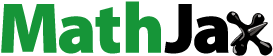
Abstract
Ozone is generally used for preservation and disinfection in many different areas, especially in the food, chemical industry, and health sector due to decompose into oxygen and leave no residue. In this study, the impacts of ozone application on the microbiological of commercially produced broiler feeds were investigated. For this purpose, Aspergillus niger NRRL 326 was introduced into the starter, grower, and finisher diets. Then the effects of gaseous ozone application at different doses (0.9 and 5.6 g h−1) and durations (15 and 30 min) were investigated. A. niger and total mould-yeast numbers were decreased in the feed samples as a result of the ozone application and statistically, a significant difference was found (p < .05). In this study, ozone dose, duration, and their interactions were found to be effective on the decrease of mould-yeast counts in all treated broiler feeds. However, the effect of ozone was higher on pelleted form feeds (grower and finisher). It might be concluded that the disinfection properties of ozone can change according to the physical and chemical structure of feed material and the surface area which contact with ozone.
Ozone application can result in a moderate reduction in A. niger and total mould-yeast counts
The most reduction on microbial load was observed in the feed applicated with 5.6 g h−1 gaseous ozone for 30 min.
Ozone treatment might be useful to preserve and extend the shelf-life of broiler feeds
Ozone efficiency also depends on the contact surface of the application area apart from the treatment time and dose.
Highlights
Introduction
In the last decades, the growing concerns of consumers about safe, healthy, and high-quality food have led producers to produce higher quality food products of animal origin for consumers. Management conditions, meeting nutrient requirements of animals, and feed quality are important to obtain healthy and high-quality products from farm animals. One of the factors affecting food and feed quality is feed hygiene. The topic is covered by Regulation (EC) No 183/2005 of the European Parliament and of the Council of 12 January 2005 laying down requirements for feed hygiene. Feed hygiene is defined as ‘the measures and conditions necessary to control hazards and to ensure fitness for animal consumption of a feed’ in the Regulation (European Commission, Citation2005).
Feed hygiene is threatened by fungal activity which is the main source of mycotoxin contamination. Food and Agriculture Organisation (FAO) acknowledged that contamination of food-crop with mycotoxin was anticipated as 25% before 1985. However, a recent review stated that this value is greatly underestimated, the actual value can be as high as 60–80% (Eskola et al. Citation2020). Fungal toxins are secondary metabolites produced by some fungi including Aspergillus, Fusarium, and Penicillium. Fungal contamination may occur at any step of food and feed chains (Kovalsky et al. Citation2016). Various physical, chemical, and biological methods are used to protect feed against microbial activities and mycotoxin contamination (Ricke et al. Citation2019; Conte et al. Citation2020).
Ozone, which is naturally present in the stratosphere, is formed by the UV rays of the sun (Ding et al. Citation2019). It is a colourless gas at room temperature with a characteristic odour and containing three oxygen atoms in its structure (Pandiselvam et al. Citation2019). Ozone is one of the most effective antimicrobial and deodorising agents known. Ozone can be applied in gas and liquid form. Advantages of ozone over other disinfectants are its rapid disintegration into oxygen after application and leaving no residues. Due to its high oxidation power, it is used in many different fields, such as storing fruit, vegetables; preventing microbial activities and pests; extending the shelf life of foods; in the treatment of industrial wastes, grains, and feeds; disinfection of drinking water; air sterilisation of chicken meat, and slaughterhouse equipment (Remondino and Valdenassi Citation2018; Ding et al. Citation2019).
This study is planned to reveal the impacts of ozone application in feed production so the facilities may integrate this treatment into their process flow to maintain feed hygiene. With the help of ozone application, possible risks and losses during production might be prevented. This study aimed to investigate the effects of ozone application on microbiology and the fatty acid profile of broiler feeds.
Materials and methods
Broiler feeds based on corn, and soybean meals were purchased from a commercial company and used as research material. These diets were prepared to meet the nutrient requirements of broiler chickens in three different growth periods (such as starter, grower, and finisher). The starter diet was in mash form which is suitable for chicks’ consumption. However, grower and finisher diets were used in pellet form which was commonly used in the broiler sector. Two different doses (0.9 and 5.6 g h−1) and two different periods (15 and 30 min) of ozone were applied to the feeds at room temperature (22 °C; 46% Relative Humidity).
