Abstract
Acute-phase proteins (APPs) are known to mediate the body's first line of defence against external insults in poultry and there is a growing interest to use the proteins to diagnose poultry health and physiological status. However, there is no report describing APPs during the prenatal and neonatal stages in poultry. The objectives of the study were to determine the presence and circulating concentrations of APPs in the embryos, and newly hatched chicks. Cobb 500™ eggs were incubated under a standard condition for 21 d. Blood samples were collected from embryos on days 14 (ED 14), 16 (ED 16), and 18 (ED 18) of incubation as well as newly hatched chicks to determine serum levels of corticosterone (CORT), alpha-1 acid glycoprotein (AGP), ceruloplasmin (CPN), and heat shock protein 70 (Hsp70). Both CORT and CPN showed a dramatic increase from ED 14 to ED 16 (p < .0001) but declined at ED 18 (p = .0004). The newly hatched chicks had elevated CORT (p < .0001) and CPN (p < .0001). Serum AGP rose from ED 14 to ED 18 and was followed by a sharp fall in the newly hatched chicks (p = .0074). Serum concentrations of Hsp70 were consistently elevated across the embryos' developmental stage and remained high in the newly hatched chick (p < .0001). In conclusion, APPs were detected in the blood of chicken embryos and neonates for the first time and the levels vary according to the development stage. These results have raised the need to better understand the physiological relevance of APPs in developing embryos and newly hatched chicks.
Acute-phase proteins were detected and quantified in the circulation of developing chicken embryos and newly hatched chick for the first time.
The magnitude of production of acute-phase proteins varied according to developmental stages.
Acute-phase proteins may be of physiological relevance for the developing chicken embryos.
Highlights
Introduction
The acute phase response is a complex systemic early-defense system of reactions that are activated by various noxious stimuli such as inflammation, tissue damage, infection, and tissue injury (González et al. Citation2008; O’Reilly and Eckersall Citation2014). One of the major elements of this response is the increased hepatic synthesis of some plasma proteins, collectively known as acute-phase proteins (APPs) (Gruys et al. Citation2005). There is an increasing interest in APPs as biomarkers of inflammation, infection, and trauma in veterinary science (Gomez-Laguna et al. Citation2011). Recent studies in poultry showed that overcrowding (Najafi et al. Citation2015), feed deprivation (Najafi et al. Citation2016), and corticosterone administration (Zulkifli et al. Citation2014) elevated serum levels of APPs. The functions of the APPs included protease inhibitors, enzymes, transport proteins, coagulation proteins, and modulators of the immune response. Ceruloplasmin is a copper-containing ferroxidase that protects tissues from iron-mediated free radical injury by oxidation of toxic ferrous iron to non-toxic ferric form (Patel et al. Citation2002). Alpha 1-acid glycoprotein is a sialoglycoprotein produced and then secreted typically by hepatocytes (Fournier et al. Citation2000). The primary function of alpha 1-acid glycoprotein is to act as a natural anti-inflammatory factor inhibiting neutrophil activation and increasing the secretion of IL-1 receptor antagonists by macrophages (Murata et al. Citation2004). This has a clearance function in removing bacterial lipopolysaccharide by binding and neutralising their toxicity (Murata et al. Citation2004), and it also acts as a plasma transport protein (Ceciliani and Pocacqua Citation2007).
While ample evidence exists of APPs in juvenile and adult poultry, there is no report describing APP in developing embryos and newly hatched chicks. The embryo is a critical stage of life because it is likely to be more vulnerable to external conditions than are post-hatching individuals (Jezierska et al. Citation2009). Studies in avian species suggested that embryos have physiological ways to cope with their pre-hatching environments (Andrewartha et al. Citation2011). Thus, it is possible that the APPs are of physiological relevance for the developing embryos due to their unspecific antimicrobial effects (Murata et al. Citation2004) and association with restoring homeostasis (Cray et al. Citation2009). The presence of alpha 1-acid glycoprotein in the circulation of bovine foetuses and newborn calves has been reported (Itoh et al. Citation1993). The authors noted a rise in foetal and neonatal isoforms of alpha 1-acid glycoprotein concentrations in foetuses before birth, the highest concentrations at birth, and a decrease during the first three weeks of life to adult values. We hypothesised that APPs would also predominate in the embryos and newly-hatched chicks and that the levels would vary according to the stage of embryonic development. The possibility of using APPs to diagnose the health and physiological status of the chicken embryos and newly hatched chicks can be ascertained only in the light of precise information about the pattern of blood APP levels during the prenatal and neonatal stages.
