Abstract
This study aimed to investigate the effects of replacement different levels and sources of methionine (Met) with betaine on jejunal morphology, duodenal mitochondrial respiration, and lipid peroxidation in heat-stressed broiler chickens. A total of 1,200 one-day-old Ross 308 broilers were randomly assigned to two similar poultry houses. The experiment was designed as a 2 (ambient temperatures) × 2 (Met sources) × 3 (Met levels) × 2 (betaine amounts) split-plot factorial arrangement. Basal diets (Low-Met) were formulated with DL-or L-Met to meet Ross 308 nutrient recommendations except for Met which was 30% lower than the recommendation. Met level in basal diets was increased to the recommendation and/or 30% more than recommendation (High-Met) by supplemental DL-or L-Met. Betaine was or was not partially substituted at a 30% equivalent level of supplemental DL- or L-Met. HS was induced by increasing ambient temperature to 32 °C for 6 h daily in one house from 10 to 42 d. The highest feed conversion ratio (FCR) was observed in Low-Met diets. Low-L-Met diets showed greatest FCR than Low-DL-Met diets groups. Breast muscle malondialdehyde (MDA) concentration was decreased by increasing dietary Met level under HS. Duodenal MDA concentration and complex (Cox) III activity was lower and higher in L-Met diets than DL-Met diets, respectively. Cox II activity was increased in High-Met diets, and also was improved by betaine replacement. Villus height (VH) and Villus surface (VS) was increased in L-Met diets compared to DL-Met diets. Generally, L-Met was more effective than DL-Met in jejunal morphology, reducing duodenal MDA, and increasing Cox III activity. Betaine had the potential to be a partial replacement for Met.
L-Met was more efficient than DL-Met in duodenal malondialdehyde concentration and complex III activity.
Heat stress reduced the growth performance of broilers through its negative effects on intestinal development and duodenal activity of respiratory chain complexes.
Betaine could have a protective effect on mitochondrial function in the tissues of broiler chickens.
HIGHLIGHTS
Introduction
Heat stress (HS) triggers many metabolic changes in the body by which broiler's welfare and productivity are diminished. HS can induce oxidative damage that may be associated with perturbations in the physiological function of mitochondria, such as a decrease in the activity of mitochondrial respiratory chain complexes and an increase in the production of reactive oxygen species (ROS) (Del Vesco et al. Citation2014). Enhanced production of ROS in the mitochondria may lead to disruption in the structure and function of the intestinal epithelium, damage to the enterocyte membrane, and lipid oxidation (Belhadj Slimen et al. Citation2016).
Methionine (Met) is the main limiting amino acid (AA) in practical poultry diets. Methyl donation, scavenging of oxygen species, antioxidant activity, and being the precursor to glutathione (GSH) have been well-defined as some functional roles of Met in the body (Shen et al. Citation2014). In poultry diets, supplemental Met is provided either as DL-Met, L-Met, or liquid DL-Met hydroxy analogue-free acid (MHA-FA). Animal cells can utilise only L-AAs for protein synthesis, whereas D-AAs need to be converted into L form by D-amino acid oxidase, before being utilised by the body cells (Park et al. Citation2018). Thus, the efficacy of metabolic utilisation of exogenous D-Met relies on how it can be efficiently transformed into L-Met. Between the two pathways of Met uptake (sodium-dependent and sodium-independent), the sodium-independent pathway leads to less D-Met uptake than L-Met and loses energy under HS conditions. Therefore, considering that L-Met may have more efficient advantages compared to D-Met, it can be assumed that growth performance and gut development in broilers fed with a diet supplemented with L-Met will be better than DL-Met (Wickramasuriya et al. Citation2019).
As an alternative for Met, betaine plays an important role in cellular protection against environmental stresses, such as high temperature, and allows cells to continue regular metabolic activities in conditions that normally inactivate them. Furthermore, osmolytes like betaine maintain cellular free water content and ion balance and thus, permit cell proliferation under stress conditions (He et al. Citation2015). Further studies have approved that betaine had a protective effect on the morphology of mitochondria, endoplasmic reticulum, Golgi apparatus, and nuclear DNA via an increasing amount of S-adenosyl methionine (SAM) (Craig Citation2004). Andringa et al. (Citation2010) showed that betaine normalised SAM:S-adenosylhomocysteine (SAH) ratio and maintained mitochondrial genome during mitochondrial superoxide production and preserved mitochondrial respiratory capacity. However, there is a controversy on the ability of betaine for partial sparing of Met, and also the mechanism for the modulating effect of betaine on the activity of mitochondrial complexes is not well-known. Therefore, this study was done to mainly investigate the potential of betaine as a replacement of different sources of Met (L and DL) in maintaining intestinal development and activity of duodenal respiratory enzymes in broiler chickens subjected to HS. The overall hypothesis of this study was that the nutritional bioefficacy of L-Met is higher than that of DL-Met and betaine can partially spare Met in heat-stressed broiler, which would be reflected in the parameters measured in the study.