Nutrition analysis
The physicochemical analyses, including dry matter (%), crude protein (%), ash (%), ether extract (%), and crude fibre (%) were performed according to the AOAC Methods (AOAC Citation1990).
Preparation of mould suspension
The mould (Aspergillus niger NRRL 326) strain used in the study was cultured in ichloran-rose bengal chloramphenicol (DRBC) medium and allowed to incubate at 30 °C for 7 days. At the end of the incubation, 10 mL of 0.01% (v/v) Tween 80 (Merck, Germany) sterile serum was added to the cultures and mixed for 1 min. After mixing, mould suspension was poured into two spray bottles containing 240 mL of sterile serum to obtain inoculation suspensions.
Spraying process
A spraying process was applied to ensure the homogenous and reproducible mould contamination on the feeds. Before ozone application, the samples were contaminated with Aspergillus niger NRRL 326. The contamination process was performed according to the modified method of Guner (Citation2014).
Ozone application
The mould-contaminated feed was treated with two different doses (0.9 and 5.6 g h−1) which are the lowest and highest doses of the ozone generator used in this study, and two different periods (15 and 30 min) of ozone at room temperature. The application was made by PCS Ozonmatic device (Maltepe, Istanbul).
Microbial analysis
DRBC (Merck 1.00466) agar was used for counting total yeast and mould before and after ozone treatment. Cultured agars were incubated at 25 °C for 5 days and then the count of moulds and yeasts per gram of samples were calculated at the end of the incubation period (Tournas et al. Citation2017). Mould-yeast analysis of starting feed materials (as starter, grower, finisher) before mould contamination were found 4.08, 4.08, and 3.77 log cfu g−1, respectively.
Fatty acid analysis
Fatty acid profile was evaluated to determine the effect of ozone exposure on fat components of feed samples. Lipid extraction from the feed was carried out by hexane extraction under the operating conditions specified in Paquot (Citation2013) IUPAC methods no. 1.121. The total fat content of the samples is expressed as a percentage by the mass of the product.
Fatty acid methyl esters are formed by trans-esterification with methanolic potassium hydroxide (ISO-5509:2000). The fatty acid methyl ester was injected in gas chromatography [with a capillary column, TR-CN100 (0.25 mm × 100 m × 0.2 mm)] and analysed by gas chromatography (Shimadzu 2010, Japan) equipped with a flame-ionisation detector. The carrier gas was helium, with a flow rate of 0.5 mL/min. The temperatures of the injector and the detector were held at 250 and 280 °C, respectively. The initial oven temperature of 120 °C was maintained for 10 min, raised to 240 °C at a rate of 5 °C/min. The injection volume was 1 μL. The peaks were identified by comparing the retention times and area percentages with those of authentic standards of FAMEs obtained from Nu-Chek-Prep Inc. (Paquot Citation2013).
Statistical analysis
The study was planned by following the 2 × 2 factorial design with two periods of time (15 and 30 min) and two levels of ozone application dose (0.9 g h−1; 5.6 g h−1) with three replicates for each treatment. The obtained data were analysed according to Duncan Multiple Comparison Test if the F test was significant (SPSS Citation2018). In addition, paired t-test was used to compare the results before and after ozone application. The applied mathematical model was as follows (EquationEquation (1)(1)
(1) ):
(1)
(1)
Yijk: observation applying the ith application time with jth ozone dose
µ: overall average
Ai: effect of ith ozone application time
Bj: effect of jth ozone application dose
(AB)ij: interaction effect of ith application time x jth ozone dose
eijk: error associated with each observation
Results and discussion
Wendee analysis results of feeds
Nutrient compositions of broiler starter, grower, and finisher diets were given in Table . The data were obtained before and after the ozone application process. While protein contents of starter diets were higher, ether extract levels were found lower, as expected. This is because the energy requirements of broilers are higher during the grower and finisher periods (Leeson and Summers Citation2005).
