Abstract
This study investigated the effects of low-cost dried yeast and multi-strain probiotics on laying hens. A total of 108 Charoen Pokphand (CP) brown hens aged 25 weeks were used in a completely randomised design (CRD) experiment involving 18 cages of six hens per replicate and six replicates per group. The hens were allocated to three groups: basal diet (BD, control), BD plus dried yeast (additive-fed), and BD plus dried yeast and probiotics (probiotic-fed). The hens were fed the BD for 2 weeks, the experimental diet for 4 weeks, and the BD for 4 weeks, and the ends of these periods were termed before feeding (BF), during feeding (DF) and after stopping feeding (ASF). At DF, the additive-fed group showed the highest egg production and % albumen, and at ASF, the Haugh unit (HU) was higher than that of the probiotic-fed group. At DF, the probiotic-fed group exhibited the most intense yolk colour and the highest eggshell thickness. Only the probiotic-fed group produced jumbo and extra-large size eggs. The expression of the immune response-related genes IL-1β, IL-2, IFNγ and HSP90 did not differ among the groups and feeding periods with the exception of the IL-2 level of the additive-fed group at ASF, which was significantly lower than that of the control. Thus, dried yeast delivered probiotics effectively and thereby improved the egg production and quality and the immune response, and the combination of yeast with probiotics induced synergistic increases in the yolk colour and egg size (p < .05, .01).
Yeast supplementation improved egg production and quality and the immune response of 25- to 35-week-old laying hens.
The addition of yeast combined with probiotics synergistically increased yolk colour and egg size.
Yeast additive reduced the expression of the signalling cytokine IL-2, and the effect lasted for at least 4 weeks.
HIGHLIGHTS
Introduction
Enhanced egg production and quality could economically contribute to the profitability of commercial egg producers. Hence, monitoring and management of contamination of laying hens with some pathogens, such as Salmonella and Campylobacter, are essential, and antibiotics have been commonly used for the treatment. The use of medication and chemicals has been minimised or banned to avoid accumulation of antibiotic residues and prevent the emergence of antibiotic-resistant bacteria that can contaminate poultry products and pose a serious threat to human health (Attia et al. Citation2020). Consequently, supplementation of the diet with prebiotics, probiotics or synbiotics is an environment friendly and safe potential alternative approach in poultry production (Osman et al. Citation1996; Attia et al. Citation2012).
Prebiotics are the substances that act as substrates to promote the growth of probiotics colonising the digestive tract (Pourabedin and Zhao, Citation2015). Probiotics are defined as live microorganisms that improve host health by balancing the microflora in the intestines (FAO/WHO, Citation2002). They can utilise prebiotics in a specific manner, balance the intestinal microflora, produce digestive enzymes and antimicrobial peptides, reduce stress and modulate the protective immunity of the host against several pathogens (Levkut et al., Citation2012; Wang et al. Citation2018). Mixtures of prebiotics and probiotics are termed as synbiotics, and they could benefit host physiology and productivity via several mechanisms, such as by balancing the microbe population in the gut, promoting homeostatic balance and improving the immune system (Pineda-Quiroga et al., Citation2019; Al-Ajeeli et al. Citation2020). Examples of prebiotics that have been investigated as growth promoters in poultry are live yeast culture (Osman et al. Citation1996; Yalcin et al. Citation2015; Attia et al. Citation2020; Zhang et al. Citation2020), baker’s yeast (Hameed et al. Citation2019), whole yeast autolysate (Yalcin et al. Citation2015), yeast cell wall (Ahiwe et al. Citation2020), yeast with bacteriocin and Lactobacillus culture (Chen et al. Citation2016), marine-derived polysaccharides (Guo et al. Citation2020), chitosan and chitooligosaccharides (Swiątkiewicz et al. Citation2015; Xu et al. Citation2020).
The live yeast culture and the yeast cell wall improved the internal and external egg quality of laying hens (Hashim et al., Citation2013; Zhang et al. Citation2020). Inactive yeast (0.1%) resulted in the production of low-cholesterol eggs (Yalcin et al. Citation2015). Supplementation with baker’s yeast at 0.15–0.2% for 10 weeks was sufficient to increase the layer performance via a significant improvement in yolk weight, albumin height, Haugh unit (HU) and grading value (Hameed et al. Citation2019). The supplementation of the marginally-nutrient-deficient diet with 0.02% or 0.04% active yeast improved the growth performance of broilers (Attia et al. Citation2020). The addition of dried yeast containing 1 mg of bacteriocin (albusin B) per 1 g of yeast product enhanced blood parameters in broilers by reducing the cholesterol and low-density protein levels (Chen et al. Citation2016). When dried yeast plus bacteriocin were fed in combination with Lactobacillus culture, they improved the body weight gain and feed conversion ratio (FCR) of broilers.
The most frequently studied probiotics, including those of the genera Lactobacillus, Enterococcus and Pediococcus, which are classified as lactic acid bacteria (LAB), are able to survive in and colonise in the intestine. Non-colonising species include spore-producing Bacillus spp. and the yeast Saccharomyces cerevisiae (Kabir, Citation2009; Tellez et al., Citation2012). As spore-forming species, Bacillus spp. strains are the most promising feed supplements in the poultry industry, because their spores can tolerate the low-pH in the gastrointestinal tract (Hong et al. Citation2005; Shivaramaiah et al., Citation2011). B. subtilis has been recognised as safe for inclusion in animal diets. Dietary supplementation with B. subtilis induces many positive responses in laying hens, such as improvements in egg production and feed efficiency (Mazanko et al., Citation2018; Guo et al., Citation2020); increases in the yolk colour score, HU and eggshell quality (Liu et al., Citation2019; Upadhaya et al., Citation2019); modulation of the immune response (Chen et al. Citation2019); and decreased oxidative stress (Liu et al., Citation2019). B. subtilis-based probiotics also reduced heat stress-related behaviours and the inflammatory response in broilers (Wang et al. Citation2018).