Materials and methods
Experimental design
Basal diets (Low-Met) were formulated with DL- or L-methionine to meet Ross 308 nutrient recommendations (2014) for starter (1–10 d), grower (11–24 d), and finisher (25–42 d) periods with the exception that amount of Met was 30% lower than the recommendation (Table ). Met levels in basal diets (Low-Met) were increased to the level of recommendation (Rec-Met) and/or 30% more than the recommendation (High-Met) level by adding DL- or L-Met (Table ). Betaine (Sigma Aldrich, St. Louis, MO, USA) was substituted for 30% of DL- or L-Met in Low-, Rec- and High-Met diets according to its methyl donating capacity. Therefore, there were 12 dietary treatments: (1) Low-DL-Met, (2) Low-L-Met, (3) Low-DL-Met + Betaine, (4) Low-L-Met + Betaine, (5) Rec-DL-Met, (6) Rec-L-Met, (7) Rec-DL-Met + Betaine, (8) Rec-L-Met + Betaine, (9) High-DL-Met, (10) High-L-Met, (11) High-DL-Met + Betaine, and (12) High-L-Met + Betaine.
Table 1. Ingredients and nutrient composition of basal diets, as-fed basisa.
Table 2. Analysed vs. calculated methionine (Met) and betaine (Bet) amounts in all diets (g/kg).
A total of 1,200 one-day-old Ross 308 broiler chicks were randomly divided to be kept in two poultry houses. Each house was composed of 60-floor pens (10 birds per pen) with their own feeder and water line. Five pens in each house were given one of 12 experimental diets (five replicates/dietary treatment). The birds had ad libitum access to clean water and feed. Ambient temperature was reduced gradually from 32 to 22 °C by ∼0.5 °C/day and maintained constant until the end of the rearing period. A cyclic HS episode (32 °C/6 h/d) was applied from 11 to 42 d in one of the houses. Relative humidity of ∼60% and a cycle of 23 h light/1 h dark were also considered from day one until the end of the experiment.
Growth performance
All chickens were weighed at the beginning and end of each rearing period and their body weight gain (BWG) was measured. Feed intake (FI) was also recorded periodically by taking into account mortality weights. Feed conversion ratio (FCR) was calculated by dividing FI by BWG.
Sample collection and processing
One chick from each replicate close to the average weight of each pen was selected and killed by cervical dislocation on the 42nd day. No fasting was performed before slaughter. The gastrointestinal tract was excised and contents of the duodenum and jejunum were thoroughly fluxed with normal saline to remove digesta. Finally, 2-cm length from the mid-section of the jejunum was cut and immersed in 10% formaldehyde–phosphate buffer and was maintained for microscopic assessment of mucosal morphology (Burkholder et al. Citation2008). Washed duodenal segments were opened longitudinally to obtain mucosa and were scraped into a microassay tube and were frozen in liquid nitrogen (Ojano-Dirain et al. Citation2005b) for further analyses. The duodenal mucosa and pectoralis major breast muscle samples were stored at −80 °C until further analysis for mitochondria isolation and malondialdehyde (MDA) concentration.
Measuring malondialdehyde concentration
MDA concentration was assessed in breast muscle and duodenal mucosa samples spectrophotometrically at a wavelength of 532 nm according to the method proposed by Botsoglou et al. (Citation1994) and was expressed as ng of MDA per g of tissue.
Isolation of duodenal mitochondria and evaluation of respiratory enzyme activities
Mitochondria were isolated by differential centrifugation (Kolath et al. Citation2006) with some modifications as previously described by Sahebi Ala et al. (Citation2019) (Supplementary Material S1). The activity of respiratory chain complexes was measured in a 96-well plate spectrophotometer at 37 °C with duplicate, using a final volume of 100 μL as described previously (Ojano-Dirain et al. Citation2005a) with minor modifications (see more details in Supplementary Material S1). Measurements were corrected for a path length of the 96-well plate and with appropriate blanks. The used reagents were purchased from Sigma–Aldrich Co. (St. Louis, MO, USA) or Merck Co. (Whitehouse, NJ, USA). Enzymes activities were expressed as units per mg of mitochondrial protein.
Histomorphometry of the jejunum
Segments of the jejunum were cut to the longitudinal axis of the intestine and were embedded in paraffin wax. Transverse sections were cut into parts with 5–6 μm of thickness by a rotary microtome (Leica, Model: JUNG RMZOUS). Then, these sections were placed on glass slides and were stained by eosin and hematoxylin-alcian blue, and were analysed under a light microscope (Carl ZEISS standard 20, Germany) using image-analysis software (Dino-lite, Ver. 3.3.0.0, Korea). There was one cross-section per sample and the mean of three well-oriented intact villi and their associated crypt per cross-section was measured as an average value for each analysis. Morphometric variables were measured by the villus height (VH), crypt depth (CD), VH/CD ratio, and villus width (VW). Villus surface (VS) was calculated using the following formula: 2π × (VW/2) × VH.
Statistical analysis
All the data were subjected to analysis of variance (ANOVA) with a completely randomised design in a 2 × 2 × 3 × 2 split-plot factorial arrangement with two temperature conditions; two sources of Met; three levels of Met; and two levels of betaine (for details on a statistical model, see Supplementary Material S2). Statistical analysis was performed using generalised linear model (GLM) procedure in SAS Institute Inc. (Citation2009) statistical software (SAS Institute Inc., Cary, NC, USA) and differences between means were specified by the Tukeyś post-hoc test. A p-value of <.05 was considered statistically significant.
