figure ) vaccination. The present data suggest that Ross-308 broilers digestible SAAs requirement during the grower period to optimise LBW, WG, FE, RBW, and immune responses to ND and AI vaccination were 0.73, 0.69, 0.78, 0.86, 0.73, and 0.72%, respectively.
Figure 1. Live body weight at 24 d of age (Y, in g/b) as a function of dietary digestible sulphur amino acids level (X, in % of diet) fed from 11 to 24 d of age. Linear broken-line, Y = 900–764(0.73-X), p < .005, R2=0.29. The break point occurred at 0.73 ± 0.09.
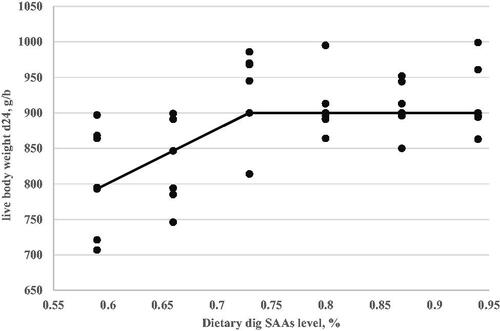
Figure 2. Daily weight gain (Y, in g/b) as a function of dietary digestible sulphur amino acids level (X, in % of diet) fed from 11 to 24 d of age. Linear broken-line, Y = 45.71–123.2(0.69-X), p < .001, R2=0.47. The break point occurred at 0.69 ± 0.03.
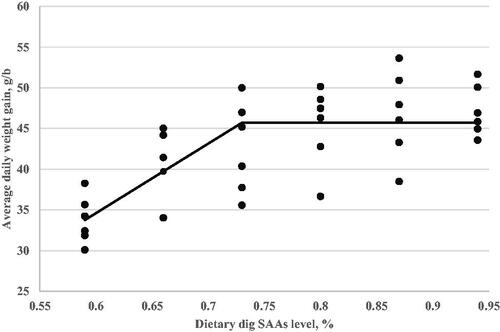
Figure 3. Feed efficiency (Y, g WG/100 g FI) as a function of dietary digestible sulphur amino acids level (X, in % of diet) fed from 11 to 24 d of age. Quadratic broken-line, Y = 62.30–236(0.78–X)2, p<.006, R2=0.33. The break point occurred at 0.78 ± 0.09.
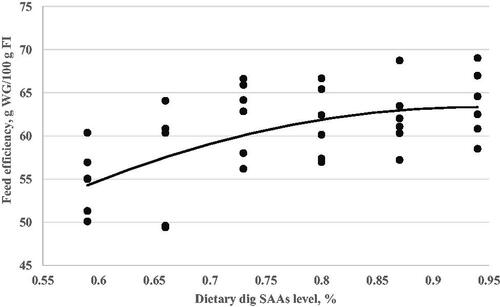
Figure 4. Breast relative weight at 24 day of age (Y, in % of live body weight) as a function of dietary digestible sulphur amino acids level (X, in % of diet) fed from 11 to 24 d of age. Quadratic broken-line, Y = 23.95-67.93(0.86-X)2, p < .001, R2=0.50. The break point occurred at 0.86 ± 0.09.
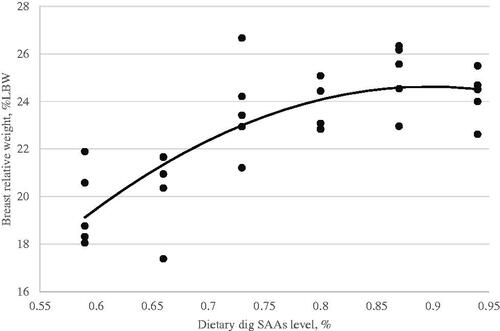
Figure 5. Serum antibody titre in response to Newcastle disease vaccination (Y, in log2) as a function of dietary digestible sulphur amino acids level (X, in % of diet) fed from 11 to 24 d of age. Quadratic broken-line, Y = 5.56–1.2(0.73-X)2, p < .003, R2=0.41. The break point occurred at 0.73 ± 0.07.