Due to the growth-promoting effects of probiotics, the utilisation of prebiotics in particular animals is based on their biological function, formulation, safety, delivery route, cost and technological usability. Previously, we characterised some strains of B. subtilis and LAB isolated from the guts of broilers and native fowl that exhibit probiotic properties in broilers (Khochamit et al. Citation2015; Buahom et al. Citation2018; Khochamit et al. Citation2020), but their probiotic properties in laying hens have not yet been investigated. In the present study, all strains were selected according to their antibacterial activity against some pathogens, including Salmonella spp. and showed no antagonistic action against each other. Dried brewer’s yeast (S. cerevisiae), a non-living yeast that remains after the brewing process, is considered as a prebiotic that can aid animal digestion and act as a nutrient source for probiotics (Attia et al. Citation2020). Among all the natural growth promoters used in poultry production, S. cerevisiae is one of the most prominently reviewed in the literature (Ogbuewu et al. Citation2019). Yeast is rich in digestible proteins, vitamins and many beneficial mineral elements. The yeast cell wall contains mannan oligosaccharide (MOS) and fructooligosaccharide (FOS) dietary fibres that acts as a prebiotic to enhance the growth of beneficial gut bacteria in broilers (Yang et al. Citation2008; Attia et al. Citation2020).
Whole dried yeast, a byproduct of the brewery industry, was selected as a feed additive due to its low-cost, rich nutrition and prebiotic properties. In addition, its effects when delivered in combination with probiotics as a synbiotic in laying hens could be evaluated. Age is one of the factors that affects the health status and laying performance of hens. The early phase is the stage when hens begin to lay their eggs and can have long-term effects on their overall laying performance. Early-phase laying hens (25 weeks old) were selected for this study.
Materials and methods
Bacterial strains and culture conditions
B. subtilis CE330 (MN848256) and D13 (MT800818) were isolated from the caecum of broiler chickens. Six strains (four species) of LAB were isolated from different parts of broiler chickens: Enterococcus faecium BL1 from the duodenum and BL4 from the colon, Enterococcus durans CH33 (MF066896) and Lactobacillus salivarius CH24 (MF066895) from the intestine (Buahom et al. Citation2018) and E. faecalis BL22 (MT807902) from the duodenum and BL11 from the colon. In addition, E. faecium CA4 (MF066894) and NL4 were isolated from the caecum and duodenum of domestic fowl, respectively. The selected B. subtilis and LAB strains showed antibacterial activity against both Gram-positive and Gram-negative bacterial pathogens (Buahom et al. Citation2018). They were all resistant to low-pH and bile salts. The two B. subtilis strains effectively produced extracellular enzymes, including protease, cellulase, lipase and amylase. All strains were cultivated in broth medium (0.5% yeast extract, 1% tryptone and 2% glucose). B. subtilis and LAB were grown under aerobic conditions at 42 °C with shaking at 150 rpm for 24 h and under static conditions at 37 °C for 48 h, respectively.
Bacterial cell immobilisation on the additives by mixing and freeze drying
The yeast additives were prepared by mixing 80 g of dried brewer’s yeast, 10 g of chitosan and 10 g of carboxy methyl cellulose (CMC) at an 8:1:1 (w/w) ratio and sterilised by autoclaving at 121 °C for 15 min. A total of 12 g of yeast additives was mixed with 108 g of basal diet. Thus, one hen of the additive-fed group was fed daily with 120 g of basal diet plus yeast additives (8% dried yeast + 1% chitosan + 1% CMC).
For the preparation of probiotics immobilised on yeast additives, all bacterial strains were individually cultivated under the above-described conditions. Then, 25 g of sterile yeast additives were added to 100 ml of each bacterial culture at a 1:4 (w/v) ratio and mixed at 37 °C with shaking at 150 rpm for 1 h. The bacterial cells and yeast additives were collected by centrifugation at 9000 rpm, washed twice with sterile dH2O, frozen at −80 °C overnight and dried in a freeze-dryer (LabGeneTM, Lemvig, Denmark) for 72 h. The viable count of the freeze-dried bacterial cells was determined by spreading on Luria–Bertani (LB) agar for B. subtilis and on de Man, Rogosa and Sharpe (MRS) agar for LAB and incubating at 42 °C for 24 h and at 37 °C for 48 h, respectively. The viable freeze-dried cells of each bacterial strain were counted and prepared as 1 × 106 colony forming units (CFUs). All 10 strains were mixed and adjusted to a total of 1 × 107 CFUs/12 g of yeast additives. 12 g of yeast additive plus probiotics was mixed with 108 g of basal diet. Thus, one hen of the probiotic-fed group was fed daily with 120 g of basal diet plus yeast additives (8% dried yeast + 1% chitosan + 1% CMC) and 1 × 107 CFUs of multi-strain probiotics.
Hens, diets and experimental design
A total of 108 Charoen Pokphand (CP) brown laying hens (aged 25 weeks) obtained from the commercial company were wing-banded. After a 1 week adaptation period in the cages (45 cm length × 40 cm width × 35 cm height), the hens were allocated to 18 cages of six hens per replicate (two hens per cage), and six replicates of each group were included in the study. The hens were divided into three dietary groups: basal diet (control group), basal diet plus additives (additive-fed group), and basal diet plus probiotics (107 CFUs/g) immobilised on additives (probiotic-fed group). The hens were fed the commercial basal diet (corn-soybean meal) prior to the beginning of the experiment. The commercial basal diet was formulated to contain 35% corn and 15% soybean meal, and the nutritional level of the corn-soybean meal basal diet is presented in Table .
Table 1. Chemical composition of the basal diet.
Housing and husbandry
The cages were equipped with a nipple drinker and trough feeders, and the hens were housed in a semi-opened laying house with a temperature of approximately 30–35 °C and fan air-circulation. The 10-week trial was divided into three periods: the hens were fed the basal diet for the first 2 weeks, the corresponding experimental diet for the next 4 weeks and the basal diet for the last 4 weeks, and the ends of these periods were termed before feeding (BF), during feeding (DF) and after stopping feeding (ASF), respectively. All the diets and additives were prepared every other week. The hens were allowed ad libitum access to water throughout the experiment. The hens were exposed to light for a total of 16 h, which included natural light for 12 h from 6:00 am to 6:00 pm and light provided by an electric lamp (5 lux) for 4 h from 6:00 to 10:00 pm.
