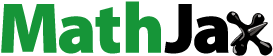
Abstract
Various studies have evaluated the relationship between cholecalciferol (vitamin D3) and reproductive performance. This study aimed at investigating the effects of maternal D3 supplementation during gestation on reproductive performance and antioxidant capacities of gilts and their offsprings. Twenty-three Landrace × Yorkshire gilts were randomly allocated into two groups and fed on one of the following two diets during gestation: control diet or D3 supplemented diet. It was found that D3 supplementation had a tendency to increase the number of total born and born alive piglets. Moreover, it elevated serum 25-hydroxycholecalciferol concentrations in gilts and newborn piglets. Besides, D3 supplementation improved the activities of antioxidant enzymes (GSH-Px, T-AOC and T-SOD) in the blood of gilts, umbilical cord and newborn piglets and piglets’ liver, while serum malondialdehyde concentrations of gilts and umbilical cord were reduced in the D3 group. In addition, D3 supplementation upregulated the expression of antioxidant related genes in the placenta and piglets’ liver. In conclusion, D3 supplementation during gestation might improve antioxidant capacities in gilts, placenta and in newborn piglets. Therefore, D3 supplementation has the potential to enhance the reproductive performance of gilts.
D3 supplementation has a significant role in the nutrition of gilts.
Maternal D3 supplementation during gestation increased the number of born and born alive piglets.
Maternal D3 supplementation during gestation improved the antioxidant capacities of gilts, placenta and newborn piglets.
HIGHLIGHTS
Introduction
Various studies have evaluated the relationship between cholecalciferol (vitamin D3) deficiency and autoimmune diseases, intestinal microbiota disorders and reproductive disturbance (Simmons et al. Citation2000; Holick Citation2004; Coffey et al. Citation2012; Ooi et al. Citation2013). Sows, which are often raised in confinement facilities, exhibit inhibited capacities for endogenous D3 production (Thayer et al. Citation2019). Therefore, D3 supplementation might be beneficial for sow’s health.
It has been reported that D3 has various effects on reproductive performance (Weber et al. Citation2014). Sows fed on higher doses (1400 or 2000 IU/kg) of D3 were found to have less stillborn piglets compared to those fed on lower doses (200 and 800 IU/kg) (Lauridsen et al. Citation2010). Zhou et al. reported that numbers of total born and born alive piglets were significantly increased by 50 μg/kg 25-hydroxycholecalciferol (25-OHD3) supplementation during gestation (Zhou et al. Citation2017). However, findings on the effects of D3 on reproductive performance of sows have not been conclusive. For instance, Thayer et al. reported that the performance of sows and suckling piglets were not affected by maternal 25-OHD3 (50 μg/kg) supplementation, compared to the control group (Thayer et al. Citation2019). Flohr et al. documented that there were no significant differences in the number of total born and stillborn piglets between sows fed on high D3 dose (2720 IU/kg) and sows fed on low doses (680 and 1360 IU/kg) (Flohr et al. Citation2014). Moreover, the specific mechanism through which D3 improves sow performance has not been established.
Classically, D3 has been implicated in calcium and phosphate homeostasis, as well as bone health. Active vitamin D analog was shown to upregulate the expression of nuclear factor E2-related factor 2 (NRF2), which activated the expressions of various reactive oxygen species (ROS) detoxifying and antioxidant genes, while suppressing nuclear factor-kappa B (NF-κB) and NADPH oxidase activity to improve antioxidant capacities in diabetic rat (Nakai et al. Citation2014). In Caenorhabditis elegans, D3 promoted protein homeostasis and slow ageing by upregulating IRE-1, XBP-1, and SKN-1, which regulate a wide range of stress responses, detoxification factors while suppressing irreversible protein oxidation, nitration, and carbonylation (Mark et al. Citation2016). Vitamin D suppresses ROS levels by improving mitochondrial functions and integrity, and by suppressing the NF-κB inflammatory pathway (Ryan et al. Citation2016; Kim et al. Citation2020). A recent study indicated that D3 may prevent human umbilical vein endothelial cell death by inhibiting superoxide anion generation, maintaining mitochondrial functions and cell viability, activating survival kinases, and inducing NO production (Uberti et al. Citation2014). Meanwhile, 1,25(OH)2D3 alleviated oxidative stress through the NF-κB/SOD signalling pathway to slow down male reproductive senescence in ageing mice (He et al. Citation2021). However, the effects of maternal D3 supplementation on antioxidant capacities in gilts and piglets have not been clearly established.