Results
Growth performance
As shown in Table , cyclic HS had negative effects on FI (p = .025), BWG (p = .008), and FCR (p = .003). The main effects of Met source and partial replacement of betaine on the growth performance of the chicks were not significant (p > .05). Met level had a significant main effect on FI (p = .003), BWG (p < .001), and FCR (p < .001). Although, FI was depressed in High-Met-fed chickens compared to those fed with Low- and Rec-Met diets (p = .003) however, BWG and FCR were improved by the increased Met levels (p < .001). An interaction effect of Met source × Met level was observed for FCR (p = .03) so that, FCR was greater in the birds fed with Low-DL-Met diets than those fed with Low-L-Met diets (Figure ). All other interaction effects were non-significant (p > .05).
Figure 1. Interaction effect of methionine (Met) levels and Met source on feed conversion ratio (FCR) of broilers reared under normal or heat stress conditions. ‘Low-DL or L-Met, 30% lower that Ross 308 recommendation (2014)’; ‘Rec-DL or L-Met, Ross 308 recommended level’; ‘High-DL or L-Met, 30% more than Ross 308 recommendation’. Each of the four-factor combinations had five replicate pens of 10 birds each (r = 5). Values are means with their standard deviations represented by vertical bars. a–cMeans without common superscript are significantly different (p < .05).
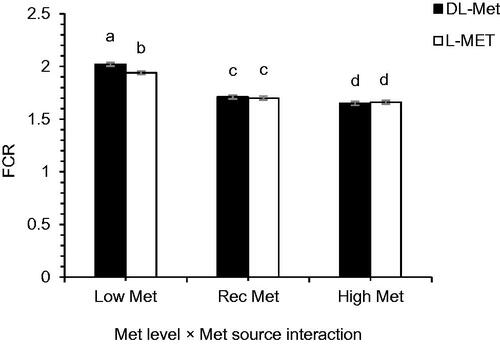
Table 3. Main effects of ambient temperature (Temp), dietary methionine (Met) level and source as well as betaine (Bet) partial replacement on feed intake (FI), body weight gain (BWG), feed conversion ratio (FCR), duodenal respiratory chain complex (Cox) activities (U/mg of mitochondrial protein), and muscle and duodenal malondialdehyde (MDA) concentration (ng MDA/g tissue) in broiler chickens1.
Lipid peroxidation
Cyclic HS elevated MDA concentration in both breast muscles and intestinal tissues. The main effect of Met level on muscle MDA concentration was significant (p = .009), while Met source had a significant effect on the concentration of MDA in duodenal mucosa (p > .05, Table ). No effect was detected regarding partial replacement of betaine for MDA concentration in muscle and duodenal mucosa (p > .05). The interaction effects of Met levels and ambient temperature were pronounced (p = .020, Table ) for MDA concentration in muscle. HS increased concentration of MDA in breast muscle of the birds fed with Low-Met diets (p = .020), whereas no significant difference was observed for MDA concentration of breast between HS-exposed and thermoneutral chickens fed with Rec- or High-Met diets (p > .05, Figure ). A significant interaction effect was also observed between Met source and ambient temperature for the concentration of MDA in duodenal mucosa (p = .038). Under thermoneutral conditions, the birds fed with L-Met diets exhibited lower mucosal MDA concentration than those fed with DL-Met diets (p = .038, Figure ). Although, the main effect of Met level on MDA concentration of duodenal mucosa was non-significant at the highest level of Met (p > .05) however, mucosal MDA concentration was lower in birds fed with a diet supplemented with L-Met than those received Met supplement in DL form (p = .007, Figure ).
Figure 2. Interaction effect of methionine (Met) levels and temperature on breast muscle lipid peroxidation (ng MDA/g wet tissue) of broilers reared under normal or heat stress conditions. ‘Low-DL or L-Met, 30% lower that Ross 308 recommendation (2014)’; ‘Rec-DL or L-Met, Ross 308 recommended level’; ‘High-DL or L-Met, 30% more than Ross 308 recommendation’. Each of the four-factor combinations had five replicate pens of 10 birds each (r = 5). Values are means with their standard deviations represented by vertical bars. a–cMeans without common superscript are significantly different (p < .05).
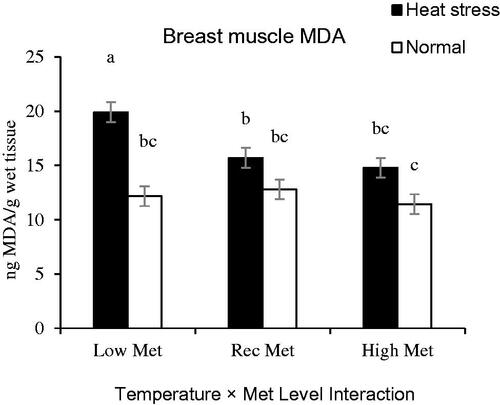
Figure 3. Interaction effect of temperature and methionine (Met) sources on duodenal lipid peroxidation (ng MDA/g wet tissue) of broilers reared under normal or heat stress conditions. ‘Low-DL or L-Met, 30% lower that Ross 308 recommendation (2014)’; ‘Rec-DL or L-Met, Ross 308 recommended level’; ‘High-DL or L-Met, 30% more than Ross 308 recommendation’. Each of the four-factor combinations had five replicate pens of 10 birds each (r = 5). Values are means with their standard deviations represented by vertical bars. a,bMeans without common superscript are significantly different (p < .05).