Table 1. Nutrient composition of feeds (starter, grower, and finisher).
Fatty acid profiles of ozone-treated feed samples
For quality assessment, fatty acid oxidation was evaluated. Lipid oxidation may occur when ozone oxidises the unsaturated lipids of the feed samples. The fatty acid profiles of the starter feed are shown in Table . As the ozone application dose increased, a decrease was observed in unsaturated fatty acids with high carbon numbers, indicating that lipid was affected by ozone under the experimental conditions.
Table 2. Fatty acid profiles of starter feed for broilers.
Table shows the fatty acid profiles of the grower feed. Numerically a reduction was observed in C18:2n6c fatty acid with double bond due to high ozone dose. In addition, Table shows the fatty acid profiles of the finisher feed, and C:18:3n6 fatty acid was found in the control group but not in the ozone-treated groups.
Table 3. Fatty acid profiles of grower feed for broilers.
Table 4. Fatty acid profiles of finisher feed for broilers.
Sandhu et al. (Citation2011) reported that ozone oxidises lipids in wheat flour. Also, Kells et al. (Citation2001) found similar results in their study and found that fatty acids were oxidised by ozone. Byun et al. (Citation1997) stated that exposure of aloe powder, bee pollen, and red ginseng powder to high ozone concentrations (about 18.0 mg L−1) for 8 h caused significant changes in fatty acid composition because of the destruction of double bonds in the fatty acids. However, Naito (Citation1989) reported that the oxidation of lipids in cereal grains was rarely observed after 0.05–5 ppm ozone treatment for 1 h. In contrast to these findings, Dubois et al. (Citation2006) reported in their study that the lipid content in the wheat kernel subjected to ozone gas treatment was not affected. Additionally, Mendez et al. (Citation2003) also indicated that high (50 ppm for 30 days) ozone dosing did not alter the fatty acid composition of grains, such as soybean, wheat, and corn. Although, they were pointed that the contact surface area might change the effectiveness of ozone application.
The difference between our findings may be attributed to the matrix effect of particle size. Starter feed was mash and so porous however the grower and finisher feed was in pellet form and the ozone might not penetrate effectively in it. These findings draw attention to that ozone treatment depends on the surface area of the samples.
Effects of ozone application on microbial quality of feeds
Feed samples contaminated with A. niger were treated with different ozone concentrations (0.9 and 5.6 g h−1) for 15 and 30 min, and fungi growth was recorded for each feed type individually, to evaluate the effectiveness of ozone on inhibition of the moulds.
The effect of ozone application on starter feed at different doses and times on A. niger and mould counts was demonstrated (Table ). Also, Box and Whisker plots of the results were shown in . The biggest inhibition rate in A. niger and mould-yeast numbers were observed in starter feeds where ozone was applied at 5.6 g (per hour) for 30 min (p < .01), and resulted in a 23.33% decrease in A. niger numbers and a 26.23% decrease in total mould-yeast numbers. This result approves that as the dose and duration of ozone increases, its effectiveness also increases. It was proved that ozone exposure with longer time and higher dose leads to more effective microbial inactivation (Brodowska et al. Citation2018). Furthermore, Freitas-Silva and Venâncio (Citation2010) stated that ozone causes fungal inactivation by damaging the membrane integrity. As a result of ozone application, A. niger and total mould-yeast counts also decreased in the grower feed samples (Table ; ). Similar to the starter feed, high dose, and long duration were found to be effective in grower feed (p < .05). While A. niger was found to be completely inhibited (p < .001), total mould-yeast counts were reduced by 77.84%.
Table 5. Effects of ozone application on A. niger, and total mould and yeast counts of starter feed.
Table 6. Effects of ozone application on A. niger, and total mould and yeast counts of grower feed.
As shown in Table , the A. niger (p < .05) and mould-yeast (p < .001) counts were inhibited by the 5.6 g h−1 and 30 min ozone application to the finisher feed. In addition, Box and Whisker plots of the results were shown in . These results are consistent with the study conducted by Torlak et al. (Citation2016). They also reported that AFB1 levels and mould-yeast spores were largely neutralised by exposure to gaseous ozone (2.8 and 5.3 mg L−1 at room temperature) on feed samples (Torlak et al. Citation2016). In another study, Luo et al. (Citation2014) noted that ozone at a concentration of 90 mg L−1 had a clear effect on decreasing both mould count and aflatoxin level of corn.