During gestation, the placenta and foetus secrete elevated levels of reactive oxygen species (ROS) (Al-Gubory et al. Citation2010). The imbalance between ROS levels (oxidative stress) and antioxidants is a feature of normal pregnancy. The oxidative stress induced by elevated ROS levels has been attributed to impaired rates of successful pregnancy (Agarwal et al. Citation2006; Zenclussen and Hämmerling Citation2015; Mullen et al. Citation2020). Oxidative stress affects embryonic and foetal development by modifying key transcription factors, including hypoxia-inducible factor (HIF-1), nuclear factor-kB (NF-kB), activator protein-1 (AP-1) and p53 (Dennery Citation2004). Moreover, oxidative stress is involved in the development of different placental lesions, including maternal or foetal vascular malperfusion or chronic villitis, leading to a decrease in the exchange of nutrients and oxygen between the mother and foetus (Schoots et al. Citation2018). Oxidative stress is associated with the pathogenesis of various reproductive diseases, such as endometriosis, infertility, polycystic ovarian syndrome (PCOA), premature delivery and abortion (Agarwal et al. Citation2012). Elevated maternal oxidative stress levels can affect their offsprings, which increases the risk of metabolic diseases in later life (Yin et al. Citation2013). Some nutrients are beneficial for embryonic and foetal survival as well as development by improving maternal, placental and foetal antioxidant capacities (Lin et al. Citation2012; Mou et al. Citation2020). Furthermore, it has not been determined whether maternal D3 supplementation during gestation can improve antioxidant capacities of gilts and piglets, and whether it has a beneficial effect on reproductive performance of gilts. Therefore, the study aimed at evaluating the effects of D3 supplementation during gestation on reproductive performance and antioxidant capacities of gilt and their offsprings.
Materials and methods
Experimental animals and study design
A total of 23 gilts (Landrace × Yorkshire) with similar body weights (BW) (initial body weight 163.73 ± 1.62 kg) and backfat thickness (initial backfat thickness 18.12 ± 0.47 mm) were used in this study. Gilts were artificially inseminated with pooled semen obtained from 2 purebred Duroc boars with the same genetic background (housed in the Research Farm) on the day of oestrus, and then, 24 h and 36 h later. Then, gilts were randomly assigned into two groups and provided with any one of the following two diets during gestation: control diet (CON, basal diet, 800 IU/Kg D3, n = 12), and the D3 supplemented diet (D3, basal diet + D3, 2000 IU/Kg D3, n = 11). D3 was premixed and added to both diets. D3 (500 000 IU/Kg) was obtained from Chengdu Kefei Feed Technology Co. Ltd. Nutrition contents in the basal diet are showed in Table , which met or exceeded the daily recommendations of the National Research Council.
Table 1. Ingredients and chemical compositions of basal diet (as-fed basis, %).
During gestation, the gilts were provided with 2.34 kg diet daily (9:00 and 15:00) from day 0 to day 90 of gestation and 2.92 kg diet daily (9:00 and 15:00) from day 91 of gestation to parturition. Gilts were moved to the farrowing pen on day 107 of gestation. At farrowing, the numbers of born alive, stillborn and mummy of newborn piglets were recorded. The newborn piglets were kept in piglet incubator away from the sows before suckling till the born of the last piglet of the litter. Then, 6 piglets were randomly selected from each group (male, one piglet per litter with body weight closest to the average body weight of the litter; CON: 1.56 ± 0.06 kg and D3: 1.55 ± 0.04 kg) for blood collection before suckling. After blood collection, the 6 piglets were euthanized by intravenous injection of sodium pentobarbital (60 mg/kg BW) for liver sample collection.
In the gestation room, gilts had a free access to drinking water while the ambient temperature was maintained at 22 °C. The study began with 23 gilts, and the final number of gilts used for the analysis was 22. This was because 1 gilt from the D3 group had only 4 newborn piglets at farrowing and was, therefore, excluded.
Sample collection
Body weights (BW) and backfat thickness of gilts were measured at mating and day 110 of gestation. Backfat thickness was measured at 65 mm to the midline of the dorsal rib (P2) using an ultrasound scanner (Renco Lean-Meater; Renco Corporation, Minnesota, Minnesota, United States).
On the mating day, 6 gilts whose weights were closest to the average weights for each group were randomly selected for blood sample collection. Fasting blood samples (10 mL) of the gilts (n = 6) were obtained from marginal ear veins on day 30, 60, and 90 of gestation and on the farrowing day. Immediately after farrowing, umbilical cord blood was collected from as many umbilical cords as possible. Blood samples were centrifuged at 3500 × g for 20 min at 4 °C. The obtained serum was stored at −80 °C for further analysis.
Placental samples were collected from the placenta of newborn piglets whose BWs were closest to litter average BW immediately after parturition. Placental samples were collected as previously described (Mou et al. Citation2018). Immediately after sacrifice, liver samples were also obtained from newborn piglets whose BWs were closest to litter average BW. Then, placenta and liver samples were rinsed in ice-cold 0.9% NaCl, after which they were snap frozen followed by storage at −80 °C for further analysis. Approximately 0.1 g of frozen liver samples were weighed and used to prepare a 10% homogenate. Liver samples were prepared as previously described (Mou et al. Citation2020).
Biochemical analysis
Determination of 25-OHD3 concentrations
Serum concentrations of 25-OHD3 were determined using a 25-Hydroxy Vitamin D ELISA kit for gilts and piglets (IDS Immunodiagnostic Systems Ltd, Tyne and Wear, UK) as previously described (Zhou et al. Citation2017).
