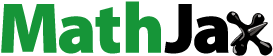
Abstract
An 8-week feeding experiment was designed to examine the influences of single or/and double strains of probiotic compared to antibiotic colistin on growth, hematological variables, blood serum metabolites and caecal fermentation in post-weaning rabbits exposed to heat stress conditions. A total of one hundred (35-day-old; average initial body weights 694 ± 7.33) New Zealand White rabbits were distributed into five groups. The experimental groups were the basal diet (control), or basal diet fortified with 120 mg colistin (COL); 5 × 106 cfu Clostridium butyricum (CB); 2 × 108 cfu Enterococcus faecium NCIMB 11181 (EF); or 2.5 × 106 cfu C. butyricum + 1 × 108 cfu E. faecium/kg diet (CB + EF). The results demonstrated that both the EF and CB + EF treatments enhanced (p < .05) final body weight and overall feed conversion ratio compared to control and COL treatment. The carcase yield percentage and relative organs weight were insignificantly affected in all treated groups. The COL treatment increased (p < .05) mean corpuscular volume and albumin/globulin ratio compared to the control group. Compared to the control, all probiotic and COL groups decreased (p < .05) serum total triglycerides and very low-density lipoprotein. The concentrations of Serum total protein, globulin, high-density lipoprotein, complement component 3, and lysozyme activity were higher (p < .05) in probiotic groups than those in the control group. Probiotic application improved the caecal total volatile fatty acids, propionate concentrations, and intestinal histomorphometry parameters. In conclusion, EF and CB + EF probiotic supplements could be used as alternatives to antibiotic colistin to enhance the growth and health of weaned rabbits under heat stress conditions.
Two strains of probiotics as alternatives to antibiotics were studied in rabbits under heat stress conditions.
Supplemental EF and CB + EF probiotic enhanced rabbit growth and feed utilisation.
Probiotic application improved biochemical and immunological indicators.
The application of the tested probiotics improved the caecal volatile fatty acids production and the intestinal histomorphometry parameters.
EF and CB + EF probiotic supplements could be used as an alternative to antibiotics to promote the growth of rabbits under heat stress conditions.
HIGHLIGHTS
Introduction
Numerous antibiotics, including chlortetracycline, tiamulin, bacitracin, monensin, and colistin, have been commonly used in European commercial rabbit farms in prophylactic dosages to act as growth promoters or to control pathogenic bacteria (Mateos et al. Citation2010; Attia et al. Citation2019). However, repeated use of antibiotics leads to increasing their residue in animal products together with antibiotic resistance in bacteria in both animal and human (Romero et al. Citation2012). Due to these restrictions, in addition to the few antibiotic numbers authorised by the European Union, there was an imperative need to search for viable alternatives to antibiotics in rabbit feeding. These alternatives should be able to promote rabbit growth and maintain the sanitary status of the digestive system (Attia, Hamed, Abd El-Hamid, Al-Harthi, et al. Citation2015; Attia, Hamed, Abd El-Hamid, Shahba, et al. Citation2015; Attia et al).
In tropical and subtropical regions, rabbits suffer from hypersensitivity to high ambient temperatures due to their dense fur and few sweat glands (Daader et al. Citation2018). Under hot climate, especially during summer, rabbits suffer from slow growth, loss of appetite, and poor feed utilisation which leads to increased mortality rates and serious economic losses (Hassan et al. Citation2021). Therefore, it is essential to enhance the heat stress tolerance of growing rabbits using practical approaches such as nutritional strategies. Probiotic products are a very appropriate choice as possible growth promoters in the rabbit industry as they positively boost the immune system, reduce pathogens, and improve intestinal barrier function (Attia et al. Citation2018). Additionally, the probiotic combats the problem of bacterial resistance resulted from antibiotic residues.
Enterococcus faecalis is a Gram-positive facultative anaerobic coccus, which belonging to lactic acid-producing bacterial species (Domann et al. Citation2007). Currently, the probiotic strain E. faecium 11181 (EF) was approved by the European Food Safety Authority (EFSA) as a feed additive to improve animal growth performance (EFSA Citation2012; Pajarillo et al. Citation2015). This strain has been reported to efficiently enhance feed utilisation, daily weight gain, and gut health by hindering the gut pathogens proliferation and promoting the growth of beneficial bacteria (Vrotniakienė and Jatkauskas Citation2013; Pajarillo et al. Citation2015).
Clostridium butyricum (CB), a Gram-positive anaerobe bacterium, produces butyrate in the gastrointestinal of healthy animals (Yang et al. Citation2012; Wang et al. Citation2019). Furthermore, it has stronger tolerance ability in high temperature environment, low pH, bile salt, compared with other probiotics. Consequently, CB has been recognised as effective and safe feed supplement (Kong et al. Citation2011). In an earlier experiment in broilers, CB has been demonstrated to boost the immune response, enhance growth performance indices, and modify the microflora structure (Yang et al. Citation2012). Furthermore, CB can increase the content of caecal volatile fatty acid (Han et al. Citation2018), maintain the intestinal flora balance, and stimulate the nutrients absorption (Mallo et al. Citation2010).
There are fewer studies deals with CB and EF probiotics in rabbits than in other monogastric farm species. Furthermore, knowledge on the effects of tested strains on the growth, intestinal morphology, and heat stress tolerance in post-weaning rabbits is limited. Based on the earlier reported beneficial impacts of both probiotic strains, we hypothesise that could be used as alternatives to antibiotic to stimulate the rabbit growth under summer conditions. Hence, the existing study was carried out to investigate the effects of EF and CB as a substitute for antibiotic colistin on growth, blood metabolites, immune function, intestinal morphology, and volatile fatty acids production in weaned rabbits reared under heat stress conditions.