Egg collection and laboratory analysis
The individual egg production (EP) was recorded daily and maintained throughout the experiment. A total of 108 eggs were collected from each group at BF, DF and ASF, and a total of 324 eggs were used for the analyses of EP. The following equations were used for the calculation of EP:
Total eggs laid by each group at BF, DF, or ASF/Total experimental period (in days)=X1, X2 or X3
X1 + X2 + X3/Total eggs tested =Y1, Y2, Y3
Y1, Y2, Y3/Total replicates × 100 = % egg production
Two fresh eggs from each cage were individually weighted and used for determination of the egg weight (EW), egg mass (EM), egg size, feed intake and FCR. Thus, a total of 36 eggs from 18 cages per group were analysed. The EM was calculated as EM = EW × EP/100. The feed intake was assigned and fixed to 120 g per hen per day. The FCR was calculated based on the gram of the basal diet fed per gram of EM during each experimental period. The egg size specifications in Thailand were used to give the eggs a score in the range of 0–5 based on their weight: 0, >70 g; 1, 65–69 g; 2, 60–64 g; 3, 55–59 g; 4, 50–54 g; and 5, 45–49 g.
A total of 36 eggs (six eggs per replicate) were randomly collected from each group between 09:00 and 12:00 h for the assessment of various egg quality-related parameters. The egg quality parameters, including the % yolk, % albumen, HU, % eggshell weight (SW), eggshell thickness, colour score and egg size, were evaluated every week of the experiment (Burke and Attia, Citation1994; Attia, Burke, Yamani Citation1994, Attia, Burke, Yamani, Jensen Citation1995). The eggs were cracked, and the albumen and yolk were separated. The egg yolk was then separated from the albumen using a spatula, and the yolk was placed on blotting paper to remove the albumen before the yolk was weighed (YW). The % albumen and % yolk (YW) were determined by dividing the AW and YW by the weight of the fresh egg multiplied by 100.
The albumen height was measured using a QCH electronic height gauge (TSS QCM System, York, UK). Shells with a membrane were washed, dried at 50 °C for 7 days and weighed to obtain the SW. The albumen weigh (AW) was calculated as the difference between EW and the sum of YW and SW. The HU was determined using the following formula: HU = 100log (H + 7.57 − 1.7W0.37), where HU is the Haugh unit, H is the albumen height (mm) and W is the egg weight (g).
The yolk colour score was determined based on a 15-point scale (1, light yellow; 15, orange) according to the Roche yolk colour fan (Roche Co., Basel, Switzerland). Shells were rinsed free of albumen and air dried for 48 h, and the shell plus membrane was then used to determine the SW. The % SW was calculated as the dry SW without the eggshell membrane divided by the EW multiplied by 100. The eggshell thickness (without the eggshell membrane) was measured at three sites, namely, the air cell, equator and sharp end, using a digital micrometre calliper (TSS QCT System, York, UK), and the mean value from the three parts was calculated.
Assessment of effects on the immune system by quantitative real-time PCR (RT-PCR)
At DF, DF and ASF, blood samples from 18 randomly selected hens from each group (one per replicate, six hens per treatment) were collected from the vena brachialis under the wing into a lithium heparin (anticoagulant) tube. A 1 ml whole blood sample was collected from each hen and centrifuged at 3,000 rpm for 5 min, and the buffy coat containing leukocytes was then transferred to a new centrifuge tube. Total RNA was extracted using the AurumTM Total RNA Mini Kit (Bio-Rad, Hercules, CA, USA) according to the manufacturer’s instructions. Total RNA was reverse transcribed to cDNA using reverse transcriptase. Briefly, 16 µl of the following reaction mixture was combined with 4 µl of the RNA sample: 3.4 µl of H2O DEPC, 0.4 µl of RiboSafe RNase inhibitor (Bioline, Meridian Bioscience, OH, USA), 0.2 µl of reverse transcriptase, 10 µl of 2x SensiFAST No-ROX One-Step Mini Kit (Bioline), 1 µl of forward primer and 1 µl of reverse primer. The mRNA abundances of the target genes were analysed by real-time PCR. The sequences of the IL-1β, IL-2, IFNγ and HSP90 primers for laying hens and broilers are shown in Table , and 18S rRNA was used as an internal control. The reactions were incubated in a 96-well plate at 95 °C for 5 min and then subjected to 40 cycles of 95 °C for 10 s, 60 °C for 40 s and 72 °C for 90 s. Samples without RNA were included in the PCR assay as a negative control. The data were analysed using the 2-D CT method (Livak and Schmittgen, Citation2001).
Table 2. Primer sequences of immune response-related genes used in the quantitative RT-PCR assay.
Statistical analysis
The experimental trial included 108 Charoen Pokphand (CP) brown laying hens aged 25–34 weeks. The hens were assigned to one of three groups according to the completely randomised design (CRD) experiment, each group contained 18 cages and 36 hens belonging to six replicates, and each replicate consisted of six hens. All the data were subjected to one-way ANOVA (JMP Statistical DiscoveryTM from SAS Version 14.1.0). The significance of the differences among the treatment groups was determined by Tukey’s honestly significant difference test. Multiple comparisons of the data were performed using a minimum range method, and the results are presented as the means ± SEMs. The differences were defined as statistically significant if p < .05.