Analysis of oxidant and antioxidant levels
Activities of glutathione peroxidase (GPH-Px), total superoxide dismutase (T-SOD) and total antioxidant capacity (T-AOC) as well as concentrations of malondialdehyde (MDA) in serum and liver lysates were determined using their respective commercial assay kits (Nanjing Jiancheng Institute of Bioengineering, Nanjing, China), according to the manufacturers’ instructions.
RNA extraction and quantitative real-time PCR
Gene expression levels were detected by quantitative real-time PCR (qRT-PCR) as previously described (Bin et al. Citation2017). Briefly, total RNA was extracted from frozen placenta and liver samples using the TRIzol reagent (Invitrogen). Total RNA (1 μg) was used to synthetise cDNA using the Prime-Script™ RT reagent kit (Takara), according to the manufacturer’s instructions. qRT-PCR analyses were performed on an ABI Q5 Prism Sequence Detection System (Applied Biosystems, Foster City, CA, USA) with SYBR Green RT-PCR reagents (Biorad). The PCR protocol was: 1 cycle of 95 °C for 30 s and 40 cycles of 95 °C for 15 s followed by 60 °C for 1 min. The relative mRNA abundance of genes was calculated using the 2-delta CT method with β-actin as the reference gene. The mRNA expression level for each gene in the CON group was set as 1. The primers used in this study are shown in Table .
Table 2. Primer sequences for qRT-PCR.
Statistical analysis
SPSS Statistics 20.0 was used for data analysis. Each gilt or piglet was considered to be an experimental unit. The Shapiro-Wilk test was performed to assess the normality of continuous variables. Differences in reproductive performance as well as antioxidant related indicators of the umbilical cord, placenta and newborn piglets between the two groups were determined by Independent-sample T-test. Two-way repeated-measures ANOVA were used to investigate serum antioxidant indicators and serum 25-OHD3 concentrations of gilts at each stage during gestation. The model is as follows:
where Yijk is the response variable, μ is the overall mean, αi, the fixed effects of dietary D3 supplementation, βj, the fixed effects of gestation stage, (αβγ)ij is the interaction between the dietary D3 supplementation and gestation stage, εijk is the residual error. When the main effects were significant, a Tukey post-hoc was conducted to determine where the differences occurred. Data are expressed as mean ± standard errors. p < .05 was set as the threshold for statistical significance, while tendency was defined as .05 ≤ p < .10.
Results
Effects of maternal D3 supplementation on the reproductive performance of gilts
The number of total born piglets (p = .074) and born alive piglets (p = .073) tended to be increased by maternal D3 supplementation during gestation, when compared to the CON group (Table ). Besides, placenta weights were significantly increased by maternal D3 supplementation (p < .05) compared to the CON group. However, body weights (BWs) and backfat of gilts were not affected by D3 supplementation (Supplementary Table S1). There was no significant difference in BWs of newborn piglets between the D3 and CON groups (Table ).
Table 3. Effects of dietary vitamin D3 supplementation during gestation on the reproductive performance of giltsa,b.
Maternal D3 supplementation increased serum 25-OHD3 concentrations in both gilts and newborn piglets
Serum 25-OHD3 concentrations of gilts were significantly affected by gestation stage (p < .01, Figure ). Gilts of the D3 supplemented group exhibited elevated (p < .01) concentrations of serum 25-OHD3 on days 60 and 90 of gestation and at farrowing, when compared to gilts of CON group (Figure ). Besides, serum concentrations of 25-OHD3 (p < .01) in newborn piglets were significantly elevated by maternal D3 supplementation, compared to the CON group (Figure 1B).
Figure 1. Effects of maternal vitamin D3 supplementation during gestation on serum 25-OHD3 concentrations of gilts and in newborn piglets. A. Serum 25-OHD3 concentrations in sows; B. Serum 25-OHD3 concentrations in newborn piglets. Gx = day x of gestation. Data are expressed as mean ± standard error; n = 6 in each group; CON, basal diet, 800 IU/Kg D3; D3, basal diet + D3, 2000 IU/Kg D3; **, p < .01.
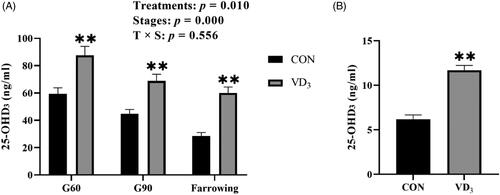
Effects of maternal D3 supplementation on serum antioxidant indicators in gilts
Serum GSH-Px, T-SOD and MDA of gilts were significantly affected by the stage of gestation (p < .01 or p < .05, Table ). The serum GSH-Px activities of gilts on G90 and farrowing were higher than those on G30 and G60. The serum T-SOD activities of gilts at farrowing were higher than those on G30, G60 and G90. Besides, the serum activities of GSH-Px and T-AOC of sows were significantly elevated by D3 supplementation (p < .05). Furthermore, serum MDA concentrations in gilts were affected by interactions between treatments and gestation stage (p < .01, Table ). Serum MDA concentrations in gilts were significantly reduced by D3 supplementation on day 60 of gestation, compared to the CON group (p < .05).