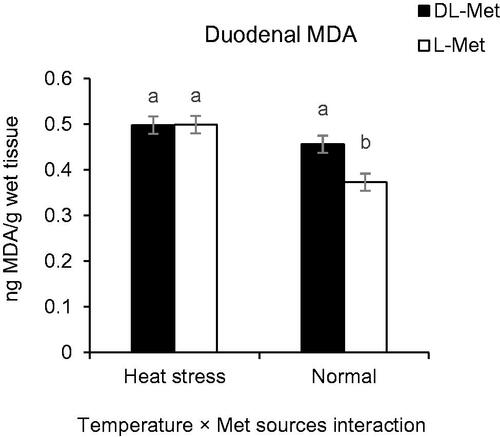
Figure 4. Interaction effect of methionine (Met) levels and sources on duodenal lipid peroxidation (ng MDA/g wet tissue) of broiler reared under normal or heat stress conditions. ‘Low-DL or L-Met, 30% lower that Ross 308 recommendation (2014)’; ‘Rec-DL or L-Met, Ross 308 recommended level’; ‘High-DL or L-Met, 30% more than Ross 308 recommendation’. Each of the four-factor combinations had five replicate pens of 10 birds each (r = 5). Values are means with their standard deviations represented by vertical bars. a,bMeans without common superscript are significantly different (p < .05).
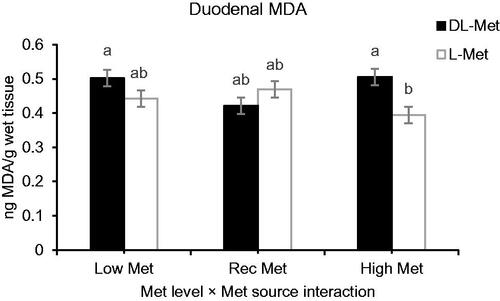
Table 4. Interaction effects of ambient temperature (Temp), dietary methionine (Met) level and source as well as betaine partial replacement on feed intake (FI), body weight gain (BWG), feed conversion ratio (FCR), duodenal respiratory chain complex (Cox) activities (U/mg of mitochondrial protein), and muscle and duodenal malondialdehyde (MDA) concentration (ng MDA/g tissue) in broiler chickensa.
Activity of duodenal mitochondrial complexes
Except for complex IV (p > .05), the activities of other complexes were negatively influenced by HS (p < .05, Table ). The main effects of Met level (p < .001) and partial replacement of betaine (p = .007) were significant on Cox II, whereas the activity of Cox III was influenced by Met source (p = .023). There was a significant interaction effect between Met levels and betaine for Cox II activity (Table , p = .041) so that, partial replacement of the betaine increased Cox II activity in birds fed with a High-Met diet (Figure ). All other interactions were not significant for complex activity (p > .05).
Figure 5. Interaction effect of methionine (Met) levels and betaine replacement on duodenal complex II activity (U/mg of mitochondrial protein) of broilers reared under normal or heat stress conditions. ‘Low-DL or L-Met, 30% lower that Ross 308 recommendation (2014)’; ‘Rec-DL or L-Met, Ross 308 recommended level’; ‘High-DL or L-Met, 30% more than Ross 308 recommendation’. Each of the four-factor combinations had five replicate pens of 10 birds each (r = 5). Values are means, with their standard deviations represented by vertical bars. a–cMeans without common superscript are significantly different (p < .05).
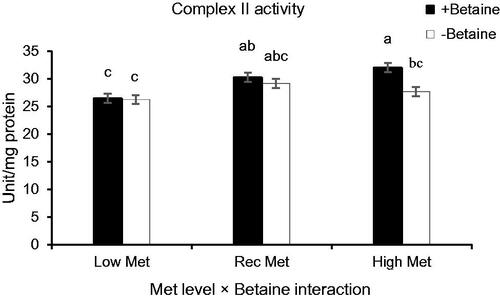
Jejunal morphology
The chickens reared under the HS condition showed lower VH, VW, and VS compared to those reared under thermoneutral conditions (p < .05). The birds fed with diets supplemented with L-Met had greater VH (p = .044) and VS (p = .021) compared to those fed with diets supplemented with DL-Met (Table ). No significant main effects of Met level and partial replacement of betaine were observed for jejunal morphology parameters (p > .05).
Table 5. Main effects of ambient temperature (Temp), dietary methionine (Met) level and source as well as betaine (Bet) partial replacement on jejunum morphology in broiler chickens1.