Materials and methods
Animals, design, and management
This study was performed at the Rabbit Research Farm, Faculty of Agriculture, Zagazig University, Zagazig, Egypt during the hot climatic summer season.
The rabbits were reared under similar sanitary, management, and environmental conditions. All animals were acclimatised for one week before starting the experiment and fed on the control diet without additives until the start of the trial. The rabbits received the experimental diets formulated to cover their nutrient requirements according to De Blas and Mateos (Citation2010) recommendations. All animals were raised jointly in galvanised wire cages (Dimensions of each cage were 35 × 40 × 60 cm) in a naturally ventilated room. The cages had manual feeders and automatic nip drinkers to continuously supply clean fresh water. The experimental pelleted diet and water were offered ad libitum. All care and experimental procedures including test animals in the current study were conducted in line with the scientific and ethical regulations recommended by the European Parliament Directive (2010/63/EU).
For the experimental trial, a total of 100 (35-day-old; average initial body weights 694 ± 7.33 g) New Zealand White male rabbits were selected and randomly distributed into five groups. Each group contained ten replicates, with two rabbits per replicate (cage). The experimental groups were: (1) basal diet without probiotic and antibiotic supplement (control), (2) basal diet + colistin at 120 mg/kg diet (3) basal diet + CB at 5 × 106 cfu/kg diet, (4) basal diet + EF at 2 × 108 cfu/kg diet, or (5) basal diet + CB and EF complex at 2.5 × 106 cfu and 1 × 108 cfu/kg diet, respectively. The dose of probiotic supplements used in this experiment has followed the recommendation of the producing company. The colistin level was selected based on Romero et al. (Citation2012) recommendation. CB (Clostri-mix®) was obtained from Cheil Bio Company, LTD., Youngdungpo-Gu, Seoul, South Korea. EF is a commercial product (Protexin probiotic®) obtained from Probiotics International Ltd. Company, UK. The constituents and chemical analysis of the basal diet are shown in Table . The chemical composition of the basal diet was analysed according to AOAC (Citation2006) methods. Neutral detergent fibre and acid detergent fibre were estimated following the Van Soest et al. (Citation1991) method.
Table 1. Ingredients and composition of the basal diet.
Temperature-humidity index calculation
Throughout the experiment, relative humidity and ambient temperature were recorded by an automatic thermo-hygrometer (TFA Dostmann GmbH and Company. KG, Wertheim, Germany). Temperature-humidity index (THI) was used to assess the degree of exposure to natural thermal stress. The THI value was calculated based on Marai et al. (Citation2001) equation as follows: THI = Tem − [(0.31 − 0.31 × RH) (T − 14.4)], where RH refers to relative humidity percentage/100 and ambient temperature in Celsius. The values obtained are categorised as follows, THI <27.8 used as a thermal comfort zone, 27.8 to <28.9 indicates mild to moderate thermal stress, 28.9 to <30.0 indicates severe thermal stress, and >30.0 shows very severe thermal stress condition.
Performance measurements
The growth performance trial was continued for eight consecutive weeks (from 35 to 91-day of age). Feed intake was recorded for each cage by measuring the remaining quantities of diet then subtracting them from the supplied before giving the next quantities. All rabbits were weighed separately at the start of the feeding trial (35-d), 63, and 91 day of age. The relative growth rate (RGR) where W1 refers to the final weight, and W0 denotes the initial weight. Feed conversion ratio (FCR) was estimated as the ratio of feed intake (g) to body weight gain (g).
Carcase traits
At the feeding trial end, five rabbits per treatment were weighed and sacrificed to assess the carcase characteristics. When complete bleeding was reached, skin, and all entails were removed, and the carcase weight and some carcase organs were weighed (Blasco et al. Citation1993). The weight of the heart, liver, kidneys, spleen, and lungs were weighed and recorded as g/kg of pre-slaughter weight (SW). Carcase yield, as percentage, was measured by dividing the carcase weight on SW.
Blood sampling
At day-91 of age, five rabbits from each group were subjected to collections of blood sample. Two separate blood samples of each rabbit were collected from the lateral ear vein without harming the rabbits. The first sample (2 ml) was collected into an EDTA (Ethylenediaminetetraacetic acid) tube to estimate hematological evaluations. The second aliquot was used to separate the serum after centrifugation at 3000 rpm for 20 min. The collected sera was kept at −20 °C until measuring the biochemical and immunological parameters.
Hematological and biochemical parameters
In the aliquot contained EDTA (1 mg/ml), total erythrocytes (RBCs), total leukocytes (WBCs), and haemoglobin (Hb) were determined following the methods of Jain (Citation1986). Consequently, the mean cell haemoglobin [MCH = (Hb × 10)/RBCs], mean corpuscular volume [MCV = (PCV × 10)/RBCs], and mean corpuscular haemoglobin concentration [MCHC = (Hb × 10)/PCV] were calculated. The sera samples were used to estimate the total protein, albumin, total cholesterol (TC), triglyceride (TG), high-density lipoprotein (HDL), low-density lipoprotein (LDL), and very low-density lipoprotein (VLDL) contents spectrophotometrically by commercial kits (Diamond Diagnostic, Dokki, Giza, Egypt) according to the manufacturer’s guidelines. Serum globulin content of the tested animals in each group was computed by subtracting the albumin from total protein levels. Using commercial ELISA kits, serum concentrations of lysozyme activity and complement component 3 was determined following the manufacturer directives.