Table 4. Effects of dietary vitamin D3 supplementation during gestation on serum oxidant status of giltsa,b.
Effects of maternal D3 supplementation on antioxidant indicators in umbilical cord blood
Activities of T-SOD and T-AOC (p < .05) in blood obtained from the umbilical cord were higher in the D3 supplemented group than those in the CON group (Table ). Besides, compared to the CON group, MDA concentrations in umbilical cord blood were reduced by maternal D3 supplementation (p < .05).
Table 5. Effects of maternal vitamin D3 supplementation during gestation on oxidant status of umbilical cord blood and newborn pigletsa,b.
Effects of maternal D3 supplementation on antioxidant indicators in newborn piglets
Serum activities of T-SOD (p < .05) in newborn piglets were significantly elevated by maternal D3 supplementation, compared to the CON group (Table ). Besides, compared to the CON group, serum activities of GSH-Px (p = .067) tended to be elevated while serum MDA (p = .063) levels tended to be reduced by maternal D3 supplementation (Table ). However, serum activities of T-AOC in newborn piglets were not affected by maternal D3 supplementation (Table ).
Compared to the CON group, the hepatic MDA concentrations (p = .084) tended to be reduced by maternal D3 supplementation (Table ). Besides, piglets in the D3 group exhibited elevated hepatic T-AOC (p < .05) activities when compared to piglets in the CON group.
Maternal D3 supplementation regulated the expression of redox related genes in the placenta and newborn piglet liver
Maternal D3 supplementation significantly upregulated mRNA expression levels of GPx3 (p < .05) and GPx4 (p < .01) in the placenta, while expression levels of VDR (p = .059) tended to be elevated by maternal D3 supplementation, compared to the CON group (Figure ).
Figure 2. Effects of maternal vitamin D3 supplementation during gestation on the expression of antioxidant activity related genes in the placenta. n = 6 for each group. CAT, catalase; GPx1, glutathione peroxidase 1; GPx2, glutathione peroxidase 2; GPx3, glutathione peroxidase 3; GPx4, glutathione peroxidase 4; SOD1, superoxide dismutase 1; SOD2, superoxide dismutase 1; NRF2, nuclear erythroid 2-related factor 2; VDR, vitamin D receptor. CON, basal diet, 800 IU/Kg D3; D3, basal diet + D3, 2000 IU/Kg D3; Data are normalised against β-actin, with results expressed relative to the control using the 2-delta CT method (Ct is cycle threshold); #, 0.05< p < .10; *, p < .05; **, p < .01.
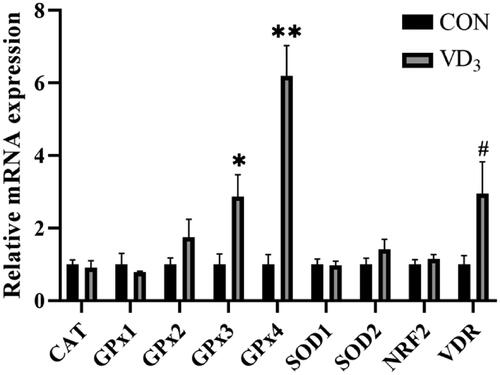
Moreover, maternal D3 supplementation significantly upregulated mRNA expression levels of GPx1 (p < .01), GPx3 (p < .01), GPx4 (p < .01), SOD2 (p < .01) and NRF2 (p < .05) in the liver of newborn piglets (Figure ).
Figure 3. Effects of maternal vitamin D3 supplementation during gestation on the expression of genes related to antioxidant activities in newborn piglet liver. n = 6 for each group. CAT, catalase; GPx1, glutathione peroxidase 1; GPx2, glutathione peroxidase 2; GPx3, glutathione peroxidase 3; GPx4, glutathione peroxidase 4; SOD1, superoxide dismutase 1; SOD2, superoxide dismutase 1; NRF2, nuclear erythroid 2-related factor 2; VDR, vitamin D receptor. CON, basal diet, 800 IU/Kg D3; D3, basal diet + D3, 2000 IU/Kg D3; Data are normalised against β-actin, with results expressed relative to the control using the 2-delta CT method (Ct is cycle threshold); *, p < .05; **, p < .01.
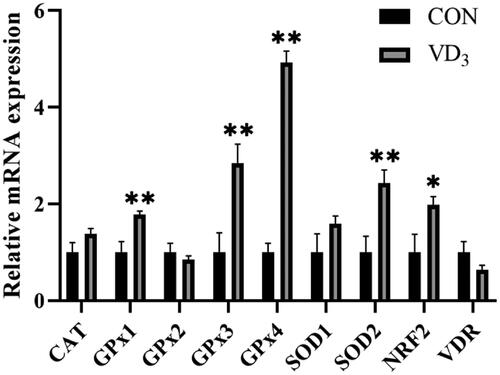
Discussion
Oxidative stress impairs female health and the reproductive process (Agarwal et al. Citation2005; Lu et al. Citation2018). Maternal oxidative stress can be passed on to offsprings, thereby damaging the health of offsprings in later life (Yin et al. Citation2013). Therefore, this study aimed at evaluating the effects of maternal D3 supplementation during gestation on reproductive performance and on the antioxidant abilities of gilts and their newborn offsprings.