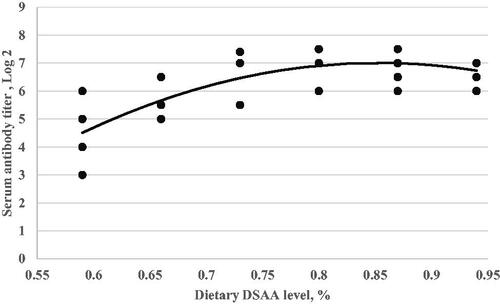
Figure 6. Serum antibody titre in response to avian influenza vaccination (Y, in log2) as a function of dietary digestible sulphur amino acids level (X, in % of diet) fed from 11 to 24 d of age. Quadratic broken-line, Y = 5.31–136(0.72-X)2, p < .001, R2=0.76. The break point occurred at 0.72 ± 0.03.
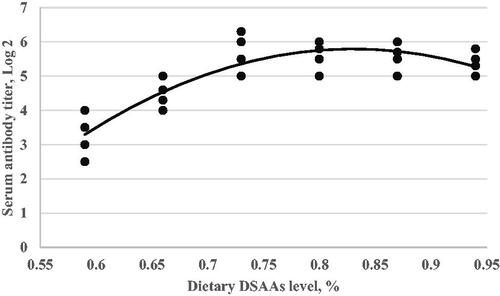
Table 7. Estimated digestible sulphur amino acids (DSAAs) requirement of male Ross-308 broilers during grower period (d11-24 of age) for optimisation of growth performance, and immune responses (antibody titre log2) to Newcastle disease and avian influenza vaccination by broken‐line regression fit models analysisa.
Discussion
Growth performance
In agreement with other published work (Ohta and Ishibashi Citation1995; Conde-Aguilera et al. Citation2013; Rehman et al. Citation2019; Ghavi et al. Citation2020; Ren et al. Citation2020; Zarghi et al. Citation2020b), in the current study increasing supplemental DL-Met improved growth performance (Table and Figures ). Methionine supplementation improves dietary amino acid balance and consequently promotes LBW and WG growth by increasing protein synthesis and decreasing fat synthesis, leading to enhanced feed efficiency. Increasing DL-methionine supplementation significantly improved daily weight gain and feed conversion efficiency in the broilers fed pelleted diet (Schutte et al. Citation1997). Feed intake was not influenced by increasing dietary levels of digestible SAAs. This finding agrees with the other researchers (Ohta and Ishibashi Citation1995; Rehman et al. Citation2019; Zarghi et al. Citation2020b). They have observed no significant difference in FI due to dietary supplementation with DL-Met. In contrast, Moran and Edwin (Citation1994) has shown that broilers increase the consumption of feed when the diet contains inadequate amounts of methionine. Goulart et al. (Citation2011) showed a linear decline effect of digestible SAAs levels on the feed intake of Cobb broilers during the 8–21 days of age period. However, during the 1–7, 22–35, and 36–42 days of age periods, there was no significant effect of dietary digestible SAAs levels on feed intake. Xie et al. (Citation2004) reported increasing dietary levels of DL-Met from 0.285 to 0.685% led to decrease feed intake and weight gain in early Peking ducklings because of higher plasma homocysteine concentration and discussed this might be related to differences in the source of methionine used. In the conversion of D-Met to L-Met, different methionine sources may perform differently (Rehman et al. Citation2019) .