Determination of caecal volatile fatty acid profile
Samples from caecum contents were immediately collected from five rabbits per group after slaughtering. The caecal contents were squeezed into a beaker through four layers of cheesecloth. A caecal content of 1 ml was added to 200 μl meta-phosphoric acid 25% (w/v) in microcentrifuge tubes and centrifuged for 20 min at 30,000×g (15,000 rpm, JA–17 rotor) and. The supernatant was refrigerated at −20 °C for future analysis. The supernatant was removed to GC vials for VFA analysis according to Palmquist and Conrad (Citation1971).
Histopathological and histomorphometric measurements of the duodenum
At the end of the trial, rabbits were sacrificed, the abdomen was opened through a midline incision and the small intestine was gently removed. The segments of the duodenum (2 cm posterior to stomach) were collected from each rabbit and were first rinsed with saline (0.85% NaCl). Then cross-sectional lengths of 1 cm were cut from the duodenum. The collected tissue samples were fixed in 10% formalin solution and consequently dehydrated, cleared in xylene, embedded and blocked in paraffin by an automatic tissue processor. Then, four μm thickness sections were cut via a rotary microtome and lastly stained with Haematoxylin and Eosin (H&E) according to Suvarna et al. (Citation2013). Five sections per animal were inspected in a blinded manner by the light microscope (Olympus, Tokyo, Japan) at diverse magnifications to qualitatively and quantitatively detect histological changes resulted from different experimental materials.
From each animal, duodenal sections that represented the best view of villus and crypts were selected to be analysed using stereological image software, with its micrometric ruler. The following parameters were evaluated in five randomly-selected sections stained with H&E according to Seyyedin and Nazem (Citation2017) and Stamilla et al. (Citation2020). (1) Villous height (VH) was defined from their tip to the villus–crypt junction. (2) Villous width (VW) estimated by measuring the average of the base and tip of the villus. (3) Crypt depth (CD) was measured as the distance from top of crypt to the invagination between two villi. (4) Submucosal layer thickness (SMT). (5) Muscular layer thickness (MT). (6) Tunica serosa thickness (TST).
Statistical analysis
For the body weight and weight gain data, the experimental unit was the individual rabbit (20 rabbits per treatment), and, for the feed intake and feed conversion data, the experimental unit was the cage (10 cages per treatment). For the carcase traits and hemato-biochemical parameters, five rabbits per treatment were used. The SPSS.21® software was used to analyse the obtained data. (IBM Cooperation, Armonk, NY, USA). The data were statistically analysed via one-way ANOVA, according to the following model: Yij = μ + Di + eij, where μ= the overall mean, Di = dietary supplement effect, and eij = random error. Differences among means were analysed by Tukey's test. Probability values of < .05 were considered significant.
Results
Meteorological data
Throughout the experiment, the average daily relative humidity and the ambient temperature inside the farm were recorded between 50% and 68% (60.19% ± 1.20%) and 27.80 °C to 36.10 °C (31.78 °C ± 0.58 °C), respectively. Accordingly, the calculated THI values were ranged from 26.35 to 33.28. The overall mean of THI was 29.62 ± 0.49 throughout the experiment, which indicates that the rabbits were subjected to severe thermal stress following Marai et al. (Citation2001) equation.
Growth performance and carcase traits
Final body weight was higher (p = .042) and weight gain and RGR (d35–d91) tended to be greater (p = .054) in the groups (Table ). No significant differences were recorded on daily feed intake among groups during all experimental intervals. Dietary EF or EF + CB supplementation in rabbits diets had significantly (p = .028) enhanced FCR (35 and 91 d) compared with those fed the control diet (Table ). On the other hand, the carcase yield percentage and relative organs (heart, liver, kidneys, spleen, and lungs) weight were insignificantly affected in the treated groups (Table ).
Table 2. Effect of dietary supplemental probiotics and colistin as growth promoters on growth performance of broiler rabbit exposed to heat stress conditions.
Table 3. Effect of dietary supplemental probiotics and colistin as growth promoters on carcase traits (as % of pre-slaughter weight, SW) of broiler rabbit exposed to heat stress conditions.
Hemato-biochemical and immunological parameters
The data shown in Table indicates that dietary supplemental EF or EF + CB significantly (p = .011) increased blood haemoglobin levels as compared with control. Also, dietary administration with colistin led to a significant (p = .038) increase in the level of MCV compared to the non-supplemented group. Regarding the leukograms, there was no significant change in WBCs count, lymphocytes, monocytes, neutrophils, and eosinophils percentages among all experimental groups (Table ).
Table 4. Effect of dietary supplemental probiotics and colistin as growth promoters on hematological parameters of broiler rabbit exposed to heat stress conditions.
As shown in Table , the serum concentrations of total protein, globulin, and HDL were increased (p < .001) in the probiotic-supplemented groups. In contrast, lower total glycerides (p = .002) and VLDL (p = .003) values were obtained in rabbits groups fed on colistin or probiotics. Also, total cholesterol levels of EF and EF + CB groups were decreased (p = .039) compared with the control. Colistin-supplemented rabbits had a higher (p < .001) A/G ratio than the other groups. Lysozyme activity in the probiotic-supplemented groups was significantly (p = .004) higher than that in the non-supplemented group. The levels of serum C3 of EF and EF + CB groups were significantly increased (p = .005) compared with those of the control rabbits (Table ).
Table 5. Effect of dietary supplemental probiotics and colistin as growth promoters on protein and lipid profiles and immunological indices of broiler rabbit exposed to heat stress conditions.
Caecal fermentation
Both the EF and EF + CB treatments increased (p = .018) the caecal total VFA and butyrate concentration compared to the non-supplemented group (Table ). The propionate amount in all probiotic-supplemented groups was significantly (p < .001) increased compared with control group. In the same line, the EF + CB treatment resulted in the highest (p = .017) proportions of propionate (Table ).