Zotti et al. (Citation2008), found that ozone has a completely inhibitory effect on the growth of A. niger. In different Aspergillus strains, the development of mould spores abated. In addition, some researchers reported that A. fumigatus, and A. niger mutants are more susceptible to inactivate by disinfecting agents (Lukiewicz et al. Citation1980; Jahn et al. Citation1997).
Table 6. Effects of ozone application on A. niger, and total mould and yeast counts of finisher feed.
El-Desouky et al. (Citation2012), in their study on Aspergillus strains in wheat grains, have demonstrated the effects of ozone application in different periods and doses. In this study, it was observed that 40 ppm and 20 min ozone treatment inactivated Aspergillus moulds in wheat grains and it was reported to be the ideal ratio for the destruction of aflatoxin.
Ochratoxin A is an important mycotoxin produced by Aspergillus and Penicillium species commonly found in cereals. Qi et al. (Citation2016) observed that ozone application significantly reduced the content of Ochratoxin A in maize. Oztekin et al. (Citation2006) applied 5 and 10 ppm doses of ozone gas on dried figs. As a result, total aerobic mesophyll, yeast/mould counts decreased by respectively 38 and 72%, and all coliform bacteria were inhibited.
In the study, ozone in both mash and pellet feed form was found to be effective on A. niger and mould-yeast numbers. However, the effect of ozone on grower and finisher feeds (in pellet form) was observed to be higher. It can be said that the physical, chemical structure, and ingredient of the feed material and the surface area in which it interacts are effective in the oxidation ability of ozone. Supporting our results, Proctor et al. (Citation2004) also demonstrated that higher aflatoxin degradation was detected on peanut kernels, compared to the flour. They pointed that the greater exposure area, and easy ozonation of kernel surfaces might be reasons for this effect.
To be considered microbiologically clean of feed and raw materials, the number of fungi per gram should not exceed 3 log and the number of bacteria should not exceed 4 log. However, microorganisms can develop and multiply very quickly under appropriate conditions and increase their number by 10 times (Eagon Citation1962). An increase in microorganism loads may be observed depending on the storage conditions, and duration of the feeds. The studies presented thus far provide evidence that ozone might be an eligible disinfectant to keep feed samples uncontaminated.
Conclusions
In this study, the effects of gaseous ozone application on microbiological properties of broiler feeds were investigated. Ozone dose and duration were found to be effective on inactivation of A. niger and mould-yeast in all feeds. This study showed that ozone preserves feeds and improves microbial quality. Due to the high oxidation capacity of ozone, it is very useful to investigate nutrient losses in broiler feeds to further eliminate microbial contamination in feeds. Also, the determination of parameters, such as high oxidation capacity, thiobarbituric acid analysis, fat-free acidity, and peroxide value, which are the criteria of lipid quality, would be more useful in understanding the effect of ozone. Furthermore, in vivo experiments are needed to understand the effects of ozone-treated feed on performance, internal organs, gut microbiota, histology, and meat quality in broilers.
Ethical approval
This study follows the principles of the declaration of Helsinki.
Geological information
The geological location where the study was conducted is 40.991879°N, 27.581678°E.
Acknowledgements
The manuscript hereby presented is a part of the MSc thesis of Orcun CELIK. The authors are grateful for the technical support of the Agricultural Faculty, Tekirdag Namik Kemal University, Turkey. This research did not receive any specific funding. Also, this study was achieved with the support of PCS Electronic in İstanbul (Turkey), in particular Mehmet EFE who is the ozone master.
Disclosure statement
The authors declare no potential conflict of interest.
Data availability statement
The data that support the findings of this study are available from the corresponding author, [AAO], upon reasonable request.
Figure 1. Box and Whisker plots of starter feed’ microbial quality; (a) A. niger, and (b) Mould-yeast counts.