Our results in this experiment showed increasing dietary digestible SAAs level up to 0.87% improved relative carcass and breast weight of broiler chickens (Table and Figure ), which confirms previous studies (Schutte et al. Citation1997; Yoo et al. Citation2017; Rehman et al. Citation2019), where levels of methionine had a significant influence on breast weight. Reported dietary Met level will affect growth performance and carcasses quality (Wu et al. Citation2012). The SAAs may be considered to be the principal S-containing AAs which are incorporated into protein structure (Ghavi et al. Citation2020). Besides protein synthesis, methionine is involved in other important aspects of metabolism in the animal body. For instance, methionine is required as a substrate in physiologic reactions as a methyl donor in transmethylation, and this process could be spared by additional choline or betaine (Ghavi et al. Citation2020). Also, Met is a substrate for the transsulfuration process, being converted to cysteine. Therefore, the cystine concentration in the basal diet was considered whenever the methionine requirement was determined. Although cystine cannot replace methionine, it can lower the methionine requirement (Scott et al. Citation2002). Therefore, with growth studies, it isn’t easy to separate the different requirements for methionine, especially if cystine and methyl donors are not carefully controlled (Chamruspollert et al. Citation2002).
Blood metabolites
The values for TG, whole cholesterol, LDL-C, and creatinine were quadratic and significantly affected by dietary digestible SAAs levels. Our results in this experiment showed a negative correlation between LBW with blood serum TG and LDL-C concentration. Additionally, the correlation between FE with blood serum TG, whole cholesterol, LDL-C, and UA concentration was negative. These results agree with other reported on investigated quantitative feed restriction on blood metabolites (Jahanpour et al. Citation2015). Among the amino acids, sulphur amino acids have the highest potential for adjustment to the fat metabolism. Indeed, the addition of Met has been correlated with the tendency to less lipogenesis (Ghavi et al. Citation2020). Methionine plays an essential role in lipid metabolism as a precursor for L-carnitine (Sigolo et al. Citation2019). L-carnitine has hypolipidemic effects, lead to reduce blood concentrations of whole cholesterol, TG, free fatty acids, and VLDL-C (Diaz et al. Citation2000).
Liver functional enzymes were affected by dietary digestible SAAs level. Whereas in the birds fed 0.59% digestible SAAs level diet, the blood serum AST and ALP concentration were the highest and linearly decreased by increasing dietary digestible SAAs concentration (Table ). Also, 24d LBW, RBW, and FE negatively correlated with blood serum AST and ALP concentration (Table ). Liver functional enzymes are commonly used for evaluating hepatocyte damage and liver inflammation. The liver, as the main organ in body metabolism, is sensitive to nutritional modifications. The serum ALT and AST concentration are usually considered essential indices for understanding liver health. It has been demonstrated that the liver enzymes involved in AAs catabolism (Muramatsu et al. Citation1971). It is cleared, the concentration of these two enzymes in serum will reduce in broiler chickens when the liver works healthy (Rezaeipour et al. Citation2016). Sulphur containing amino acids (cystine and methionine) may improve antioxidant properties by participating in the production of GSH (Afkhami et al. Citation2020). Reported stress increased liver enzyme activity, but supplementation of antioxidant nutrients could alleviate the adverse effects of stress (Kanani et al. Citation2016). Rezaeipour et al. (Citation2016) reported diet formulation on the digestible amino acid basis led to significantly reduce blood ALT concentration in broilers.
Immune responses
The current study was conducted to investigate whether the increasing levels dietary digestible SAAs may offer an improved immune response of broilers inoculated with ND and AI vaccines. Methionine is not only the first limiting amino acid for broiler growth but has also been shown to play vital roles in immune function. Methionine is a precursor of polyamines required for immune cell proliferation, induction/regulation of inflammation, and pathogen recognition (Ren et al. Citation2020). Met deficiency may impair the immune system and metabolic disorders (Rehman et al. Citation2019). A significant increase in the immune response to ND and AI vaccination in a quadratic trend was recorded in the present study by DL-Met supplementation. Higher antibody titre for ND and AI vaccination was observed in chicks fed on the diets that contained up to 0.73% digestible SAAs, whereas adding above this amount did not cause more improvement in antibody titre (Table , Figures and ). It was reported that deficiency of dietary protein or amino acids impairs immune system activity and increases the susceptibility of animals and humans to infectious diseases (Afkhami et al. Citation2020). Methionine plays a key role in the humoral and cellular immune function (Jankowski et al. Citation2014). Our founding demonstrated that SAAs have a beneficial influence on immune and inflammatory responses. One of the mechanisms proposed to explain methionine interference on immunity is based on the proliferation of cells sensitive to intracellular variations glutathione and Cys levels (Shini et al. Citation2005). Amino acids are needed for clonal proliferation of lymphocytes, the establishment of germinate centre in the bursa of Fabricius to refine immunoglobulin affinity, recruitment of new bone marrow, and synthesis of effector molecules immunoglobulins (Swain and Johri Citation2000).