Table 6. Effect of dietary supplemental probiotics and colistin as growth promoters on caecal volatile fatty acids (VFA) of broiler rabbit exposed to heat stress conditions.
Table 7. Effect of dietary supplemental probiotics and colistin on histomorphometric measurements in the intestine of broiler rabbit exposed to heat stress conditions.
Histological evaluation of tissues of duodenum
Histological examination of the duodenum of control heat stressed group showed marked injury (Figure ). The later represented through partial or complete desquamation of enterocytes from the tip of villi associated with villus flattening, thickening, and shortening as well as a fusion of adjacent villi. Focal necrosis and erosions with exposure of the lamina propria were detected accompanied with necrotic masses inside intestinal lumen. In addition, there was vacuolar degeneration of the epithelium. It was noticeable that mild epithelial cells hyperplasia and polyp formation in some villi were seen. Intraepithelial lymphocytosis was clearly visible. The crypt showed loss and/or hyperplasia and inflammation in some parts. There was predominant goblet cell loss in-between enterocytes in this group. Marked villous stroma broadening was accompanied with leukocytes infiltrations that included mononuclear cells as well as scattered neutrophils and sometimes extending to other layers. Submucosa appeared oedematous and congested and possessed atrophied intestinal glands. Muscular layer became thin in some parts of the examined tissues, in addition to, there was partial thick serosa. On the other side, treatment with colistin-treated rabbits showed decreased histological alterations than in control animals (Figure ). It was observed that the histological examinations of rabbits of probiotics (EF and CB) showed more improvement and more decrease in the morphological changes compared to heat stressed control group (Figure ). They appeared close to normal with some dysregulation in epithelial cell lining as well as little submucosal edoema and inflammatory cells. Conversely, the animals of group that treated with EF and CB together revealed that normal healthy and regular intestinal villi and crypts (Figure ).
Figure 1. Representative photomicrographs of duodenum of growing rabbit reared under summer conditions of different experimental groups. (A–C) control group fed non-supplemented diets, (D–F) colistin group, (G–I) Enterococcus faecium (EF) NCIMB 11181 group, (J–L) Clostridium butyricum (CB) group, (M–O) EF + CB group. Villi: V; Necrotic masses: N; Intestinal glands: G; Muscular layer: M; Serosa: red arrow; Crypt: C; Inflammatory cell infiltrations: I; Epithelium: E; Erosions: S; Stroma: ST; Intraepithelial lymphocytosis: L; Hyperplasia: HV; Congestion: black arrow; Goblet cell: green arrow (1st, 2nd, 3rd column of photos stained with H&E at 40×, 100×, and 400×, respectively).
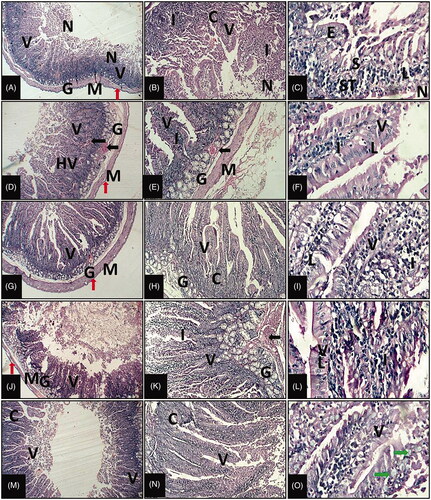
Histomorphometric measurements of the duodenum
Histomorphometric measurements were performed to histopathological alterations of the duodenum. As mentioned in , the villus height in the duodenum was increased in the treatment groups compared with heat stressed group. However, the villus width decreased in treated groups except control group. It was noticeable that the groups treated with probiotics and colistin showed increasing in Crypt depth compared with the heat stressed group. Also, the submucosal layer thickness was lesser in probiotics and colistin groups than control group. However, muscular layer thickness in the duodenum was greater compared to the control group. It should also be noted that a significant decreasing in tunica serosa thickness compared to heat stressed group ().
Discussion
Throughout the current experiment, based on the calculated THI value (29.62), growing rabbits were severely heat stressed during the feeding trial. Nevertheless, distinctive improvement in growth performance, blood constituents, and immune response were evident because of EF and/or CB dietary supplementation.
Initially, a growth-enhancing activity represented by a significant improvement in final body weight and FCR as well as a rising tendency of weight gain and RGR were apparent in growing rabbit fed diet supplemented with EF and EF + CB in the summer season. In weaning piglets, this EF strain has been displayed to efficiently augment daily weight gain and enhance feed conversion (Herzig et al. Citation2003; Vrotniakienė and Jatkauskas Citation2013) and broiler (Wu et al. Citation2019). In this study, the growth improvement in EF group could be probably owed to increases in the lactic acid bacteria count or improve the structure of intestinal mucosal thus augmenting the absorption of nutrients (Samli et al. Citation2007). Moreover, EF probiotic has also been shown to increases growth performances in monogastric animals like pigs via augmenting jejunal mucosa absorptive and secretory ability, enhancing intestinal barrier function, boosting disease resistance to pathogenic infection and preventing diarrhoea (Kreuzer-Redmer et al. Citation2016). On the other hand, the growing rabbit fed diet fortified with CB showed a tendency for enhanced growth but not significant. This could be linked to increased production of SCFAs in the caecum especially butyric acid that has high potency to enhance feed utilisation via balancing gut micro-ecological environments (Nakanishi et al. Citation2003).