Results
Egg production
The effects of yeast and/or probiotics on egg production are presented in Table . The egg production at the beginning of the trial (BF) was not significantly different among the groups, and the egg production profile, which consisted of a significant increase from BF to DF and remaining unchanged at ASF, was similar. The highest egg production (93.8%) at DF was found in the additive-fed group, followed by the probiotic-fed (86.3%) and control (85.3%) groups (p = .0455). When determining the improvement level from BF to DF, the egg production of the additive-fed group increased by 27.1%, whereas values of 22.1% and 15.3% were observed in the probiotic-fed and control groups, respectively. The additives had no effect on egg weight; nevertheless, at DF, the additive- and probiotic-fed groups tended to show improvements of 28.8% and 26.1% in egg mass, respectively, whereas 16.1% was found in the control group (p = .0866). Although the FCR did not significantly differ among all the groups, the additive-fed and probiotic-fed groups presented a lower FCR at 2.23 and 2.38, respectively, compared to that of the control group (2.47) at DF.
Table 3. Effects of dietary treatments on the performance of early-phase laying hens.
Egg quality
The effects of additive and/or probiotic supplementation on egg quality are presented in Table . The % yolk was not significantly different among the groups. The egg yolk colour at BF was not different among the groups. According to the testing periods, the colour showed a similar pattern, which involved an increase in intensity from BF to DF and remaining unchanged at ASF, and this finding might indicate a natural laying performance. However, at DF (during weeks 28–31), the yolk colour intensity of the probiotic-fed group was higher than those of the additive-fed and control groups (p = .0002).
Table 4. Effects of the dietary treatments on the quality of eggs produced by early-phase laying hens.
The additive-group showed the highest % albumen (p < .0001) at DF, and at ASF, this group presented a higher HU (p = .0344) than the probiotic-fed group, but the value did not differ from that of the control. The additive-fed group also increased its % albumen from BF to DF (p < .0001). In the probiotic-fed group, the HU varied, and the value at ASF was lower than those at BF and DF (p = .0188). The eggshell weight at DF did not differ among all the groups. The eggshell thickness of the additive-fed (p = .0001) and control (p = .0037) groups was reduced after BF. In the probiotic group, the thickness was reduced after DF (p = .0017). However, at DF, the eggshell thickness of the additive-fed and probiotic-fed groups was higher than that of the control (p = .0226).
Egg size and economic value
Overall, most hens produced medium-sized eggs in the size range of 2–4, and the size of most of the eggs (56.5%) was classified as 3. The control and additive-fed groups showed similar results and produced small eggs with a size of 5 (peewee) at BF and DF. At ASF, the number of size-3 eggs was higher in the additive-fed group (75.0%) than in the control group (61.1%). However, at DF, only the probiotic-fed group produced larger eggs, with sizes of 0 (jumbo) and 1 (extra-large), and none of the eggs produced had a size of 5, while eggs of size 5 were produced in the control and additive-fed groups at BF and DF. Based on a price analysis, according to Thailand’s values, the probiotic-fed group yielded the highest price of $29.3 per 300 eggs produced from all periods, followed by $29.0 and $28.9 for the additive-fed and control groups, respectively. According to the particular period, the highest price of $9.93 per 100 eggs was found at DF in the probiotic-fed group (Table ).
Table 5. Effects of the dietary treatments on the size of eggs produced by early-phase laying hens.
Expression of the immune response-related genes IL-1β, IL-2, IFNγ and HSP90
The expression of cytokines in each group was analysed relative to the control (Figure ). No significant differences in the expression of the proinflammatory cytokines IL-1β, IFNγ and HSP90 were found among the groups and periods. The expression of IL-2 at ASF (week 35) was significantly downregulated in the additive-fed group compared with the control group but did not differ from that in the probiotic-fed group (p<.05). However, comparison of the additive-fed group between periods showed that the IL-2 expression level changed 6.25-fold at BF (week 27), but the value decreased to 2.03-fold at DF (week 31) and 0.0905-fold at ASF (week 35). In the probiotic-fed group, the IL-2 gene expression levels changed 2.00-fold (BF), 0.545-fold (DF) and 0.368-fold (ASF). A similar profile was observed for the IL-1β gene: the expression levels in the additive-fed group changed 3.92-fold (BF), 0.0340-fold (DF) and 0.0025-fold (ASF). The expression of IFNγ in the additive-fed and probiotic-fed groups was overall lower than 1.00-fold that in the control. The expression of HSP90 in the additive-fed group changed 0.205-fold (BF), 2.23-fold (DF) and 0.237-fold (ASF). Based on the overall evaluation, the expression levels of IL-1β, IL-2, IFNγ and HSP90 were the lowest in the additive-fed group at ASF.
Figure 1. Effects of the dietary treatments on IL-1β, IL-2, IFNγ and HSP90 expression. The effects were calculated relative to the effects on the level of the housekeeping gene 18S rRNA in the control group. The bars represent the means ± SEMs. The letters a and b indicate a significant difference between groups at p<.05.
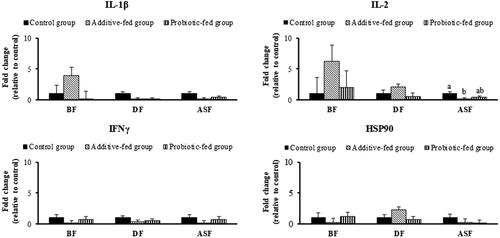
Discussion
Dried brewer’s yeast is rich in nutrients, and the value of S. cerevisiae in several forms as a prebiotic has been successfully evaluated. In this study, 8% yeast was selected as the main feed additive and potential prebiotic, while 1% CMC and 1% chitosan were used as probiotic carriers due to their advantageous biological, chemical and physical properties. In addition, chitosan is considered a prebiotic in poultry (Attia et al. Citation2012; Citation2020; Swiątkiewicz et al. Citation2015; Li et al. Citation2016). Although the biological effect of chitosan cannot be ruled out, the results observed in this study were discussed based on the impact derived mainly from dried yeast. The probiotics used in this study were composed of B. subtilis and LAB, originating from chickens and selected due to their probiotic properties in vitro and in broilers (Buahom et al. Citation2018; Khochamit et al. Citation2020). 10 strains screened from all sections of the chicken gastrointestinal tract were selected to cover all possible strains that are physiologically fitted to the digestive system of chickens. The additives and probiotics on the additive carriers were prepared in a dried granule form that can be conveniently mixed with the feed. In this study, the feed intake was limited to 120 g per day, which is the nutrient requirement level suitable for Charoen Pokphand (CP) brown laying hens to avoid fat accumulation. No leftover basal diets were detected through the experiment, demonstrating that the layers had sufficient feed for their growth and performance.