Estimated digestible sulphur amino acids requirements
In the present study, the digestible SAAs requirements estimated by the best-fitted regression models (the highest adj. R2 and the lowest RMSE and AIC) to optimise LBW, WG, FE, RBW, and immune responses to ND and AI vaccination were 0.73, 0.69, 0.78, 0.86, 0.73, and 0.72%, respectively (Table and Figures ). However, varying amounts of the digestible sulphur amino acids requirement have been reported (Table ). Published estimates of the broiler chicken’s digestible SAAs requirement are pretty variable and range from 0.68% (Faridi et al. Citation2016) to 0.8–1.0% (Ren et al. Citation2020). Several factors might have caused a wide range in the requirements among those experiments. First, it may be due to a variation in methionine sources whose bioavailability’s differed. Second, different breeds and ages of chickens require different amounts of methionine and total sulphur amino acids. Third, various basal diets and experimental conditions that may cause variation were used (Chamruspollert et al. Citation2002). Amino acid requirements can vary depending on the dietary protein source and quality, dietary energy level, genetic strain, sex, experimental conditions, and statistical evaluation (Rehman et al. Citation2012; Taghinejad-Roudbaneh et al. Citation2013).
Table 8. A comparison of the digestible sulphur amino acids (DSAAs) requirements of male broiler chicks determined with different parameters.
Our result in this experiment approved that estimation of amino acid requirements depends on what production parameter is taken into consideration for optimised. The estimated digestible SAAs requirement for optimised FE was 13.04 higher than WG. It is well known that Met supplementation improves amino acid balance, and consequently, promotes growth by increasing protein synthesis and decreasing fat synthesis, leading to enhanced feed efficiency (Majdeddin et al. Citation2019). Concerning digestible SAAs responses, it has been demonstrated that requirements for maximal gain to feed often exceed weight gain requirements (Goulart et al. Citation2011; Faridi et al. Citation2016; Pontin et al. Citation2018; Ghavi et al. Citation2020) thus corroborating the current findings. This would be even more prominent with modern high breast meat yielding broiler strains (Majdeddin et al. Citation2019). In the current experiment, the effects of graded levels of Met were particularly notable on the RBW trait. The estimated digestible SAAs requirement for optimised RBW was the highest amount (0.86%). These findings confirmed the results of other researchers that levels of Met to maximise breast meat yield appear to be greater than requirements to optimise gain (Kalinowski et al. Citation2003; Pontin et al. Citation2018; Ghavi et al. Citation2020). The anabolic effect of Met is partly due to increased substrate supply at the sites of protein synthesis; however, recent insights have highlighted its action as a signal molecule in the regulation of protein metabolism (Majdeddin et al. Citation2019). Other studies have confirmed that, in fast-growing chickens, supplementary Met promotes anabolism and reduces catabolic processes, favouring muscle protein synthesis (Vesco and Gasparino Citation2013 ; Wen et al. Citation2017), though others did not find any conclusive evidence (Zeitz et al. Citation2019) .