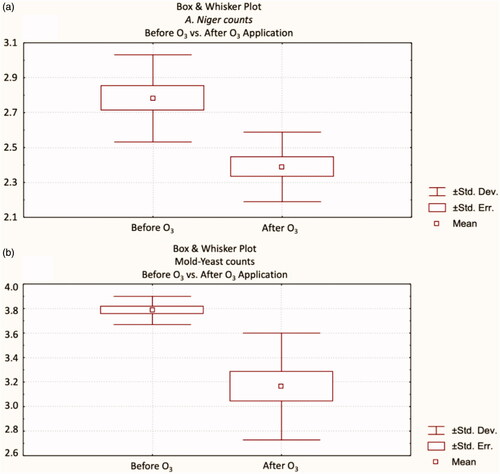
References
- AOAC. 1990. Official methods of analysis. 15th ed. Association of Official Analytical Chemists Inc. Arlington, VA, p.770–771.
- Brodowska AJ, Nowak A, Śmigielski K. 2018. Ozone in the food industry: principles of ozone treatment, mechanisms of action, and applications: an overview. Crit Rev Food Sci Nutr. 58(13):2176–2201.
- Byun MW, Yook HS, Kwon OJ. 1997. Comparative effects of gamma irradiation and ozone treatment on hygienic quality of aloe powders. Int J Food Sci Technol. 32(3):221–227.
- Conte G, Fontanelli M, Galli F, Cotrozzi L, Pagni L, Pellegrini E. 2020. Mycotoxins in feed and food and the role of ozone in their detoxification and degradation: an update. Toxins. 12(8):486.
- Ding W, Jin W, Cao S, Zhou X, Wang C, Jiang Q, Huang H, Tu R, Han SF, Wang Q. 2019. Ozone disinfection of chlorine-resistant bacteria in drinking water. Water Res. 160:339–349.
- Dubois M, Coste C, Despres AG, Efstathiou T, Nio C, Dumont E, Parent-Massin D. 2006. Safety of Oxygreen, an ozone treatment on wheat grains. Part 2. Is there a substantial equivalence between Oxygreen-treated wheat grains and untreated wheat grains? Food Addit Contam. 23(1):1–15.
- Eagon RG. 1962. Pseudomonas natriegens, a marine bacterium with a generation time of less than 10 minutes. J Bacteriol. 83(4):736–737.
- El-Desouky TA, Sharoba AMA, El-Desouky AI, El-Mansy HA, Naguib K. 2012. Effect of ozone gas on degradation of aflatoxin B1 and Aspergillus flavus fungal. J Environ Anal Toxicol. 2(2):1–6.
- Eskola M, Kos G, Elliott CT, Hajšlová J, Mayar S, Krska R. 2020. Worldwide contamination of food-crops with mycotoxins: validity of the widely cited ‘FAO estimate’ of 25%. Crit Rev Food Sci Nutr. 60(16):2773–2789.
- European Commission. 2005. Regulation (EC) No 183/2005 of the European Parliament and of the Council of 12 January 2005 Laying Down Requirements for Feed Hygiene; [accessed 2021 April 15]. https://eur-lex.europa.eu/legal-content/EN/TXT/PDF/?uri=CELEX:02005R0183-20160423&from=HR
- Freitas-Silva O, Venâncio A. 2010. Ozone applications to prevent and degrade mycotoxins: a review. Drug Metab Rev. 42(4):612–620.
- Guner KG. 2014. Çeşitli aromatik bitkilerin, meyve kurutmada küf-maya gelişimi, fonksiyonel ve duyusal özeliklere etkileri [Effects of different aromatic plants on fungal growth, functional and sensorial properties of dried fruits] [PhD thesis]. Tekirdag: Namık Kemal University, Graduate School of Natural and Applied Sciences, Department of Food Engineering. p.159.
- Jahn B, Koch A, Schmidt A, Wanner G, Gehringer H, Bhakdi S, Brakhage AA. 1997. Isolation and characterization of a pigmentless-conidium mutant of Aspergillus fumigatus with altered conidial surface and reduced virulence. Infect Immun. 65(12):5110–5117.