In this study, maternal dietary 2000 IU/kg D3 supplementation during gestation had a tendency to increase the number of total born and live born piglets without change the average born alive weight, compared to the CON group. Sows supplemented with 200 μg/day 25-OHD3 from day 90 of gestation to farrowing had increased numbers of total born and live born piglets (Wang et al. Citation2020). A recent study reported that numbers of total born and live born piglets were significantly increased by dietary supplementation of 50 μg/kg 25-OHD3 during gestation (Zhou et al. Citation2017). The above findings imply that D3 can effectively improve the reproductive performance of sows. However, the effects of D3 on reproductive performance of sows are not conclusive. For instance, Weber et al. reported that total numbers of born and live born piglets were not affected by dietary D3 supplementation (2000 IU/kg) and different parities (1–4) of sows (Weber et al. Citation2014). In a previous study, variations in D3 supplementation concentrations (800–9600 IU/kg) had no effect on the reproductive performance of sows (Flohr et al. Citation2016). Differences in outcomes among studies were attributed to different doses and forms of D3, treatment times, or to the different parities of sows (Flohr et al. Citation2014, Citation2016). Besides, there were no significant changes in BW and backfat of gilts in the two treatments. It is worth noting that gilts were used in this experiment, therefore, their growth needs could also be attributed to this outcome. A previous study showed that 25-OHD3 supplementation during gestation had no significant effects on the growth performance of gilts (Zhou et al. Citation2017).
Serum 25-OHD3 is the main circulatory form of D3 and is used to determine the body’s vitamin D status (Holick Citation2007). A previous study showed that 2720 IU/lb D3 supplementation during gestation increased serum levels of 25-OHD3 in sows and in piglets (Flohr et al. Citation2014). Zhang et al. reported that maternal 25-OHD3 supplementation elevated serum levels of 25-OHD3 in sows and in piglets (Zhang et al. Citation2020). In agreement with these studies, we found that gilts supplemented with D3 during gestation exhibited elevated concentrations of serum 25-OHD3, compared to the control group, as well as to newborn piglets. Genomic actions of VDR are important in VD3 functions, and it is regulated by 1,25(OH)2D3 (Christakos et al. Citation1996). VDR, the receptor for the active form (1,25(OH)2D3) of D3, is widely distributed in tissues. 1,25(OH)2D3/VDR/RXR regulates the expression of target genes to execute the traditional genomic functions of D3 (Haussler et al. Citation2011). We found that maternal D3 supplementation during gestation had a tendency to improve the expression of the VDR gene in the placenta.
Severe oxidative stress occurs during gestation, and is one of the reasons that impair the success of female gestation (Hempstock et al. Citation2003; Agarwal et al. Citation2005). Maternal oxidative stress levels, which are correlated with MDA levels, can be transferred to the foetus through the placenta (Takahashi and Oishi Citation2000; Yi et al. Citation2011). Studies have reported on the antioxidant effects of D3, however, the antioxidant properties of vitamin D in pigs have not been reported before. We found that maternal dietary supplementation of 2000 IU/kg D3 significantly elevated serum 25(OH)D3 concentrations in gilts and in newborn piglets, had a tendency to improve the expression levels of VDR in the placenta, significantly improved serum activities of GSH-Px, T-SOD and T-AOC in gilts, umbilical cord blood and in newborn piglets, as well as elevated T-AOC activities in the liver of newborn piglets. Serum MDA concentrations were significantly reduced by D3 supplementation in gilts and umbilical cord blood. As previously mentioned, D3 can exert its antioxidant functions by modifying the activities of antioxidant enzymes. A recent study showed that dietary 50 μg/kg 25(OH)D3 supplementation enhanced serum GSH-Px activities in sows and serum SOD activities in suckling piglets, compared to the control group (Zhang et al. Citation2020). In a previous study, D3 pre-treatment (25 μg/kg) for 1, 24 and 48 h before LPS injection alleviated LPS-induced mice renal oxidative stress, by elevating the expression of inducible nitric oxide synthase, SOD1 and SOD2 genes in kidneys (Xu et al. Citation2015).