The values estimated by quadratic broken-line for LBW, WG, FE, RBW, and immune response to ND and AI vaccination were 6.85, 17.39, 6.85, 16.22, 7.35, and 5.88% higher than those estimated by the linear broken-line fit model, respectively. In agreement with our results in this study, reported that the method of statistical evaluation could influence the estimation of an AA requirement (Taghinejad-Roudbaneh et al. Citation2013; Akbari Moghaddam Kakhki et al. Citation2016; Ghavi et al. Citation2020). The most common statistical models used to determine AA requirements are the broken-line (one-slope), two slopes, quadratic, and ascending quadratic with plateau models (Taghinejad-Roudbaneh et al. Citation2013). The result of this experiment closed with the results of other researchers that found quadratic procedure estimates were higher for all protein sources when compared with the straight broken-line models (Taghinejad-Roudbaneh et al. Citation2013; Ghavi et al. Citation2020).
In the current study, the ratio of dietary digestible SAAs/Lys in the basal diet was 51%, graded levels of supplementary DL-Met (+0.7 g/kg) led to increasing digestible SAAs/Lys ratio to 82%. The ratio of the digestible SAAs/Lys values; estimated by the best-fitted regression models for optimise LBW, WG, FE, RBW, and immune responses to ND and AI vaccination were 60, 67.82, 74.78, 63.48, and 62.61% for WG, FE, RBW, and immune responses to ND and AI vaccination, respectively. With regards to this, the digestible SAAs/Lys estimated for RBW equal 74.78%, that is agrees with previously reported digestible SAA/lysine 75% or above (Goulart et al. Citation2011; Dozier and Mercier Citation2013). Aviagen (Citation2019) recommended a ratio of digestible SAAs/Lys of 75.65% for the grower period. Spek (Citation2018) suggested minimal SAA/Lys ratios of 0.73–0.74 for maximum growth-maximum feed efficiency. An optimum dig TSAA to Lys ratios were estimated to be 77% for feed conversion from d 1 to 14 (Dozier and Mercier Citation2013). Goulart et al. (Citation2011) reported the level of digestible SAAs, resulting in better weight gain of chickens, was 0.93%, and the ratio of digestible SAAs to dig. Lys was 71%.
In this experiment, dietary digestible SAAs level effect on the immune response to ND and AI vaccination showed a quadratic trend (Figures and ). By increasing dietary digestible SAAs level to higher than 0.73% depressed antibody responses to ND and AI vaccination, in agreement with our result, Bhargava et al. (Citation1970) reported that the antibody response (to Newcastle Disease Virus) was higher in broilers fed diets with 0.3–0.6% methionine than those fed diets with 0.7 to 1.1% methionine. Similarly, Bhargava et al. (Citation1971) reported that antibody response (to Newcastle Disease Virus) was higher in broilers fed 0.4% methionine than in broilers fed 0.7 and 1.1% methionine. In this sense, the marginal requirement for digestible SAAs is narrow in broilers when both weight gain and immunity are considered. One possible explanation for the lack of observed weight gain and immune response to increasing dietary digestible SAAs could be related to ratios of essential amino acids. By increasing a single amino acid in the feed formulation, we may have inadvertently created a limitation in another limiting amino acid required for an efficient immune response (Ren et al. Citation2020). In contrast, the above reports and our result, reported that the methionine requirement for better health and immunity is more than for optimum weight gain (Swain and Johri Citation2000; Ren et al. Citation2020).
Conclusion
The outcomes of the present study showed that weight gain, breast yield, feed efficiency, and immunity improved in response to increasing dietary digestible SAAs concentration. Digestible sulphur amino acid requirements of broiler chickens during the grower period were estimated about 0.69–0.86% of the diet as-fed basis. The highest and the lowest were estimated for relative breast weight and weight gain, respectively. Estimated digestible SAAs requirement in the grower period of Ross‐308 meal broiler chickens was clearly under strain recommended level. Although, the result for breast yield was near strain recommendation. Overall, the estimation of digestible SAAs requirement depends on what parameter is taken into consideration for optimisation.