In the existing study, it was evident that EF or EF + CB dietary supplementation considerably improved blood haemoglobin content in growing rabbits reared under thermal stress circumstances. In the intestinal tracts, lactic acid bacteria such as EF produces lactic acid that increases the bioavailability of iron, the main component of haemoglobin (Marciano et al. Citation2015; Vonderheid et al. Citation2019). Also, this type of probiotic has been reported to produce bacteriocins and enzymes that facilitate the food digestion, decrease the intestinal pH, and subsequently increase the minerals absorption (Huang et al. Citation2019).
In the present study, the probiotic-supplemented growing rabbits showed marked enhancement in lysozyme activity than that of the non-supplemented group. In addition, the serum C3 levels of EF and EF + CB groups were significantly increased with an improvement tendency in CB group; suggesting that the EF + CB high efficacy in enhancing non-specific immune function in rabbits. Generally, the probiotic strains ability to initiate specific immune responses has been owed to the ability of theses bacterial types to retain their mucus adhesion potency following inactivation (Ouwehand et al. Citation2000). Consequently, immune cells detect individual structural components of bacterial cells and elicit specific immune responses (Taverniti and Guglielmetti Citation2011). In poultry, Wu et al. (Citation2019) suggested that a main mechanism underlying the immunomodulatory effects of EF could be the modulation of the expression of proinflammatory and anti-inflammatory cytokines and other immune mediators. From another perspective, numerous researchers have demonstrated that the immune function and volatile fatty acids are closely related (Singh et al. Citation2014; Kelly et al. Citation2015). In this regard, in weaned piglets, Wang et al. (Citation2019) confirmed that CB and EF stimulate the VAFs production by controlling the intestinal flora structure, thus boosting the immune function. This link between increased VFA content and enhanced immune function is apparent in our study. Nevertheless, the mechanisms that underlie the immunomodulatory elicited by EF and/or CB in relation to rabbit health need to be more investigated.
Herein, the serum levels of total protein and globulin were increased in the EF and/or CB-supplemented groups. Such increment in serum total protein in the rabbit by the inclusion of probiotic in their diets indicated that these probiotic strain has affected protein metabolism (El-Shafei et al. Citation2019), which is harmonious with the enhancement observed in the body weights of treated groups. Additionally, the increased globulin content is a significant indicator of the enhanced immune function.
In the present study, the caecal total VFA content was significantly increased in EF and EF + CB treated growing rabbits with an increasing tendency in CB treated ones. Moreover, the propionate concentration was significantly in all probiotic-supplemented groups. Similarly, earlier studies confirmed the increased VFA amounts in the caecal digesta of broilers (Han et al. Citation2018) and pigs (Wang et al. Citation2019) fed diet supplemented with other strains of EF and CB. The incremented caecal VFA has been reported to be associated with several favourable effects including repair and regeneration of intestinal epithelial cells, reducing the pH of the intestine, and prohibiting the growth of harmful microorganisms (Pryde et al. Citation2002; Józefiak et al. Citation2008).
In the current experiment, EF and CB dietary supplementation to growing rabbits reared under summer conditions showed a notable hypocholesterolemic effect. Several mechanisms have been hypothesised for the probiotics associated hypocholestrmic activity including bile acids enzymatic deconjugation via probiotics bile-salt hydrolase (Lambert et al. Citation2008), cholesterol assimilation by probiotics (Pereira and Gibson Citation2002), cholesterol co-precipitation with deconjugated bile (Liong and Shah Citation2006), cholesterol attachment to probiotics cell walls (Liong and Shah Citation2005), cholesterol integration into the probiotics cell membranes throughout growth (Lye, Rahmat-Ali, et al. Citation2010), cholesterol conversion into coprostanol (Lye, Rusul, et al. Citation2010) and short-chain fatty acids production upon probiotics fermentation (De Preter et al. Citation2007).
In the current study, growing rabbits reared under thermal conditions and fed non-supplemented diets showed various pathological perturbations in their intestinal sections. Of note, the earlier group showed the smallest duodenal villus length and width but the larger crypt depth. On the other hand, the single or combined EF and CB addition to the rabbit diet significantly increased duodenal villus length and width but reduced crypt depth. The increased width and length of villus suggests that the intestinal absorption area is expanded, mature enterocytes are accumulated, and the absorption and digestion capacity is increased (Kim et al. Citation2012). Moreover, the diminishing crypt size refers to the recovery of intestinal villus by speeding up the regenerative enterocyte rate (Salim et al. Citation2013). Consequently, the gut can fight distressing damage by toxins or pathogens. Consistently, Wang et al. (Citation2019) indicated that dietary CB or EF improved the intestinal health development and absorption of nutrients in weaned piglets by reducing the crypt depth and incrementing the villus height. Also, in weaned piglets, EF enhanced the intestinal villi length (Galeano et al. Citation2016). Additionally, Chen et al. (Citation2018) reported that the duodenal, jejunal, and ileal villus height was increased in lipopolysaccharide-challenged weaned piglets fed CB supplemented diet. Meanwhile, EF increased the villus length in the jejunum of suckling piglets (Liu et al. Citation2017). The increased VFA content could be partly shared to the improvement of intestinal health as earlier evidence suggested that microbiota-derived butyrate is vital for the preservation of the integrity of intestinal epithelium (Singh et al. Citation2014; Kelly et al. Citation2015).
Based on the estimated hematological, biochemical, and histological analysis, we could propose that the growth enhancing activity of the tested proioibtics could be the outcome of the enhanced immune function, improved intestinal health, and augmented beneficial metabolites like caecal VFA. In this regard, several earlier studies confirmed the positive association of growth performance, the immune function, and the intestinal integrity in rabbits with diverse feed supplements (Alagawany et al. Citation2018; Al-Sagheer et al. Citation2019, Citation2020; Ayyat et al. Citation2021).