The yeast additive resulted in the highest egg production (93.8%) at DF and an increase of 27.2% from BF to DF in the additive-fed group, which indicated that MOS and FOS present in dried yeast might act as prebiotics to enhance the growth and digestibility of beneficial microbes that naturally colonise the chicken gut (Attia et al. Citation2020; Zhang et al. Citation2020). During the 2-week BF period, when the birds were fed a basal diet, egg production was not different among all groups, suggesting that the health status of all hens was the same. Hence, dried yeast was clearly a major factor that improved egg production during additive feeding. In the probiotic-fed group, the dried yeast might have served as a nutrient source for the delivered probiotics. Consequently, no difference in egg production was detected between the probiotic-fed and control groups at DF. However, at ASF, the probiotic-fed group exhibited increased egg production by 6.48%, which was higher than the 2.00% increase detected in the additive-fed and control groups. It is speculated that after stop feeding for 4 weeks, there might be multiple probiotics that exist in the gut and thus partly enhance the productive performance of laying hens.
A similar increase in EM (p = .0866) at DF was found in the additive-fed (28.8%) and probiotic-fed (26.1%) groups compared with 16.1% in the control group, which suggested that yeast and/or probiotics likely affected EM. These results were consistent with the increase in the number of jumbo-sized eggs produced by the probiotic-fed group (Table ). Probiotics can produce their own digestive enzymes and/or may increase the activities of host digestive enzymes (Zokaeifar et al. Citation2012; Khochamit et al. Citation2020) and improve the villus height (Lei et al. Citation2014), which possibly contributes to improved digestion and the absorption efficiency of laying hens (Osman et al. Citation1996). This speculation agrees with the trend showing that the additive-fed and probiotic-fed groups had lower FCRs of 2.23 and 2.38, respectively, than the control group (FCR of 2.47).
In this study, the probiotic-fed group presented a significantly more intense yolk colour than the additive-fed and control groups, and this result might be related to the biological function of probiotics and the presence of carotenoids (isomeric lutein and zeaxanthin) in corn, a major component of the basal diet used in this study (Abdel-Aal et al. Citation2013). The yeast S. cerevisiae does not naturally produce carotenoids (Mata-Gómez et al. Citation2014). Therefore, the similar egg yolk colours of the additive-fed and control groups could be derived from the carotenoids present in the corn. Liu et al. (Citation2019) reported that dietary B. subtilis C‐3102 significantly increases yolk colour, and this effect might be related to the dietary concentration of carotenoids, primarily xanthophylls. Egg yolk contains lutein at approximately 3.84–13.2 µg/g fresh weight, but lacks zeaxanthin (Abdel-Aal et al. Citation2013). The observed results suggested that the two probiotic strains of B. subtilis used in this study, namely, CE330 and D13, might facilitate the metabolic absorption and deposition of lutein and zeaxanthin (which can be converted to lutein) from the corn into the developing ova of laying hens. Although yolk colour does not directly affect the nutritional content except for the abundant carotenoids, it has an economic influence on the marketing, shelf life and consumer preference of eggs.
The % albumen and HU are important indices of the internal egg quality and are generally influenced by the hen age and strain and/or storage. However, these parameters are also enhanced by polysaccharide prebiotics (Guo et al. Citation2020). At DF, the highest % albumen was found in the additive-fed group. However, at ASF, the HU of the hens in the additive-fed group did not differ from that of the control group, but was higher than that of the probiotic-fed group. These findings demonstrated that the basal diet contains sufficient and efficient nutrients for ensuring normal egg quality and that supplementation with additives not only exerted no adverse effect but also improved this property. The decrease in the HU observed in the probiotic-fed group might indicate that these exogenous strains changed the balance of naturally existing gut microbes and interfered with the normal physiological digestion of hens.
The weight and thickness of the eggshell directly reflect the eggshell quality. The eggshell weight did not differ among all the groups, which indicated that yeast additive, cationic chitosan and probiotics have no adverse effect on the absorption of Ca2+ to form an eggshell. A difference in the eggshell thickness between groups was observed only at DF. The highest shell thickness was detected in the probiotic-fed group. Thus, probiotics might lower the luminal pH and improve intestinal epithelial function, which would facilitate the Ca and P absorption needed for eggshell formation (Li et al., Citation2006; Salajegheh et al. Citation2020). The eggshell thickness of the probiotic-fed group was reduced at ASF, when the hens were fed only a basal diet. The high eggshell thickness obtained for the probiotic-fed group also explains why jumbo-sized eggs were only obtained at DF in this group. This result might be due to an increase in the Ca density in the shell via an enhanced Ca metabolism in hens induced by the supplemented probiotics. This finding has had a significant impact on the economics of commercial egg production, because approximately 8% of all losses in egg production are due to poor eggshell quality (Salajegheh et al. Citation2020). The control of Ca metabolism in laying hens must be tightly regulated and effective, particularly during laying. Thus, understanding the role of Ca regulation by probiotics in laying hens should be further investigated.
The immune response determines the health status of humans and animals. RT-PCR is a highly sensitive method and has been used to detect the levels of immune response-related genes in whole blood samples from laying hens (Wigley and Kaiser, Citation2003). The expression of cytokines appears to be tightly regulated at the transcriptional level by restricted types of cells and inducers (Bunting et al. Citation2006). Thus, it is relatively clear that the expression level of immune-related genes detected in this study could indicate the level of secreted proteins in the blood. Interleukins are important components of cytokines in the innate and adaptive immune systems (Abdel-Mageed et al. Citation2017). IL-1β is a major proinflammatory cytokine produced by macrophages, monocytes and dendritic cells that mediates innate immunity. IL-2, a signalling cytokine that is functionally related to T-cells, often regulates the activities of lymphocytes responsible for protective immunity (Arenas-Ramirez, Citation2015; Ross and Cantrell, Citation2018). IFNγ is a common marker of cellular immunity, and higher levels of this cytokine have been associated with enhanced protective immune responses to pathogen infection (Lillehoj and Choi, Citation1998). The lower parts of the hen oviduct open to the cloaca, which might be colonised by numerous microorganisms via the vagina, and thus, these parts of the oviduct are more susceptible to infection by these microorganisms. At the time of laying, the reproductive tract, particularly the ovary, shows decreases in the lymphocyte number but increases in the number of macrophages. It has been proposed that this change helps prevent infection of the clean oviduct at the start of laying.