Ethical approval
The experiment was conducted with the approval of the Animal Care Committee of the Ferdowsi University of Mashhad (Mashhad, Iran).
Acknowledgment
We greatly appreciate our colleagues from the Poultry Research Station and Animal Nutrition Laboratory for their valuable help, critiques, and suggestions and the Iran branch of Evonik Co. for the feedstuffs amino acid analysis. This work was supported by a grant from the Ferdowsi University of Mashhad, Iran (Grant Number: 3/50173).
Disclosure statement
No potential conflict of interest was reported by the author(s).
References
- Afkhami M, Kermanshah IH, Majidzadeh Heravi R. 2020. Evaluation of whey protein sources on performance, liver antioxidants and immune responses of broiler chickens challenged with ethanol. J Anim Physiol Anim Nutr. 104(3):898–908.
- Agostini PS, Dalibard P, Mercier Y, Van der Aar P, Van der Klis JD. 2015. Comparison of methionine sources around requirement levels using a methionine efficacy method in 0 to 28 day old broilers. Poult Sci. 95(3):560–569.
- Akbari Moghaddam Kakhki R, Golian A, Zarghi H. 2016. Effect of digestible methionine + cystine concentration on performance, egg quality and blood metabolites in laying hens. Br Poult Sci. 57(3):403–414.
- Allahdo P, Ghodraty J, Zarghi H, Saadatfar Z, Kermanshahi H, Edalatian Dovom MR. 2018. Effect of probiotic and vinegar on growth performance, meat yields, immune responses, and small intestine morphology of broiler chickens. Ital J Anim Sci. 17(3):675–685.
- AOAC. 2002. Official methods of analysis. 17th ed. Washington, DC, USA: AOAC International.
- Aviagen. 2019. Ross 308: broiler nutrition specification. Huntsville (AL): Aviagen.
- Bhargava K, Hanson R, Sunde M. 1970. Effects of methionine and valine on antibody production in chicks infected with Newcastle disease virus. J Nutri. 100(2):241–248.
- Bhargava K, Hanson R, Sunde M. 1971. Effects of methionine and valine on growth and antibody production in chicks infected with live or killed Newcastle disease virus. Poult Sci. 50:614–619.
- Chamruspollert M, Pesti GM, Bakalli RI. 2002. Determination of the methionine requirement of male and female broiler chicks using an indirect amino acid oxidation method. Poult Sci. 81(7):1004–1013.
- Conde-Aguilera JA, Cobo-Ortega C, Tesseraud S, Lessire M, Mercier Y, Van Milgen J. 2013. Changes in body composition in broilers by a sulfur amino acid deficiency during growth. Poult Sci. 92(5):1266–1275.
- Vesco APD, Gasparino E. 2013. Production of reactive oxygen species, gene expression, and enzymatic activity in quail subjected to acute heat stress. J Anim Sci. 91(2):582–587.
- Diaz M, Lopez F, Hernandez F, Urbania JA. 2000. L-carnitine effects of chemical composition of plasma lipoproteins of rabbit fed with normal and high cholesterol diets. Lipids. 35:627–632.
- Dozier WA, Mercier Y. 2013. Ratio of digestible total sulfur amino acids to lysine of broiler chicks from 1 to 15 days of age. J Appl Poult Res. 22(4):862–871.
- Faridi A, Gitoee A, Sakomura NK, Donato DCZ, Angelica Gonsalves C, Feire Sarcinelli M, Bernardino de Lima M, France J. 2016. Broiler responses to digestible total sulphur amino acids at different ages: a neural network approach. J Appl Anim Res. 44(1):315–322.
- Ghavi S, Zarghi H, Golian A. 2020. Effect of dietary digestible sulphur amino acids level on growth performance, blood metabolites and liver functional enzymes of broilers 1–11 days of age. Ital J Anim Sci. 19(1):1439–1449.