Discussion
Growth performance
The detrimental effects of HS on the growth performance of broiler chickens have been well-documented in a large body of literature (Belhadj Slimen et al. Citation2016), and are attributed to many physiological modifications, such as the increased production of ROS as well as changes in appetite regulation-related peptides and intestinal morphology (Song et al. Citation2014). In the present study, as expected, cyclic HS conditions had significant negative effects on FI, BWG, and FCR of the chickens. Higher energy loss via the elevated respiration, reduced FI, and retardation of nutrient digestibility due to the decreased VH, VW, and VS may be the cause of the reduced growth performance under acute HS in broilers (Wickramasuriya et al. Citation2019, Mello et al. Citation2015).
The main objective of this study was evaluating the potential of different Met sources and levels as well as partial replacement of Met by betaine in modulating the negative effects of HS. However, herein, no relationship was found between experimental diets and ambient temperature regarding the growth performance of the chickens. Our results were in agreement with other studies (Zulkifli et al. Citation2004; Ribeiro et al. Citation2005), showing minimal or non-significant response by Met sources and levels and betaine supplementation on animalś performance under HS condition. However, He et al. (Citation2015) reported the improved weight gain and feed efficiency through dietary supplementation of betaine in broilers subjected to chronic HS. Differences in these results can be attributed to the duration and extent of HS, growth stages, and types of broilers and diets.
In the present study, higher efficiency of L-Met for FCR was observed than DL-Met in the overall rearing period. FCR was improved in Low-Met-fed chickens when DL-Met was replaced by L-Met, most likely due to the higher relative bioavailability of L-Met compared to DL-Met. According to Shen et al. (Citation2015) relative bioavailability of L-Met to DL-Met for FCR is equal to 140.7. Park et al. (Citation2018) stated that higher relative bioavailability of L-Met than DL-Met improved FCR from 1.70 to 1.63 in turkey poults. Zeitz et al. (Citation2019) showed that plasma concentrations of D‐Met and total Met concentrations were higher in piglets fed with DL‐Met -supplemented diet. They stated that between Met enantiomers, D‐Met was eliminated from plasma at a slower rate than L‐Met, showing a certain time delay after feeding until the major part of the D‐Met is converted into L‐Met.
In contrast to these results, Dilger and Baker (Citation2007) reported that the effectiveness of DL-Met for weight gain and feed efficiency was not less than that of L-Met when young chicks were fed with either purified or practical-type diets deficient in sulphur amino acids. One possible mechanism underlying the observed effect is that the improved utilisation of L-Met may enhance jejunal development by longer villus and greater surface villus area as observed in this study. It seems that the efficiency of DL-Met for chickens may vary with respect to several factors, such as dietary factors and the age of chickens. Independent of Met source and level, partial replacement of Met by betaine had no significant effect on the growth performance of the chicks. This result suggested that DL- and L-Met supplements could be replaced by betaine at a rate of 30% in the diet of broiler chickens without any negative effect on growth performance.
Lipid peroxidation
The increased level of MDA in the tissues subjected to the HS condition observed in the present study was in agreement with the previous reports (Huang et al. Citation2015). Huang et al. (Citation2015) reported the increased levels of MDA in breast and thigh muscles representing oxidative damage caused by HS in skeletal muscles of broilers, regardless of muscle type. Yang et al. (Citation2010) documented that as the temperature was elevated, MDA level was increased in serum and liver, and MDA level returned to pre-heat level by removing it. Via the Fenton reaction, H2O2 reaction with mitochondrial Fe2+ leads to the production of highly reactive hydroxyl radical (OH•), which in turn increases lipid peroxidation and MDA concentration.
The methyl donating role of Met and its indirect effect on GSH synthesis may have a key effect on the health of gastrointestinal tract (Shen et al. Citation2014). Our results showed that increasing Met level reduced MDA concentration (High-Met= 13.1 vs. Low-Met= 16.0). This finding is in agreement with a previous study that showed supplementation of Met slightly more than the recommendations increased GSH level in liver and thigh muscle of broilers subjected to HS (Zeitz et al. Citation2020a) moreover, there were lower concentrations of cholesterol oxidation products in heat-processed thigh muscle (Zeitz et al. Citation2020b). This may be because as Met access is increased, glutathione forms a storehouse of available cysteine (Ingenbleek and Kimura Citation2013).
Our results indicated that the concentration of MDA as an indicator of lipid peroxidation may be influenced by the source of Met supplement in the diet. Apart from Met level, L form of Met showed a greater modulating effect on lipid peroxidation in duodenum mucosa than DL from. Furthermore, at Low-Met level, L-Met supplement was more efficient than DL-Met to modulate lipid peroxidation in the breast muscle of the chickens reared under the HS condition. These findings are in accordance with the study by Shen et al. (Citation2015), who indicated that L-Met had a higher potential to modulate MDA concentration of intestinal mucosa than DL-Met. Met sulfoxide is formed by a variety of ROS due to their reaction with Met residues in proteins. Reduced Met sulfoxide is catalysed back to L-Met by Met sulfoxide reductases, consequently scavenging ROS, while D-Met could not be reduced back to D-Met after oxidisation to D-Met sulfoxide (Cudic et al. Citation2016). Thus, D-Met has less antioxidant activity in mucosal cells than L-Met.