In this study, expression levels of antioxidant activity related genes in the placenta (GPx3 and GPx4) and livers of newborn piglets (GPx1, GPx3, GPx4 and SOD2) were found to be significantly upregulated by maternal D3 supplementation. GPxs are usually involved in defences against oxidative stress (Santi et al. Citation2013). Among them, GPx1 is a major intracellular antioxidant enzyme that is involved in protection against oxidative stress (Spanier et al. Citation2009), GPx3 plays an important role in protecting tissues from oxidative stress by inactivating ROS (Chen et al. Citation2011). GPx4 inhibits lipid peroxidation damage by decreasing the levels of phospholipid hydroperoxides in membranes and lipoproteins (Yant et al. Citation2003). SOD2 polymorphisms regulate mitochondrial ROS levels through SOD (Flekac et al. Citation2008). NRF2 is an important node against oxidative stress (Yamamoto et al. Citation2018). NRF2 is negatively regulated by Keap1, and it activates the expression of various antioxidant activity related genes. Chen et al. documented that 1,25(OH)2D3 improves the transcription of NRF2 to exert antioxidant functions (Chen et al. Citation2019). In this study, although the expression levels of VDR in the livers of newborn piglets were not significantly altered after maternal D3 supplementation, expression levels of genes such as NRF2, SOD and GPx were significantly upregulated. A previous study reported that D3 elevated VDR protein expression in cultured keratinocytes, without affecting VDR mRNA expression levels. Moreover, VDR was ubiquitinated in cells, but this ubiquitination was inhibited by D3 (Li et al. Citation1999). Besides, we found that serum 25-OHD3 concentrations in newborn piglets were significantly elevated by maternal D3 supplementation. Therefore, elevated 25-OHD3 concentrations may increase the expression levels of the VDR protein, thereby upregulating the NRF2 level, and further improving the expression of genes associated with antioxidant and related enzyme activities. Maternal D3 supplementation improved antioxidant capacities in gilts, placenta and in newborn piglets by elevating serum 25(OH)D3 concentrations and expression levels of the VDR gene. Improvement in maternal and placental antioxidant capacity ensures a smooth progression of pregnancy, and improves the reproductive performance of gilts. Besides, transmission of placental and umbilical cord antioxidant capacities between gilts and foetus and improvement in VD status might further enhance antioxidant capacities in newborn piglets.
Conclusion
Maternal D3 supplementation during gestation elevated serum 25(OH)D3 levels and might improve antioxidant capacities in gilts, placenta and newborn piglets, induces the expression of antioxidant related genes in the placenta and in newborn piglets’ liver, and has the potential to improve the reproductive performance of gilts. Therefore, D3 supplementation is important in improving reproductive health for both animals and humans.
Ethical approval
All animal procedures were performed in accordance with the Guidelines for the Care and Use of Laboratory Animals of Sichuan Agricultural University. Ethical approval was obtained from the Animal Care and Use Committee of Sichuan Agricultural University (Approval number: DKYB20131704).
Author contributions
The authors’ responsibilities were as follows: BF, LPZ and DW designed the study; LPZ, ZYM, DLM, LH, MY and DJD conducted the experiments; HY, XMJ, ZFF, LQC, YZ, SYX. YL, JL, CH, YFZ and LXL analysed the data; LPZ wrote the manuscript; BF and DW revised the manuscript; all authors read and approved the manuscript for publication.
tjas_a_1961616_sm7532.docx
Download MS Word (16.8 KB)Disclosure statement
No potential conflict of interest was reported by the author(s).
Data availability statement
For privacy reasons, the data analysed in this manuscript is not publicly available, however, it is available from the corresponding author upon reasonable request.
Additional information
Funding
References
- Agarwal A, Aponte-Mellado A, Premkumar BJ, Shaman A, Gupta S. 2012. The effects of oxidative stress on female reproduction: a review. Reprod Biol Endocrinol. 10:49.
- Agarwal A, Gupta S, Sharma R. 2005. Role of oxidative stress in female reproduction. Reprod Biol Endocrinol. 3(1):28–28.
- Agarwal A, Gupta S, Sikka SC. 2006. The role of free radicals and antioxidants in reproduction. Curr Opin Obstet Gynecol. 18(3):325–332.
- Al-Gubory KH, Fowler PA, Garrel C. 2010. The roles of cellular reactive oxygen species, oxidative stress and antioxidants in pregnancy outcomes. Int J Biochem Cell Biol. 42(10):1634–1650.
- Zenclussen AC, Hämmerling GJ. 2015. Cellular regulation of the uterine microenvironment that enables embryo implantation. Front Immunol. 6(17):321.
- Bin F, Xiaohua H, Dandan J, Lun H, Yong Z, De W. 2017. Endoplasmic reticulum stress inducer tunicamycin alters hepatic energy homeostasis in mice. Int J Mol Ences. 18(8):1710.
- Chen B, Rao X, House MG, Nephew KP, Cullen KJ, Guo Z. 2011. GPx3 promoter hypermethylation is a frequent event in human cancer and is associated with tumorigenesis and chemotherapy response. Cancer Lett. 309(1):37–45.
- Chen L, Yang R, Qiao W, Zhang W, Chen J, Mao L, Goltzman D, Miao D. 2019. 1,25-Dihydroxyvitamin D exerts an antiaging role by activation of Nrf2-antioxidant signaling and inactivation of p16/p53-senescence signaling. Aging Cell. 18(3):e12951.
- Christakos S, Raval-Pandya M, Wernyj RP, Yang W. 1996. Genomic mechanisms involved in the pleiotropic actions of 1,25-dihydroxyvitamin D3. Biochem J. 316(Pt 2):361–371.