- Kells SA, Mason LJ, Maier DE, Woloshuk CP. 2001. Efficacy and fumigation characteristics of ozone in stored maize. J Stored Prod Res. 37(4):371–382.
- Kovalsky P, Kos G, Nährer K, Schwab C, Jenkins T, Schatzmayr G, Sulyok M, Krska R. 2016. Co-occurrence of regulated, masked and emerging mycotoxins and secondary metabolites in finished feed and maize—an extensive survey. Toxins. 8(12):363–391.
- Leeson S, Summers JD. 2005. Commercial poultry nutrition. 3rd ed. Guelph: University Books.
- Lukiewicz S, Reszka K, Matuszak Z. 1980. Simultaneous electrochemical-electron spin resonance (SEESR) studies on natural and synthetic melanins. Bioelectrochem Bioenerg. 7(1):153–165.
- Luo X, Wang R, Wang L, Li Y, Bian Y, Chen Z. 2014. Effect of ozone treatment on aflatoxin B1 and safety evaluation of ozonized corn. Food Control. 37:171–176.
- Mendez F, Maier DE, Mason LJ, Woloshuk CP. 2003. Penetration of ozone into columns of stored grains and effects on chemical composition and processing performance. J Stored Prod Res. 39(1):33–44.
- Naito S. 1989. The influence of ozone treatment on lipids contained in cereal grains, cereal grain powders, peas, beans and pulse products (studies on utilization of ozone for food preservation part VI). J Food Sci Technol. 36(11):878–883.
- Oztekin S, Zorlugenc B, Zorlugenc FK. 2006. Effects of ozone treatment on microflora of dried figs. J Food Eng. 75(3):396–399.
- Pandiselvam R, Subhashini S, Banuu Priya EP, Kothakota A, Ramesh SV, Shahir S. 2019. Ozone based food preservation: a promising green technology for enhanced food safety. Ozone. 41(1):17–34.
- Paquot C. 2013. Standard methods for the analysis of oils, fats and derivatives. 6th ed. IUPAC, Pergamon Press, Oxford, Great Britain.
- Proctor AD, Ahmedna M, Kumar JV, Goktepe I. 2004. Degradation of aflatoxins in peanut kernels/flour by gaseous ozonation and mild heat treatment. Food Addit Contam. 21(8):786–793.
- Qi L, Li Y, Luo X, Wang R, Zheng R, Wang L, Li Y, Yang D, Fang W, Chen Z. 2016. Detoxification of Zearalenone and Ochratoxin A by ozone and quality evaluation of ozonised corn. Food Addit Contam Part A Chem Anal Control Expo Risk Assess. 33(11):1700–1710.
- Remondino M, Valdenassi L. 2018. Different uses of ozone: environmental and corporate sustainability. Literature review and case study. Sustainability. 10(12):4783–4783.
- Ricke SC, Richardson K, Dittoe DK. 2019. Formaldehydes in feed and their potential interaction with the poultry gastrointestinal tract microbial community–a review. Front Vet Sci. 6:188–111.
- Sandhu HPS, Manthey FA, Simsek S, Ohm JB. 2011. Comparison between potassium bromate and ozone as flour oxidants in breadmaking. Cereal Chem. 88(1):103–108.
- SPSS. 2018. PASW® statistics 18. Statistical package for the social sciences. Chicago (IL): SPSS Inc.
- Torlak E, Akata I, Erci F, Uncu AT. 2016. Use of gaseous ozone to reduce aflatoxin B1 and microorganisms in poultry feed. J Stored Prod Res. 68:44–49.
- Tournas V, Stack ME, Mislivec PV, Koch HA, Bandler R. 2017. BAM chapter 18: yeasts, molds and mycotoxins. Bacteriological analytical manual online. US Food and Drug Administration; [accessed 2019 April 15]. https://www.fda.gov/food/laboratory-methods-food/bam-chapter-18-yeasts-molds-and-mycotoxins
- Zotti M, Porro R, Vizzini A, Mariotti MG. 2008. Inactivation of Aspergillus spp. by ozone treatment. Ozone: Science & Engineering. 30(6):423–430.