Similar to Met, scavenging of free radicals and methyl donor properties have also been reported for betaine (Alirezaei et al. Citation2011). Fu et al. (Citation2016) stated that the birds fed with diets supplemented with betaine replaced by DL-Met at 25, 50, and 100% equivalent levels had similar MDA content in breast muscle compared to those fed with the control diet. In the current study, it was found that partial replacement of both DL- or L-Met at 30% equivalent level by betaine had no significant effect on the jejunal morphology and MDA concentration of breast muscle under thermoneutral or HS conditions. Together, these findings confirmed the potential of methyl donors to modulate lipid peroxidation in the duodenal mucosa.
Activity of duodenal mitochondrial complex
The level and activity of mitochondrial enzymes reflect oxidative capacity for adenosine triphosphate (ATP) production in animals, and enough energy production through oxidative phosphorylation in mitochondria ensures cell survival. Therefore, inefficiencies in duodenal mitochondrial function may reduce the efficiency of converting feed into demand tissues or eviscerated body mass (Ojano-Dirain et al. Citation2005a). In the current study, the activity of mitochondrial respiratory chain complexes is negatively influenced by cyclic HS that is in agreement with the previous reports (Yang et al. Citation2010). Therefore, growth performance in HS-exposed chickens than those reared under thermoneutral conditions may partially be associated with lower activity of mitochondrial complexes. Although, dietary treatments had no significant modulating effect on HS-induced reduction in the activity of duodenal complexes, a positive effect of Met level was observed on the activity of Cox II. Del Vesco et al. (Citation2014) revealed that under HS conditions where H2O2 production was increased, Met supplementation could attenuate ROS-induced damage, possibly due to its participation in the biosynthesis of GSH. Bottje and Carstens (Citation2009) hypothesised that the increase in complex II activity could be attributed to ROS- induced reduction of damages to protein complex by increasing synthesis of GSH peroxidase in treatment containing the highest Met level.
Interestingly, it was observed that partial betaine replacement at a High-Met level diet improved Cox II activity, probably by reducing the generation of nitric oxide (Kharbanda et al. Citation2012) and stimulating mitochondrial biogenesis (Lee Citation2015). These findings suggested that when dietary Met has been enough to meet the chickens' requirement, dietary inclusion of betaine may have a positive effect on duodenal Cox II activity. Furthermore, these findings provide an insight into the effect regarding the relationship between Met, betaine, and HS on mitochondrial function in broilerś duodenal tissue that has not been reported in the literature to date and maybe the starting point of further studies.
Higher activity of Cox III in duodenal tissue of chickens fed with L-Met compared to DL-Met-fed birds may be attributed to the higher affinity of transport systems for L-isomer (Dilger and Baker Citation2007) and greater efficiency of L-Met for GSH production in the gastrointestinal tract (Shen et al. Citation2015) rather than D- isomer. However, the underlying mechanisms would remain unknown.
Intestinal morphology
Disruption of intestinal epithelium integrity is one of the physiological changes in response to stress conditions (Collins et al. Citation2012). In this study, cyclic HS decreased VH, VW, and VS of the chickens. Burkholder et al. (Citation2008) showed that birds subjected to 30 °C for 24 h presented a decrease in crypt depth than those raised at 23 °C. In contrast, Quinteiro-Filho et al. (Citation2012) did not find any differences in the villus and crypt structures in birds subjected to HS conditions (31 and 36 °C). These inconsistencies in reports are probably due to the amount and duration of exposure to HS (Uni et al. Citation2001).
Villus height and surface area in the birds fed with L-Met were greater than those fed with DL-Met diets. Inconsistent with our results, Shen et al. (Citation2015) reported that L-Met as a direct source of Met supplement was more efficient than DL-Met for intestinal development. Zeitz et al. (Citation2019) hypothesised that higher L‐Met availability supported the production of the Met metabolite, SAM, which is used for the synthesis of polyamines that are crucial for intestinal epithelial growth and integrity. Also, polyamine reduction has been shown to decrease gene expression of tight junction proteins involved in gut barrierś function (Guo et al. Citation2005). Therefore, L‐Met may support gut barrierś function and villi development better than DL‐Met, as already reported for gut morphology in weaned piglets (Shen et al. Citation2014) and broiler chicks (Shen et al. Citation2015). Intestinal morphology of chickens remained unchanged by partial replacement of DL- or L-Met by betaine at Low-, Rec-, and/or High-Met levels. Therefore, it can be concluded that betaine and Met may have a similar function in intestinal morphology. Some studies have indicated that betaine improved the development of the intestinal tissues due to its osmolyte action and consequently, promoting nutrient digestibility and absorption (Eklund et al. Citation2005). When intestinal cells are exposed to ionic and osmotic stress, betaine is accumulated in stressed cells and retains intestinal cells through the replacement of inorganic ions, preventing inactivation of enzymes as well as cell membranes (Petronini et al. Citation1992).
Conclusion
Our results indicated that cyclic HS negatively influenced growth performance, intestinal development, and duodenal activity of respiratory chain complexes. These negative effects of HS were not modulated by dietary Met supplementation (DL or L form) at levels of 30% lower, equal, or higher than the recommendation. Lipid peroxidation was elevated in the breast muscle of chickens by HS, especially in the chickens fed with Low-Met diets. L-Met compared to DL form of Met supplement was more efficient to improve jejunal morphology and Cox III activity and also to reduce the duodenal concentration of MDA. All of these results were also observed when DL- or L-Met was partially replaced by betaine at a 30% equivalent level.