Of note, the combined EF and CB dietary supplementation resulted in a significant improvement of HDL, villus length, villus width, and intestinal muscle thickness rather than the single probiotic effect. Moreover, a maximal enhancement in other parameters including FBW, FCR, TG, HDL, VLDL, lysozyme activity, propionate, and crypth depth was evident in the EF + CB group. Such improvement denotes the favourable synergistic effect resulted from using these two probiotics. Similarly, Zhang et al. (Citation2012) demonstrated that addition of the Lactobacillus salivarius and Bacillus subtilis probiotics combination to the diet of layer hens had a greater effect on growth and immune function than single strains.
Conclusion
Our results showed that EF or/and CB supplementation resulted in a significant improvement in final body weight, feed conversion ratio, immune function, and blood biochemistry in post weaned rabbits as compared with the control and colistin treatment under thermal stress. Furthermore, the application of the tested probiotics improved the caecal total volatile fatty acids and propionate concentrations along with the intestinal histomorphometry parameters. The results suggested that supplementation of growing rabbits diets with 2 × 108 cfu EF or 2.5 × 106 cfu CB + 1 × 108 cfu EF/kg feed could be used as an alternative to antibiotics to promote the growth under heat stress conditions.
Ethical approval
All care and experimental procedures including test animals in the current study were conducted in line with the scientific and ethical regulations recommended by the European Parliament Directive (2010/63/EU).
Disclosure statement
No potential conflict of interest was reported by the author(s).
References
- Alagawany M, El-Hack A, Mohamed E, Al-Sagheer AA, Naiel MA, Saadeldin IM, Swelum AA. 2018. Dietary cold pressed watercress and coconut oil mixture enhances growth performance, intestinal microbiota, antioxidant status, and immunity of growing rabbits. Animals. 8(11):212.
- Al-Sagheer AA, Abdel-Rahman G, Ayyat MS, Gabr HA, Elsisi GF. 2020. Productive performance response of growing rabbits to dietary protein reduction and supplementation of pyridoxine, protease, and zinc. An Acad Bras Ciênc. 92(3):e20180989.
- Al-Sagheer AA, El-Hack A, Mohamed E, Alagawany M, Naiel MA, Mahgoub SA, Badr MM, Hussein EO, Alowaimer AN, Swelum AA. 2019. Paulownia leaves as a new feed resource: chemical composition and effects on growth, carcasses, digestibility, blood biochemistry, and intestinal bacterial populations of growing rabbits. Animals. 9(3):95.
- AOAC. 2006. Official methods of analysis. 18th ed. Washington (DC): AOAC.
- Attia YA, Bovera F, Abd‐Elhamid AE-HE, Calabrò S, Mandour MA, Al‐Harthi MA, Hassan SS. 2019. Evaluation of the carryover effect of antibiotic, bee pollen and propolis on growth performance, carcass traits and splenic and hepatic histology of growing rabbits. J Anim Physiol Anim Nutr. 103(3):947–958.
- Attia YA, Hamed RS, Abd El-Hamid AE, Shahba HA, Bovera F. 2015. Effect of inulin and mannan-oligosaccharides compared with zinc-bacitracin on growing performance, nutrient digestibility and hematological profiles of growing rabbits. Anim Prod Sci. 55(1):80–86.
- Attia YA, Hamed RS, Abd El-Hamid AE, Al-Harthi MA, Shahba HA, Bovera F. 2015. Performance, blood profile, carcass and meat traits and tissue morphology in growing rabbits fed mannanoligosaccharides and zinc-bacitracin continuously or intermittently. Anim Sci Pap Rep. 33:85–101.
- Attia YA, Hamid E AE, Ismaiel AM, de Oliveira MC, Al-Harthi MA, El-Naggar AS, Simon GA. 2018. Nitrate detoxification using antioxidants and probiotics in the water for rabbits. Rev Colomb Cienc Pecu. 31(2):130–138.
- Ayyat MS, Abd El-Latif KM, Helal AA, Al-Sagheer AA. 2021. Interaction of supplementary L-carnitine and dietary energy levels on feed utilization and blood constituents in New Zealand White rabbits reared under summer conditions. Trop Anim Health Prod. 53(2):1–8.
- Blasco A, Ouhayoun J, Masoero G. 1993. Harmonization of criteria and terminology in rabbit meat research. World Rabbit Sci. 1:3–10.
- Chen L, Li S, Zheng J, Li W, Jiang X, Zhao X, Li J, Che L, Lin Y, Xu S. 2018. Effects of dietary Clostridium butyricum supplementation on growth performance, intestinal development, and immune response of weaned piglets challenged with lipopolysaccharide. J Anim Sci Biotechnol. 9:1–14.
- Daader AH, Al Sagheer AA, Gabr HA, Abd El Moniem EA. 2018. Alleviation of heat-stress-related physiological perturbations in growing rabbits using natural antioxidants. Span J Agric Res. 16(3):e0610.
- De Blas C, Mateos GG. 2010. Feed formulation. In: De Blas C, Wiseman J, editors. Nutrition of the rabbit. London: CAB International; p. 222–232.
- De Preter V, Vanhoutte T, Huys G, Swings J, De Vuyst L, Rutgeerts P, Verbeke K. 2007. Effects of Lactobacillus casei Shirota, Bifidobacterium breve, and oligofructose-enriched inulin on colonic nitrogen-protein metabolism in healthy humans. Am J Physiol Gastrointest Liver Physiol. 292(1):G358–G368.
- Domann E, Hain T, Ghai R, Billion A, Kuenne C, Zimmermann K, Chakraborty T. 2007. Comparative genomic analysis for the presence of potential enterococcal virulence factors in the probiotic Enterococcus faecalis strain Symbioflor 1. Int J Med Microbiol. 297(7–8):533–539.