- Goulart CC, Costa FGP, Silva JHV, Souza JG, Rodrigues VP, Oliveira CFS. 2011. Requirements of digestible methionine + cystine for broiler chickens at 1 to 42 days of age. R Bras Zootec. 40(4):797–803.
- Grimble RF. 2006. The effects of sulfur amino acid intake on immune function in humans. J Nutr. 136:1660S–1665S.
- Jahanpour H, Seidavi A, Qotbi AAA, Van Den Hoven R, Rocha e Silva S, Laudadio V, Tufarelli V. 2015. Effects of the level and duration of feeding restriction on carcass components of broilers. Arch Anim Breed. 58(1):99–105.
- Jankowski J, Kubińska M, Zduńczyk Z. 2014. Nutritional and immunomodulatory function of methionine in poultry diets–a review. Ann Anim Sci. 14(1):17–32.
- Kalinowski A, Moran ET, Jr, Wyatt CL. 2003. Methionine and cystine requirements of slow-and fast-feathering broiler males from three to six weeks of age. Poult Sci. 82(9):1428–1437.
- Kanani PB, Daneshyar M, Najafi R. 2016. Effects of cinnamon (Cinnamomum zeylanicum) and turmeric (Curcuma longa) powders on performance, enzyme activity, and blood parameters of broiler chickens under heat stress. Poult Sci J. 4(1):47–53.
- Majdeddin M, Golian A, Kermanshahi H, Michiels J, De Smet S. 2019. Effects of methionine and guanidinoacetic acid supplementation on performance and energy metabolites in breast muscle of male broiler chickens fed corn-soybean diets. Br Poult Sci. 60(5):554–563.
- Moran JR, Edwin T. 1994. Response of broiler strains differing in body fat to inadequate methionine: live performance and processing yields. Poult Sci. 73(7):1116–1126.
- Muramatsu K, Odagiri H, Morishita S, Takeuchi H. 1971. Effect of excess levels of individual amino acids on growth of rats fed casein diets. Nutr J. 101(9):1117–1125.
- Ohta Y, Ishibashi T. 1995. Effect of dietary glycine on reduced performance by deficient and excessive methionine in broilers. Jpn Poult Sci. 32(2):81–89.
- Pesti GM, Vedenov D, Cason JA, Billard L. 2009. A comparison of methods to estimate nutritional requirements from experimental data. Brit Poult Sci. 50(1):16–32.
- Pontin CA, Vieira SL, Stefanello C, Kipper M, Kindlein L, Simões CT, Gonzalez-Esquerra R. 2018. Estimation of broiler responses to increased dietary methionine hydroxy analogue [DL-2-hydroxy-(4-methylthio) butanoic acid] using linear and nonlinear regression models. Poult Sci. 97(3):865–873.
- Rehman AU, Arif M, Husnain MM, Alagawany M, El-Hack A, Mohamed E, Taha AE, Elnesr SS, Abdel-Latif MA, Othman SI. 2019. Growth performance of broilers as influenced by different levels and sources of methionine plus cysteine. Animals. 9(12):1056.
- Rehman H, Ghumro PB, Dino G, Khan SH, Hussain Z, Ahmed S, Hameed A. 2012. Substitution of crystalline l-lysine with l-lysine enriched fermentation broth in feed and effect on the performance of broiler chicks. J Appl Anim Res. 40(2):118–123.
- Ren Z, Bütz DE, Whelan R, Naranjo V, Arendt MK, Ramuta MD, Yang X, Crenshaw TD, Cook ME. 2020. Effects of dietary methionine plus cysteine levels on growth performance and intestinal antibody production in broilers during Eimeria challenge. Poult Sci. 99(1):374–384.
- Rezaeipour V, Alinejad H, Asadzadeh S. 2016. Growth performance, carcass characteristics and blood metabolites of broiler chickens fed diets formulated on total or digestible amino acids basis with bovine bile salts powder and soybean oil. J Cent Eur Agric.17(2):272–284
- Robbins KR, Saxton AM, Southern LL. 2006. Estimation of nutrient requirements using broken-line regression analysis. J Anim Sci. 84(suppl_13):E155–E165.