The expression of IL-2 was significantly lower in the additive-fed group than in the control group at ASF. The IL-1β and IL-2 expression levels of this group were also decreased at DF and continued to be observed at ASF; these findings suggest that yeast supplementation can modulate the immune response and maintain a balance between innate and protective immunity in chicken blood for at least 4 weeks (Wu et al. Citation2019). The observed decrease in IL-1β expression indicated that the number of macrophages might also be reduced and that the health status of laying hens was sufficiently good and suitable for the production of hygienic eggs. This response is likely related to the improved quality of the eggs, as demonstrated by the increases in the % albumen at DF and in the HU at ASF observed in the additive-fed group, which indicated that the hens were under low stress, exhibited a low infection rate and produced good-quality eggs. The 99.1% decrease in the expression of IL-1β from 3.921-fold (BF) to 0.0340-fold (DF) in the additive-fed group indicated that hens might exhibit some microbial invasion when fed only the basal diet, but supplementation with dried yeast reduced this invasion, and this decrease was maintained at 0.0023-fold (ASF) for at least 4 weeks, even though the hens were fed only the basal diet. These results might be a consequence of the low level of existing pathogens and the lower numbers of macrophages found in the additive-fed group compared with the control group. Jang et al. (Citation2013) reported that the upregulation of IL-1β mRNA levels correlated with induced clinical signs in chickens after infection with infectious bronchitis virus. With respect to the effect on the heat shock protein, the 89.4% decrease in HSP90 expression detected in the additive-fed group (from 2.23-fold (DF) to 0.237-fold (ASF)) indicated that the yeast additive could reduce the heat stress that might occur while raising chickens in semi-opened housing under an ambient temperature, which was highest during this period.
Conclusions
Supplementation of the basal diet with dried brewer’s yeast significantly improved egg production and quality and the immune response of productive laying hens. The effectiveness of dried yeast was observed mostly during the additive feeding period, but some parameters were also maintained after the experimental feeding was stopped for at least 4 weeks. Dried yeast additives could act independently as a functional prebiotic. Moreover, a combination of low-cost dried yeast and chicken gut-originated probiotics (B. subtilis and LAB) served as a potent synbiotic that promotes the growth and health of laying hens during the early-phase laying period at weeks 27–35. This combination should be further evaluated in aged laying hens with poor health. Using this approach, a prolonged laying hen performance could be achieved.
Ethical approval
All animal experiments were conducted in accordance with the guidelines and recommendations of the Institutional Animal Care and Use Committee (IACUC) and approved by the Animal Ethics Committee of Khon Kaen University (Approval no. ACUC-KKU 62/60).
Acknowledgements
The authors thank Professor Dr. Metha Wanapat for performing a critical review of this manuscript, Ms. Thongsa Buasuk for the excellent technical assistance provided, and Miss Lalita Thanwisai, Miss Soraya Tra-ngan, Miss Juthamas Buahom, Miss Sureeporn Wichiansri, and Mr. Sarun Thongnok for their help.
Disclosure statement
We certify that there are no conflicts of interest with any financial organisation regarding the materials discussed in the manuscript.
Data availability statement
The data that support the findings of this study are available on request from the corresponding author, (WS). The data are not publicly available due to (restrictions e.g. their containing information that could compromise the privacy of research participants).
Additional information
Funding
References
- Abdel-Aal EM, Akhtar H, Zaheer K, Ali R. 2013. Dietary sources of lutein and zeaxanthin carotenoids and their role in eye health. Nutrients. 5(4):1169–1185.
- Abdel-Mageed AM, Nii T, Isobe N, Yoshimura Y. 2017. Modulatory roles of proinflammatory cytokines on the expression of cathelicidins in the lower regions of the oviduct of laying hens. Cytokines. 99:66–72.
- Ahiwe EU, Abdallh ME, Chang’a EP, Omede AA, Al-Qahtani M, Gausi H, Graham H, Iji PA. 2020. Influence of dietary supplementation of autolyzed whole yeast and yeast cell wall products on broiler chickens. Asian-Australas Journal of Animal Sciences. 33(4):579–587.
- Al-Ajeeli MJ, Hubert SH, Leyva-Jimenez H, Hashim MM, Raghad A, Abdaljaleel RA, Alsadwi AM, Athrey G, Bailey CA. 2020. Impacts of dietary protein and prebiotic inclusion on liver and spleen gene expression in hy-line brown caged layers. Animals. 10(3):453..
- Arenas-Ramirez N, Woytschak J, Boyman O. 2015. Interleukin-2: biology, design and application. Trends in Immunology. 36(12):763–777..
- Attia YA, Al-Khalaifah H, Abd El-Hamid HS, Al-Harthi MA, El-Shafey AA. 2020. Growth performance, digestibility, intestinal morphology, carcass traits and meat quality of broilers fed marginal nutrients deficiency-diet supplemented with different levels of active yeast. Livestock Sciences. 233 (103945):1–10.
- Attia YA, Burke WH, Yamani KA. 1994. Response of broiler breeder hens to forced molting by hormonal and dietary manipulations. Poultry Sciences. 73(2):245–258. https://www.ncbi.nlm.nihgov/pubmed/8146072.
- Attia YA, Burke WH, Yamani KA, Jensen LS. 1995. Daily energy allotments and performance of broiler breeders. 2. Females. Poultry Sciences. 74(2):261–270.