- EFSA. 2012. Guidance on the safety assessment of Enterococcus faecium in animal nutrition. EFSA J. 10:2682.
- El-Shafei AER, Younis T, Al-Gamal M, Hesham A. 2019. Impact of probiotic (Lactobacillus planterium) supplementation on productive and physiological performance of growing rabbits under Egyptian conditions. Egypt J Rabbit Sci. 29(1):125–148.
- Galeano JAC, Herrera AL, Suescún JP. 2016. The probiotic Enterococcus faecium modifies the intestinal morphometric parameters in weaning piglets. Revista Facultad Nacional de Agronomía Medellín. 69:7803–7811.
- Han J, Wang Y, Song D, Lu Z, Dong Z, Miao H, Wang W, He J, Li A. 2018. Effects of Clostridium butyricum and Lactobacillus plantarum on growth performance, immune function and volatile fatty acid level of caecal digesta in broilers. Food Agric Immunol. 29(1):797–807.
- Hassan F, Mobarez S, Mohamed M, Attia Y, Mekawy A, Mahrose K. 2021. Zinc and/or selenium enriched spirulina as antioxidants in growing rabbit diets to alleviate the deleterious impacts of heat stress during summer season. Animals. 11(3):756.
- Herzig I, Gőpfert E, Písaříková B, Strakova E. 2003. Testing of growth promoting and protective activity of the probiotic lactiferm in weaned piglets. Acta Vet Brno. 72(3):331–338.
- Huang L, Luo L, Zhang Y, Wang Z, Xia Z. 2019. Effects of the dietary probiotic, Enterococcus faecium NCIMB11181, on the intestinal barrier and system immune status in Escherichia coli O78-challenged broiler chickens. Prob Antimicro Prot. 11(3):946–956.
- Jain NC. 1986. Schalm's veterinary hematology. Philadelphia: Lea and Febiger.
- Józefiak D, Kaczmarek S, Rutkowski A. 2008. A note on the effects of selected prebiotics on the performance and ileal microbiota of broiler chickens. J Anim Feed Sci. 17(3):392–397.
- Kelly CJ, Zheng L, Campbell EL, Saeedi B, Scholz CC, Bayless AJ, Wilson KE, Glover LE, Kominsky DJ, Magnuson A, et al. 2015. Crosstalk between microbiota-derived short-chain fatty acids and intestinal epithelial HIF augments tissue barrier function. Cell Host Microbe. 17(5):662–671.
- Kim J, Ingale S, Kim Y, Kim K, Sen S, Ryu M, Lohakare J, Kwon I, Chae B. 2012. Effect of supplementation of multi‐microbe probiotic product on growth performance, apparent digestibility, cecal microbiota and small intestinal morphology of broilers. J Anim Physiol Anim Nutr. 96(4):618–626.
- Kong Q, He G-Q, Jia J-L, Zhu Q-L, Ruan H. 2011. Oral administration of Clostridium butyricum for modulating gastrointestinal microflora in mice. Curr Microbiol. 62(2):512–517.
- Kreuzer-Redmer S, Bekurtz JC, Arends D, Bortfeldt R, Kutz-Lohroff B, Sharbati S, Einspanier R, Brockmann GA. 2016. Feeding of Enterococcus faecium NCIMB 10415 leads to intestinal miRNA-423-5p-induced regulation of immune-relevant genes. Appl Environ Microbiol. 82(8):2263–2269.
- Lambert JM, Bongers RS, de Vos WM, Kleerebezem M. 2008. Functional analysis of four bile salt hydrolase and penicillin acylase family members in Lactobacillus plantarum WCFS1. Appl Environ Microbiol. 74(15):4719–4726.
- Liong M, Shah N. 2005. Acid and bile tolerance and cholesterol removal ability of lactobacilli strains. J Dairy Sci. 88(1):55–66.
- Liong MT, Shah NP. 2006. Effects of a Lactobacillus casei synbiotic on serum lipoprotein, intestinal microflora, and organic acids in rats. J Dairy Sci. 89(5):1390–1399.
- Liu C, Zhu Q, Chang J, Yin Q, Song A, Li Z, Wang E, Lu F. 2017. Effects of Lactobacillus casei and Enterococcus faecalis on growth performance, immune function and gut microbiota of suckling piglets. Arch Anim Nutr. 71(2):120–133.
- Lye H-S, Rahmat-Ali GR, Liong M-T. 2010. Mechanisms of cholesterol removal by lactobacilli under conditions that mimic the human gastrointestinal tract. Int Dairy J. 20(3):169–175.
- Lye H-S, Rusul G, Liong M-T. 2010. Removal of cholesterol by lactobacilli via incorporation and conversion to coprostanol. J Dairy Sci. 93(4):1383–1392.
- Mallo J, Riopérez J, Honrubia P. 2010. The addition of Enterococcus faecium to diet improves piglet's intestinal microbiota and performance. Livestock Science. 133(1-3):176–178.
- Marai IFM, Ayyat MS, Abd El-Monem UM. 2001. Growth performance and reproductive traits at first parity of New Zealand white female rabbits as affected by heat stress and its alleviation under Egyptian conditions. Trop Anim Health Prod. 33(6):451–462.
- Marciano R, Santamarina AB, de Santana AA, Silva M. d L C, Amancio OMS, do Nascimento C. M d P O, Oyama LM, de Morais MB. 2015. Effects of prebiotic supplementation on the expression of proteins regulating iron absorption in anaemic growing rats. Br J Nutr. 113(6):901–908.