- Schutte JB, De Jong J, Smink W, Pack M. 1997. Replacement value of betaine for DL-methionine in male broiler chicks. Poult Sci. 76(2):321–325.
- Schutte JB, Pack M. 1995. Sulfur amino acid requirement of broiler chicks from fourteen to thirty-eight days of age. 1. Performance and carcass yield. Poultr Sci. 74(3):480–487.
- Scott ML, Nesheim MC, Young RJ. 2002. Proteins and amino acids. Pages 58–118 in nutrition of chicken. Ithaca, NY: M. L. Scott and Association Publisher.
- Shini S, Li X, Bryden WL. 2005. Methionine requirement and cell-mediated immunity in chicks. Asia Pac J Clin Nutr. Supplement, Vol. 14, pS123-S123.
- Sigolo S, Deldar E, Seidavi A, Bouyeh M, Gallo A, Prandini A. 2019. Effects of dietary surpluses of methionine and lysine on growth performance, blood serum parameters, immune responses, and carcass traits of broilers. J Appl Anim Res. 47(1):146–153.
- Spek JW. 2018. Standardized ileal digestible methionine and cysteine requirement for broilers. CVB documentation report nr. 63. Wageningen Livestock Research. p. 50. Wageningen, Netherlands.
- Swain BK, Johri TS. 2000. Effect of supplemental methionine, choline and their combinations on the performance and immune response of broilers. Br Poult Sci. 41(1):83–88.
- Tabatabaei Yazdi F, Golian A, Zarghi H, Varidi M. 2017. Effect of wheat-soy diet nutrient density and guanidine acetic acid supplementation on performance and energy metabolism in broiler chickens. Ital J Anim Sci. 16(4):593–600.
- Taghinejad-Roudbaneh M, Babaee MJ, Afrooziyeh M, Alizadeh B. 2013. Estimation of dietary threonine requirement for growth and immune responses of broilers. J Appl Anim Res. 41(4):474–483.
- Wen C, Jiang X, Ding L, Wang T, Zhou Y. 2017. Effects of dietary methionine on breast muscle growth, myogenic gene expression and IGF-I signaling in fast-and slow-growing broilers. Sci Rep. 7(1):1–7.
- Wu BY, Cui H-M, Xi P, Jing F, Wei C, Liu X. 2012. Effect of methionine deficiency on the thymus and the subsets and proliferation of peripheral blood T-cell, and serum IL-2 contents in broilers. J Integr Agric. 11(6):1009–1019.
- Xie M, Hou SS, Huang W, Zhao L, Yu JY, Li WY, Wu YY. 2004. Interrelationship between methionine and cystine of early Peking ducklings. Poult Sci. 83(10):1703–1708.
- Yoo J, Yi YJ, Wickramasuriya SS, Kim E, Shin TK, Cho HM, Kim N, Heo JM. 2017. Evaluation of sulphur amino acid requirement of male Korean native ducklings from hatch to 21 day of age. Br Poult Sci. 58(3):272–277.
- Zarghi H, Golian A, Nikbakhtzade M. 2020a. Effect of dietary digestible lysine level on growth performance, blood metabolites and meat quality of broilers 23–38 days of age. J Anim Physiol Anim Nutr. 104(1):156–165.
- Zarghi H, Golian A, Tabatabaei YF. 2020b. Effect of dietary sulphur amino acid levels and guanidinoacetic acid supplementation on performance, carcass yield and energetic molecular metabolites in broiler chickens fed wheat-soy diets. Ital J Anim Sci. 19(1):951–959.
- Zeitz JO, Mohrmann S, Käding SC, Devlikamov M, Niewalda I, Whelan R, Helmbrecht A, Eder K. 2019. Effects of methionine on muscle protein synthesis and degradation pathways in broilers. J Anim Physiol Anim Nutr. 103(1):191–203.