- Coffey JD, Hines EA, Starkey JD, Starkey CW, Chung TK. 2012. Feeding 25-hydroxycholecalciferol improves gilt reproductive performance and fetal vitamin D status1. J Anim Sci. 90(11):3783–3788.
- Dennery PA. 2004. Role of redox in fetal development and neonatal diseases. Antioxid Redox Signal. 6(1):147–153.
- Flekac M, Skrha J, Hilgertova J, Lacinova Z, Jarolimkova M. 2008. Gene polymorphisms of superoxide dismutases and catalase in diabetes mellitus. BMC Med Genet. 9(1):30.
- Flohr JR, Tokach MD, Dritz SS, Derouchey JM, Goodband RD, Nelssen JL, Bergstrom JR. 2014. An evaluation of the effects of added vitamin D3 in maternal diets on sow and pig performance. J Anim Sci. 92(2):594–603.
- Flohr JR, Woodworth JC, Bergstrom JR, Tokach MD, Dritz SS, Goodband RD, DeRouchey JM. 2016. Evaluating the impact of maternal vitamin D supplementation: I. Sow performance, serum vitamin metabolites, and neonatal muscle characteristics1,2. Journal of Animal Science. 94(11):4629–4642.
- Haussler MR, Jurutka PW, Mizwicki M, Norman AW. 2011. Vitamin D receptor (VDR)-mediated actions of 1α,25(OH)2vitamin D3: genomic and non-genomic mechanisms. Best Pract Res Clin Endocrinol Metab. 25(4):543–559.
- He J, Wang H, Shi J, Shi M, Sun W. 2021. 1,25-Dihydroxyvitamin D deficiency accelerates male reproductive senescence in aging mice and 1,25(OH)2D3 alleviates oxidative stress via NF-κB/SOD. Am J Physiol Endocrinol Metab. 320(4):Ee732–e746.
- Hempstock J, Jauniaux E, Greenwold N, Burton GJ. 2003. The contribution of placental oxidative stress to early pregnancy failure. Hum Pathol. 34(12):1265–1275.
- Holick MF. 2004. Sunlight and vitamin D for bone health and prevention of autoimmune diseases, cancers, and cardiovascular disease. Am J Clin Nutr. 80(6 Suppl):1678S–1688S.
- Holick MF. 2007. Vitamin D Deficiency. N Engl J Med. 357(3):266–281.
- Kim D-H, Meza CA, Clarke H, Kim J-S, Hickner RC. 2020. Vitamin D and Endothelial Function. Nutrients. 12(2):575.
- Lauridsen C, Halekoh U, Larsen T, Jensen SK. 2010. Reproductive performance and bone status markers of gilts and lactating sows supplemented with two different forms of vitamin D. J Anim Sci. 88(1):202–213.
- Li XY, Boudjelal M, Xiao JH, Peng ZH, Asuru A, Kang S, Fisher GJ, Voorhees JJ. 1999. 1,25-Dihydroxyvitamin D3 increases nuclear vitamin D3 receptors by blocking ubiquitin/proteasome-mediated degradation in human skin. Mol Endocrinol. 13(10):1686–1694.
- Lin Y, Han XF, Fang ZF, Che LQ, Wu D, Wu XQ, Wu CM. 2012. The beneficial effect of fiber supplementation in high- or low-fat diets on fetal development and antioxidant defense capacity in the rat. Eur J Nutr. 51(1):19–27.
- Mullen L, Mengozzi M, Hanschmann E-M, Alberts B, Ghezzi P. 2020. How the redox state regulates immunity. Free Radic Biol Med. 157:3–14.
- Lu J, Wang Z, Cao J, Chen Y, Dong Y. 2018. A novel and compact review on the role of oxidative stress in female reproduction. Reprod Biol Endocrinol. 16(1):18–80.
- Mark KA, Dumas KJ, Bhaumik D, Schilling B, Davis S, Oron TR, Sorensen DJ, Lucanic M, Brem RB, Melov S, et al. 2016. Vitamin D Promotes Protein Homeostasis and Longevity via the Stress Response Pathway Genes skn-1, ire-1, and xbp-1. Cell Rep. 17(5):1227–1237.
- Mou D, Ding D, Yan H, Qin B, Dong Y, Li Z, Che L, Fang Z, Xu S, Lin Y, et al. 2020. Maternal supplementation of organic selenium during gestation improves sows and offspring antioxidant capacity and inflammatory status and promotes embryo survival. Food Funct. 11(9):7748–7761.
- Mou D, Wang J, Liu H, Chen Y, Che L, Fang Z, Xu S, Lin Y, Feng B, Li J, et al. 2018. Maternal methyl donor supplementation during gestation counteracts bisphenol A-induced oxidative stress in sows and offspring. Nutrition. 45:76–84.
- Nakai K, Fujii H, Kono K, Goto S, Kitazawa R, Kitazawa S, Hirata M, Shinohara M, Fukagawa M, Nishi S. 2014. Vitamin D activates the Nrf2-Keap1 antioxidant pathway and ameliorates nephropathy in diabetic rats. Am J Hypertens. 27(4):586–595.