- Mateos G, Rebollar P, De Blas C. 2010. Minerals, vitamins and additives. In: de Blas C, Wiseman J, editors. Nutrition of the rabbit. Wallingford: CABI Publishing; p. 119–150.
- Nakanishi S, Kataoka K, Kuwahara T, Ohnishi Y. 2003. Effects of high amylose maize starch and Clostridium butyricum on metabolism in colonic microbiota and formation of azoxymethane-induced aberrant crypt foci in the rat colon. Microbiol Immunol. 47(12):951–958.
- Ouwehand A, Tölkkö S, Kulmala J, Salminen S, Salminen E. 2000. Adhesion of inactivated probiotic strains to intestinal mucus. Lett Appl Microbiol. 31(1):82–86.
- Pajarillo EAB, Chae JP, Balolong MP, Kim HB, Park C-S, Kang D-K. 2015. Effects of probiotic Enterococcus faecium NCIMB 11181 administration on swine fecal microbiota diversity and composition using barcoded pyrosequencing. Anim Feed Sci Technol. 201:80–88.
- Palmquist D, Conrad H. 1971. Origin of plasma fatty acids in lactating cows fed high grain or high fat diets. J Dairy Sci. 54(7):1025–1033.
- Pereira DI, Gibson GR. 2002. Cholesterol assimilation by lactic acid bacteria and bifidobacteria isolated from the human gut. Appl Environ Microbiol. 68(9):4689–4693.
- Pryde SE, Duncan SH, Hold GL, Stewart CS, Flint HJ. 2002. The microbiology of butyrate formation in the human colon. FEMS Microbiol Lett. 217(2):133–139.
- Romero C, Rebollar PG, Moscati L, Dal Bosco A, Castellini C, Cardinali R. 2012. Effect of substitution of medium-chain organic acids for zinc bacitracin in a diet containing colistin on performance and development of intestinal lymphoid tissues in growing rabbits experimentally infected with Escherichia coli O103 and Clostridium perfringens toxinotype A. Anim Feed Sci Technol. 174(3–4):174–181.
- Salim HM, Kang HK, Akter N, Kim DW, Kim JH, Kim MJ, Na JC, Jong HB, Choi HC, Suh OS, et al. 2013. Supplementation of direct-fed microbials as an alternative to antibiotic on growth performance, immune response, cecal microbial population, and ileal morphology of broiler chickens. Poultr Sci. 92(8):2084–2090.,
- Samli HE, Senkoylu N, Koc F, Kanter M, Agma A. 2007. Effects of Enterococcus faecium and dried whey on broiler performance, gut histomorphology and intestinal microbiota. Arch Anim Nutr. 61(1):42–49.
- Seyyedin S, Nazem MN. 2017. Histomorphometric study of the effect of methionine on small intestine parameters in rat: an applied histologic study. Folia Morphol. 76(4):620–629.
- Singh N, Gurav A, Sivaprakasam S, Brady E, Padia R, Shi H, Thangaraju M, Prasad PD, Manicassamy S, Munn DH, et al. 2014. Activation of Gpr109a, receptor for niacin and the commensal metabolite butyrate, suppresses colonic inflammation and carcinogenesis. Immunity. 40(1):128–139.,
- Stamilla A, Messina A, Sallemi S, Condorelli L, Antoci F, Puleio R, Loria GR, Cascone G, Lanza M. 2020. Effects of microencapsulated blends of organics acids (OA) and essential oils (EO) as a feed additive for broiler chicken. A focus on growth performance, gut morphology and microbiology. Animals. 10(3):442.
- Suvarna KS, Layton C, Bancroft JD. 2013. Bancroft's theory and practice of histological techniques. 7th ed. Oxford: Churchill Livingstone.
- Taverniti V, Guglielmetti S. 2011. The immunomodulatory properties of probiotic microorganisms beyond their viability (ghost probiotics: proposal of paraprobiotic concept). Genes Nutr. 6(3):261–274.
- Van Soest P V, Robertson J, Lewis B, 1991. Methods for dietary fiber, neutral detergent fiber, and nonstarch polysaccharides in relation to animal nutrition. J Dairy Sci 74:3583–3597.
- Vonderheid SC, Tussing-Humphreys L, Park C, Pauls H, OjiNjideka Hemphill N, LaBomascus B, McLeod A, Koenig MD. 2019. A systematic review and meta-analysis on the effects of probiotic species on iron absorption and iron status. Nutrients. 11(12):2938.
- Vrotniakienė V, Jatkauskas J. 2013. Effects of probiotics dietary supplementation on diarrhea incidence, fecal shedding of Escherichia coli and growth performance in post-weaned piglets. Veterinarija Ir Zootechnika. 63:81–88.
- Wang K, Cao G, Zhang H, Li Q, Yang C. 2019. Effects of Clostridium butyricum and Enterococcus faecalis on growth performance, immune function, intestinal morphology, volatile fatty acids, and intestinal flora in a piglet model. Food Funct. 10(12):7844–7854.
- Wu Y, Zhen W, Geng Y, Wang Z, Guo Y. 2019. Effects of dietary Enterococcus faecium NCIMB 11181 supplementation on growth performance and cellular and humoral immune responses in broiler chickens. Poultr Sci. 98(1):150–163.
- Yang C, Cao G, Ferket P, Liu T, Zhou L, Zhang L, Xiao Y, Chen A. 2012. Effects of probiotic, Clostridium butyricum, on growth performance, immune function, and cecal microflora in broiler chickens. Poultr Sci. 91(9):2121–2129.
- Zhang J, Xie Q, Ji J, Yang W, Wu Y, Li C, Ma J, Bi Y. 2012. Different combinations of probiotics improve the production performance, egg quality, and immune response of layer hens. Poultr Sci. 91(11):2755–2760.