- Attia YA, El-Din HS, El-Shafey AS. 2012. Effect of different concentrations of Saccharomyces cerevisiae (active yeast) on growth performance, carcass traits and immune response of broiler chicks fed standard- and low-density diet. Journal of Agriculture and Environmental Sciences. 11:230–251.
- Buahom J, Siripornadulsil S, Siripornadulsil W. 2018. Feeding with single strains versus mixed cultures of lactic acid bacteria and Bacillus subtilis KKU213 affects the bacterial community and growth performance of broiler chickens. Arabian Journal of Science and Engineering. 43(7):3417–3427. 1007/s13369-017-3045-6.
- Bunting K, Wang J, Shannon MF. 2006. Control of interleukin-2 gene transcription: a paradigm for inducible, tissue-specific gene expression. Vitamins and Hormones. 74:105–145.
- Burke WH, Attia YA. 1994. Molting single comb white leghorns with the use of the สLupron depot formulation of leuprolide acetate. Poultry Sciences. 73(8):1226–1232.
- Chen CY, Chen SW, Wang HT. 2016. Effect of supplementation of yeast with bacteriocin and Lactobacillus culture on growth performance, cecal fermentation, microbiota composition, and blood characteristics in broiler chickens. Asian-Australas Journal of Animal Sciences. 30(2):211–220..
- Chen JF, Kuang YH, Qu XY, Guo SC, Kang KL, He CQ. 2019. The effects and combinational effects of Bacillus subtilis and montmorillonite supplementation on performance, egg quality, oxidation status, and immune response in laying hens. Livestock Sciences. 227:114–119..
- FAO/WHO 2002. Guidelines for the evaluation of probiotics in food. Food and Agriculture Organization of the United Nations/World Health Organization, London, Ontario.
- Guo Y, Zhao Y-H, Pan Z-Y, An L-L, Balasubramanian B, Liu W-C. 2020. New insights into the role of dietary marine-derived polysaccharides on productive performance, egg quality, antioxidant capacity and jejunal morphology in late-phase laying hens. Poultry Sciences. 99(4):2100–2107..
- Hameed R, Tahir M, Khan SH, Ahmas T, Iqbal J. 2019. Effect of yeast supplementation on production parameters, egg quality characteristics and crude protein digestibility in hens. Advances in Lifesciences. 6(4):147–151.
- Hashim M, Fowler J, Haq A, Bailey CA. 2013. Effects of yeast cell wall on early production laying hen performance. Journal of Applied Poultry Research. 22(4):792–797..
- Hong HA, Duc LH, Cutting SM. 2005. The use of bacterial spore formers as probiotics. FEMS Microbiology Reviews. 29(4):813–835. https://doi.org/https://doi.org/10.1016/j.femsre.2004.12.001
- Jang H, Koo BS, Jeon EO, Lee HR, Lee SM, Mo IP. 2013. Altered pro-inflammatory cytokine mRNA levels in chickens infected with infectious bronchitis virus. Poult Sci. 92(9):2290–2298.
- Kabir SM. 2009. The role of probiotics in the poultry industry. International Journal of Molecular Sciences. 10(8):3531–3546..
- Khochamit N, Siripornadulsil S, Sukon P, Siripornadulsil W. 2015. Antibacterial activity and genotypic-phenotypic characteristics of bacteriocin-producing Bacillus subtilis KKU213: potential as a probiotic strain . Microbiological Research. 170:36–50..
- Khochamit N, Siripornadulsil S, Sukon P, Siripornadulsil W. 2020. Bacillus subtilis and lactic acid bacteria improve the growth performance and blood parameters and reduce Salmonella infection in broilers. Veterinary World. 13(12):2663–2672.
- Lei XJ, Ru YJ, Zhang HF. 2014. Effect of Bacillus amyloliquefaciens-based direct-fed microbials and antibiotic on performance, nutrient digestibility, cecal microflora, and intestinal morphology in broiler chickens. Journal of Applied Poultry Research. 23(3):486–493..
- Lee KW, Kim DK, Lillehoj HS, Jang SI, Lee SH. 2015. Immune modulation by Bacillus subtilis-based direct-fed microbials in commercial broiler chickens. Ani Feed Sci Tech. 200:76–85.
- Levkut M, Revajová V, Lauková A, Ševčíková Z, Spišáková V, Faixová Z, Levkutová M, Strompfová V, Pistl J, Levkut M. 2012. Leukocytic responses and intestinal mucin dynamics of broilers protected with Enterococcus faecium EF55 and challenged with Salmonella Enteritidis. Research on Veterinary Sciences. 93(1):195–201.
- Li QP, Gooneratne SR, Wang RL, Zhang R, An LL, Chen JJ, Pan W. 2016. Effect of different molecular weight of chitosans on performance and lipid metabolism in chicken. Animal Feed Sciences Technology. 211:174–180.
- Li L, Xu CL, Ji C, Ma Q, Hao K, Jin ZY, Li K. 2006. Effects of a dried Bacillus subtilis culture on egg quality. Poultry Sciences. 85(2):364–368..
- Lillehoj HS, Choi KD. 1998. Recombinant chicken interferon-γ-mediated inhibition of Eimeria tenella development in vitro and reduction of oocyst production and body weight loss following Eimeria acervulina challenge infection. Avian Diseases. 42(2):307–314.
- Liu X, Peng CY, Qu XY, Guo SC, Chen JF, He CQ, Zhou XB, Zhu SW. 2019. Effects of Bacillus subtilis C-3102 on production, hatching performance, egg quality, serum antioxidant capacity and immune response of laying breeders. Journal of Animal Physiology and Animal Nutrition. 103(1):182–190..
- Livak KJ, Schmittgen TD. 2001. Analysis of relative gene expression data using real-time quantitative PCR and the 2− ΔΔCT method. Methods. 25(4):402–408..
- Mata-Gómez LC, Montañez JC, Méndez-Zavala A, Aguilar CN. 2014. Biotechnological production of carotenoids by yeasts: an overview. Microbiological Cell Facts. 13:122014, http://www.microbialcellfactories.com/content/13/1/12.