Ethical approval
All procedures were approved by the Animal Care and Use Committee of the Ferdowsi University of Mashhad, Mashhad, Iran.
Supplemental Material
Download MS Word (20.1 KB)Disclosure statement
No potential conflict of interest was reported by the author(s).
Data availability statement
The data that support the findings of this investigation are available upon reasonable request from the corresponding author [A. Hassanabadi].
Additional information
Funding
References
- Alirezaei M, Jelodar G, Niknam P, Ghayemi Z, Nazifi S. 2011. Betaine prevents ethanol-induced oxidative stress and reduces total homocysteine in the rat cerebellum. J Physiol Biochem. 67(4):605–612.
- Andringa KK, King AL, Eccleston HB, Mantena SK, Landar A, Jhala NC, Dickinson DA, Squadrito GL, Bailey SM. 2010. Analysis of the liver mitochondrial proteome in response to ethanol and S-adenosyl Met treatments: novel molecular targets of disease and hepatoprotection. Am J Physiol Gastrointest Liver Physiol. 298:732–745.
- Belhadj Slimen I, Najar T, Ghram A, Abdrrabba M. 2016. Heat stress effects on livestock: molecular, cellular and metabolic aspects, a review. J Anim Physiol Anim Nutr. 100(3):401–412.
- Botsoglou NA, Fletouris DJ, Papageorgiou GE, Vassilopoulos VN, Mantis AJ, Trakatellis AG. 1994. Rapid, sensitive, and specific thiobarbituric acid method for measuring lipid peroxidation in animal tissue, food, and feedstuff samples. J Agric Food Chem. 42(9):1931–1937.
- Bottje WG, Carstens GE. 2009. Association of mitochondrial function and feed efficiency in poultry and livestock species. J Anim Sci. 87:48–63.
- Burkholder K, Thompson K, Einstein M, Applegate T, Patterson J. 2008. Influence of stressors on normal intestinal microbiota, intestinal morphology, and susceptibility to Salmonella enteritidis colonization in broilers. Poult Sci. 87(9):1734–1741.
- Collins SM, Surette M, Bercik P. 2012. The interplay between the intestinal microbiota and the brain. Nat Rev Microbiol. 10(11):735–742.
- Craig SAS. 2004. Betaine in human nutrition. Am J Clin Nutr. 80(3):539–549.
- Cudic P, Joshi N, Sagher D, Williams BT, Stawikowski MJ, Weissbach H. 2016. Identification of activators of methionine sulfoxide reductases A and B. Biochem Biophys Res Commun. 469(4):863–867.
- Del Vesco AP, Gasparino E, Grieser DO, Zancanela V, Gasparin FRS, Constantin J, Constantin AR, Neto O. 2014. Effects of Met supplementation on the redox state of acute heat stress–exposed quails. J Anim Sci. 92(2):806–815.
- Dilger RN, Baker DH. 2007. DL-Methionine is as efficacious as L-methionine, but modest L-cystine excesses are anorexigenic in sulfur amino acid-deficient purified and practical-type diets fed to chicks. Poult Sci. 86(11):2367–2374.
- Eklund M, Bauer E, Wamatu J, Mosenthin R. 2005. Potential nutritional and physiological functions of betaine in livestock. Nutr Res Rev. 18(1):31–48.
- Fu Q, Leng ZX, Ding LR, Wang T, Wen C, Zhou YM. 2016. Complete replacement of supplemental dl-methionine by betaine affects meat quality and AA contents in broilers. Anim Feed Sci Technol. 212:63–69.
- Guo X, Rao JN, Liu L, Zou T, Keledjian KM, Boneva D, Marasa BS, Wang JY. 2005. Polyamines are necessary for synthesis and stability of occludin protein in intestinal epithelial cells. Am J Physiol Gastrointest Liver Physiol. 288(6):G1159–69.
- He S, Zhao S, Dai S, Liu D, Bokhari SG. 2015. Effects of dietary betaine on growth performance, fat deposition and serum lipids in broilers subjected to chronic heat stress. Anim Sci J. 86(10):897–903.
- Huang C, Jiao H, Song Z, Zhao J, Wang X, Lin H. 2015. Heat stress impairs mitochondria functions and induces oxidative injury in broiler chickens. J Anim Sci. 93(5):2144–2153.
- Ingenbleek Y, Kimura H. 2013. Nutritional essentiality of sulfur in health and disease. Nutr Rev. 71(7):413–432.
- Kharbanda KK, Todero SL, King AL, Osna NA, McVicker BL, Tuma DJ, Wisecarver JL, Bailey SM. 2012. Betaine treatment attenuates chronic ethanol-induced hepatic steatosis and alterations to the mitochondrial respiratory chain proteome. Int J Hepatol. 2012:962183–962110.
- Kolath WH, Kerley MS, Golden JW, Keisler DH. 2006. The relationship between mitochondrial function and residual feed intake in Angus steers. J Anim Sci. 84(4):861–865.
- Lee I. 2015. Betaine is a positive regulator of mitochondrial respiration. Biochem Biophys Res Commun. 456(2):621–625.