- Ooi JH, Li Y, Rogers CJ, Cantorna MT. 2013. Vitamin D regulates the gut microbiome and protects mice from dextran sodium sulfate–induced colitis. Journal of Nutrition. 143(10):1679–1686.
- Ryan ZC, Craig TA, Folmes CD, Wang X, Lanza IR, Schaible NS, Salisbury JL, Nair KS, Terzic A, Sieck GC, et al. 2016. 1α,25-Dihydroxyvitamin D3 regulates mitochondrial oxygen consumption and dynamics in human skeletal muscle cells. J Biol Chem. 291(3):1514–1528.
- Santi C, Tidei C, Scalera C, Piroddi M, Galli F. 2013. Selenium containing compounds from poison to drug candidates: a review on the GPx-like activity. Curr Chem Biol. 7(1):25–36.
- Schoots MH, Gordijn SJ, Scherjon SA, van Goor H, Hillebrands JL. 2018. Oxidative stress in placental pathology. Placenta. 69:153–161.
- Simmons J, Mullighan CG, Welsh KI, Jewell DP. 2000. Vitamin D receptor gene polymorphism: association with Crohn’s disease susceptibility. Gut. 47(2):211–214.
- Spanier G, Xu H, Xia N, Tobias S, Deng S, Wojnowski L, Forstermann U, Li H. 2009. Resveratrol reduces endothelial oxidative stress by modulating the gene expression of superoxide dismutase 1 (SOD1), glutathione peroxidase 1 (GPx1) and NADPH oxidase subunit (Nox4). J Physiol Pharmacol. 60(4):111–116.
- Takahashi O, Oishi S. 2000. Disposition of orally administered 2,2-Bis(4-hydroxyphenyl)propane (Bisphenol A) in pregnant rats and the placental transfer to fetuses. Environ Health Perspect. 108(10):931–935.
- Thayer MT, Nelssen JL, Langemeier AJ, Morton JM, Gonzalez JM, Kruger SR, Ou Z, Makowski AJ, Bergstrom JR. 2019. The effects of maternal dietary supplementation of cholecalciferol (Vitamin D3) and 25(OH)D3 on sow and progeny performance. Transl Anim Sci. 3(2):692–708.
- Uberti F, Lattuada D, Morsanuto V, Nava U, Bolis G, Vacca G, Squarzanti DF, Cisari C, Molinari C. 2014. Vitamin D protects human endothelial cells from oxidative stress through the autophagic and survival pathways. J Clin Endocrinol Metab. 99(4):1367–1374.
- Wang K, Chen Y, Zhang D, Wang R, Zhao Z, Feng M, Wei H, Li L, Zhang S. 2020. Effects of 25-hydroxycholecalciferol supplementation in maternal diets on reproductive performance and the expression of genes that regulate lactation in sows. Anim Sci J. 91(1):e13391.
- Weber GM, Witschi A-KM, Wenk C, Martens H. 2014. Triennial Growth Symposium – effects of dietary 25-hydroxycholecalciferol and cholecalciferol on blood vitamin D and mineral status, bone turnover, milk composition, and reproductive performance of sows12. J Anim Sci. 92(3):899–909.
- Xu S, Chen Y, Tan Z, Xie D, Zhang C, Xia M, Wang H, Zhao H, Xu D, Yu D. 2015. Vitamin D3 pretreatment alleviates renal oxidative stress in lipopolysaccharide-induced acute kidney injury. J Steroid Biochem Mol Biol. 152:133–141.
- Yamamoto M, Kensler TW, Motohashi H. 2018. The KEAP1-NRF2 System: a thiol-based sensor-effector apparatus for maintaining redox homeostasis. Physiol Rev. 98(3):1169–1203.
- Yant L, Ran Q, Rao L, Van Remmen H, Shibatani T, Belter JG, Motta L, Richardson A, Prolla TA. 2003. The selenoprotein GPX4 is essential for mouse development and protects from radiation and oxidative damage insults Free Radical. Free Radic Biol Med. 34(4):496–502.
- Yi B, Kasai H, Lee H, Kang Y, Park JY, Yang M. 2011. Inhibition by wheat sprout (Triticum aestivum) juice of bisphenol A-induced oxidative stress in young women. Mutat Res. 724(1–2):64–68.
- Yin J, Ren W, Liu G, Duan J, Yang G, Wu L, Li T, Yin Y. 2013. Birth oxidative stress and the development of an antioxidant system in newborn piglets. Free Radic Res. 47(12):1027–1035.
- Zhang L, Liu S, Li M, Piao X. 2020. Effects of maternal 25-hydroxycholecalciferol during the last week of gestation and lactation on serum parameters, intestinal morphology and microbiota in suckling piglets. Arch Anim Nutr. 74(6):445–461.
- Zhou H, Chen Y, Zhuo Y, Lv G, Lin Y, Feng B, Fang Z, Che L, Li J, Xu S, et al. 2017. Effects of 25-hydroxycholecalciferol supplementation in maternal diets on milk quality and serum bone status markers of sows and bone quality of piglets. Anim Sci J. 88(3):476–483.