- Mazanko MS, Gorlov IF, Prazdnova EV, Makarenko MS, Usatov AV, Bren AB, Chistyakov VA, Tutelyan AV, Komarova ZB, Mosolova NI, et al. 2018. Bacillus probiotic supplementations improve laying performance, egg quality, hatching of laying hens and sperm quality of roosters. Probiotics and Antimicrobial Proteins. 10(2):367–373.
- Ogbuewu IP, Okoro VM, Mbajiorgu EF, Mbajiorgu CA. 2019. Yeast (Saccharomyces cerevisiae) and its effect on production indices of livestock and poultry: a review. Comparative Clinical Pathology. 28(3):669–677..
- Osman M, Attia YA, Eglia E-SA, El-Deek AA. 1996. Effect of prozyme and yeast culture supplementations on performance of Pekin ducklings fed diets containing dried beet pulp. Egyptian Poultry Sciences Journal. 16 (III):573–559.
- Pineda-Quiroga C, Borda-Molina D, Chaves-Moreno D, Ruiz R, Atxaerandio R, Camarinha-Silva A, Aser García-Rodríguez A. 2019. Microbial and functional profile of the ceca from laying hens affected by feeding prebiotics, probiotics and synbiotics. Microorganisms. 7(5):123.
- Pourabedin M, Zhao X. 2015. Prebiotics and gut microbiota in chickens. FEMS Microbiological Letters. 362(15):fnv122.
- Ross SH, Cantrell DA. 2018. Signaling and function of interleukin-2 in T lymphocytes. Annual Reviews on Immunology. 36:411–433. 1146/annurev-immunol-042617-053352.
- Rimoldi S, Lasagna E, Sarti FM, Marelli SP, Cozzi MC, Bernardini G, Terova G. 2015. Expression profile of six stressrelated genes and productive performances of fast and slow growing broiler strains reared under heat stress conditions. Meta Gene. 6:17–25.
- Salajegheh MH, Yousef Elahi M, Salarmoini M, Baniasadi M. 2020. Efficacy of feeding various calcium source and concentration on egg quality, some blood variables and performance of aged laying hens. Poultry Science Journal. 8(1):33–42.
- Shivaramaiah S, Pumford NR, Morgan MJ, Wolfenden RE, Wolfenden AD, Torres-Rodríguez A, Hargis BM, Téllez G. 2011. Evaluation of Bacillus species as potential candidates for direct-fed microbials in commercial poultry. Poultry Sciences. 90(7):1574–1580..
- Swiatkiewicz S, Swiatkiewicz M, Arczewska-Wlosek A, Jozefiak D. 2015. Chitosan and its oligosaccharide derivatives (chito-oligosaccharides) as feed supplements in poultry and swine nutrition. Journal of Animal Physiology and Animal Nutrition). 99(1):1–12.
- Tellez G, Pixley C, Wolfenden RE, Layton SL, Hargis BM. 2012. Probiotics/direct fed microbials for Salmonella control in poultry. Food Research International. 45(2):628–633..
- Upadhaya SD, Rudeaux F, Kim IH. 2019. Efficacy of dietary Bacillus subtilis and Bacillus licheniformis supplementation continuously in pullet and lay period on egg production, excreta microflora, and egg quality of hyline-brown birds. Poultry Sciences. 98(10):4722–4728..
- Wang WC, Yan FF, Hu JY, Amen OA, Cheng HW. 2018. Supplementation of Bacillus subtilis-based probiotic reduces heat stress-related behaviors and inflammatory response in broiler chickens. Journal of Animal Sciences. 96(5):1654–1666.
- Wigley P, Kaiser P. 2003. Avian cytokines in health and disease. Revista Brasileira de Ciência Avícola. 5(1):1–14.
- Wu Y, Wang B, Zeng Z, Liu R, Tang L, Gong L, Li W. 2019. Effects of probiotics Lactobacillus plantarum 16 and Paenibacillus polymyxa 10 on intestinal barrier function, antioxidative capacity, apoptosis, immune response and biochemical parameters in broilers. Poultry Sciences. 98(10):5028–5039. https://doi.org/http://dx.doi.org/10.3382/ps/pez226.
- Xu Q, Azzam MMM, Zou X, Dong X. 2020. Effects of chitooligosaccharides supplementation on laying performance, egg quality, blood biochemistry, antioxidant capacity and immunity of laying hens during the late laying period. Italian Journal of Animal Sciences. 19(1):1181–1188.
- Yang Y, Iji PA, Kocher A, Mikkelsen LL, Choct M. 2008. Effects of dietary mannanoligosaccharides on growth performance, nutrient digestibility and gut development of broilers given different cereal based diets. Journal of Animal Physiology and Animal Nutrition. 92(6):650–659.
- Yalçın S, Şahin A, Duyum HM, Çalık A, Gümüş H. 2015. Effects of dietary ınactive yeast and live yeast on performance, egg quality traits, some blood parameters and antibody production to SRBC of laying hens. Kafkas Üniversitesi Veteriner Fakültesi Dergisi. 21:345–350.
- Yarru LP, Settivari RS, Gowda NKS, Antoniou E, Ledoux DR, Rottinghaus GE. 2009. Effects of turmeric (Curcuma longa) on the expression of hepatic genes associated with biotransformation, antioxidant, and immune systems in broiler chicks fed aflatoxin. Poultry Sci. 88(12):2620–2627.
- Zhang J-C, Chen P, Zhang C, Khalil MM, Zhang N-Y, Qi D-S, You-Wei W, Sun L-H. 2020. Yeast culture promotes the production of aged laying hens by improving intestinal digestive enzyme activities and the intestinal health status. Poultry Sciences. 99(4):2026–2032..
- Zokaeifar H, Balcázar JL, Saad CR, Kamarudin MS, Sijam K, Arshad A, Nejat N. 2012. Effects of Bacillus subtilis on the growth performance, digestive enzymes, immune gene expression and disease resistance of white shrimp, Litopenaeus vannamei. Fish Shellfish Immunology 33(4):683–689..