- Mello JL, Boiago MM, Giampietro-Ganeco A, Berton MP, Vieira LD, Souza RA, Ferrari FB, Borba H. 2015. Periods of heat stress during the growing affects negatively the performance and carcass yield of broilers. Archiv Zoot. 64(248):339–345.
- Ojano-Dirain C, Iqbal M, Wing T, Cooper M, Bottje W. 2005a. Glutathione and respiratory chain complex activity in duodenal mitochondria of broilers with low and high feed efficiency. Poult Sci. 84(5):782–788.
- Ojano-Dirain C, Pumford NR, Iqbal M, Wing T, Cooper M, Bottje WG. 2005b. Biochemical evaluation of mitochondrial respiratory chain in duodenum of low and high feed efficient broilers. Poult Sci. 84(12):1926–1934.
- Park I, Pasquetti T, Malheiros RD, Ferket PR, Kim SW. 2018. Effects of supplemental L-methionine on growth performance and redox status of turkey poults compared with the use of DL-methionine. Poult Sci. 97(1):102–109.
- Petronini PG, De Angelis EM, Borghetti P, Borghetti AF, Wheeler KP. 1992. Modulation by betaine of cellular responses to osmotic stress. Biochem J. 282(Pt 1):69–73.
- Quinteiro-Filho WM, Gomes AVS, Pinheiro ML, Ribeiro A, Ferraz-de-Paula V, Astolfi-Ferreira CS, Ferreira AJP, Palermo-Neto J. 2012. Heat stress impairs performance and induces intestinal inflammation in broiler chickens infected with Salmonella enteritidis. Avian Pathol. 41(5):421–427.
- Ribeiro AML, Dahlke F, Kessler ADM. 2005. Methionine sources do not affect performance and carcass yield of broilers fed vegetable diets and submitted to cyclic heat stress. Rev Bras Cienc Avic. 7(3):159–164.
- Sahebi Ala F, Hassanabadi A, Golian A. 2019. Effects of dietary supplemental methionine source and betaine replacement on the growth performance and activity of mitochondrial respiratory chain enzymes in normal and heat stressed broiler chickens. J Anim Physiol Anim Nutr. 103(1):87–99.
- SAS Institute Inc. 2009. SAS statistics user’s guide. Statistical analysis system, 9.2 version. Cary (NC): SAS Institute Inc.
- Shen YB, Ferket P, Park I, Malheiros RD, Kim SW. 2015. Effects of feed grade L-methionine on intestinal redox status, intestinal development, and growth performance of young chickens compared with conventional dl-methionine. J Anim Sci. 93(6):2977–2986.
- Shen YB, Weaver AC, Kim SW. 2014. Effect of feed grade L-methionine on growth performance and gut health in nursery pigs compared with conventional dl-methionine. J Anim Sci. 92(12):5530–5539.
- Song J, Xiao K, Ke YL, Jiao LF, Hu CH, Diao QY, Shi B, Zou XT. 2014. Effect of a probiotic mixture on intestinal microflora, morphology, and barrier integrity of broilers subjected to heat stress. Poult Sci. 93(3):581–588.
- Uni Z, Gal-Garber O, Geyra A, Sklan D, Yahav S. 2001. Changes in growth and function of chick small intestine epithelium due to early thermal conditioning. Poult Sci. 80(4):438–445.
- Wickramasuriya SS, Kim E, Cho HM, Shin TK, Kim B, Lee M, Seo S, Heo JM, Choi H. 2019. Differential effects of dietary methionine isomers on broilers challenged with acute heat stress. J Poult Sci. 56(3):195–203.
- Yang L, Tan GY, Fu QF, Feng JH, Zhang MH. 2010. Effects of acute heat stress and subsequent stress removal on function of hepatic mitochondrial respiration, ROS production and lipid peroxidation in broiler chickens. Comp Biochem Physiol C Toxicol Pharmacol. 151(2):204–208.
- Zeitz JO, Ehbrecht T, Fleischmann A, Most E, Gessner DK, Friedrichs S, Sparenberg M, Failing K, Whelan R, Lütjohann D, et al. 2020b. Effect of DL-methionine supplementation on tissue and plasma antioxidant status and concentrations of oxidation products of cholesterol and phytosterols in heat-processed thigh muscle of broilers. Animals. 10:2050.
- Zeitz JO, Fleischmann A, Ehbrecht T, Most E, Friedrichs S, Whelan R, Gessner DK, Failing K, Lütjohann D, Eder K. 2020a. Effects of supplementation of DL-methionine on tissue and plasma antioxidant status during heat-induced oxidative stress in broilers. Poult Sci. 99(12):6837–6847.
- Zeitz JO, Kaltenböck S, Most E, Eder K. 2019. Effects of L-methionine on performance, gut morphology and antioxidant status in gut and liver of piglets in relation to DL-methionine. J Anim Physiol Anim Nutr. 103(1):242–250.
- Zulkifli I, Mysahra SA, Jin LZ. 2004. Dietary supplementation of betaine (Betafin®) and response to high temperature stress in male broiler chickens. Asian Australas J Anim Sci. 17(2):244–249.