Administration of exogenous corticosterone was reported to elevate serum levels of CORT, Hsp70, and APPs in broiler chickens (Zulkifli et al. Citation2014). The authors concluded that corticosterone modulated the elicitation of Hsp70 and APPs. It is well documented that stressful stimuli such as heat stress, crating, road transportation, feed restriction, and social isolation may elicit Hsp70 expression in chickens (Al-Aqil and Zulkifli Citation2009; Soleimani et al. Citation2011). Kregel (Citation2002) suggested that heat shock protein plays a role in protecting cells from damage attributed to thermal and nonthermal stressors. Exposing chicken embryos to either cold or heat stress elevated Hsp70 expression in various organs on the 20th day of incubation (Givisiez et al. Citation2001). Scott et al. (Citation1981) reported that corticosterone levels from the 10 to 14 d of incubation fluctuated slightly and increased rapidly until the 16th day of incubation. At this time, serum CORT remained relatively constant through day 18 with an apparent increasing trend up to the day 20th.
The objectives of the study were to determine the presence and serum levels of ceruloplasmin (CPN) and alpha 1-acid glycoprotein (AGP) in the developing chick embryos at 14, 16 and 18 d of incubation, and newly hatched chicks. We selected those sampling ages because most of the APPs are synthesised in hepatocytes (O’Reilly and Eckersall Citation2014) and haemopoiesis in the liver of the chicken embryos begins on day 7 of incubation and peaks on day 14 (Wong and Cavey Citation1992). In the current study, given the relationship between APP and other standard physiological indices of stress in the avian species (Zulkifli et al. Citation2014), we also measured the serum levels of corticosterone (CORT) and heat shock protein 70 (Hsp70) in the present study.
Materials and methods
Animal experimental procedures were conducted following the Guidelines for Experimental Animals approved by the Research Policy on Animal Ethics of Universiti Putra Malaysia. (UPM/IACUC/AUP-R058/2019)
Experimental incubation
A total of 144 Cobb 500™ broiler chicken eggs of a relatively equal weight of 68.29 ± 0.45 g were obtained from a local hatchery. The age of the breeding flocks was 44 weeks. The eggs were fumigated and incubated in a double insulated egg incubator (Brinsea® OvoEasy 190 Advance Series, USA). The incubator’s temperature was maintained at 37.5 °C (precision of 0.5 °C), and humidity at 70% (68–72%). The eggs were turned every two hours. Aeration was provided with a forced draft of air, and the levels of temperature and humidity were uniform throughout the chamber.
Pre-hatching response
Sixteen hatching eggs were randomly selected on days 14 (ED14), 16 (ED16), and 18 (ED18) of incubation, and blood was directly sampled from the vitelline artery and vein into blood tubes according to methods of Scott et al. (Citation1981). Approximately 0.5 mL of blood sample was collected from each embryo. The blood samples were centrifuged at 2000 × g for 15 min at 4 °C and serum was separated and stored at −20 °C until assayed. The embryos were then individually weighed. Serum samples were used for later determination of CORT, CPN, AGP and Hsp70 concentrations. The collection of samples on different days of incubation allowed us to determine the exact detection time of the blood parameters that may have had on the developing embryos.
Hatching response
The remaining number of eggs in the incubator were allowed to hatch. The hatchability rate was recorded with the observations of hatching chicks started from the beginning of the 20th day of incubation until the 23rd day, and those eggs that did not hatch by day 23rd were considered as non-hatchable. The percentage of hatchability was calculated as the number of chicks hatched from the fertile eggs. Collection of blood samples from the newly hatched chicks was carried out through decapitation, and harvested serum was kept at −80 °C until analysis. Serum samples were used to determine the CORT, CPN, AGP, and Hsp70.
Corticosterone assay
According to manufacturer recommendations, serum CORT concentration was determined using commercially available high sensitivity EIA kits (AC-15F1, IDS, Boldon, UK). Cross-reactivity of the CORT antiserum was less than 6.7% and 7.8%, respectively, and the detection limit was 27 ng/mL.
Acute phase proteins assay
The AGP concentration was determined using a commercial ELISA kit specific to chicken (Cat. No.: NB-E60049, Life Diagnostics Inc., West Chester, PA). The level of CPN was determined, as previously explained (Zulkifli et al. Citation2014). 20.375 g of sodium acetate trihydrate was dissolved in 250 mL of distilled water and adjusted to pH 6.2 using glacial acetic acid. Subsequently, 0.615 g of 1,4-phenylenediamine dihydrochloride (Sigma P1519) was added to the prepared buffer and kept in the dark for at least 45 min. Then, 100 µL of the described buffer was mixed with 10 µL of samples or standards and were added to each microplate wells, gently shaken and kept in the dark for 20 min. Right after 20 min, the plate was read using a microplate reader at 550 nm (Multiskan™ FC Microplate Photometer, USA). Serial dilution of standards was prepared using a known CPN concentration against purified CPN (Sigma Chemical Co.). The combination of saline buffers to reach different concentrations of 12.75 (20 µL of pig serum + 60 µL of saline buffer) 6.375, 3.1875, 1.59375, 0.79608, 0.39804, 0.199, and 0.099 mg/mL of CPN.
Heat shock protein 70 assay
Hsp70 determination was performed using a commercial ELISA kit specific to chicken (Cat. No. 201-16-0033, Shanghai Sunred Biological Technology, Shanghai, China) according to manufacturer recommendations. All samples were run in the same assay to prevent inter-assay variability.
Statistical analysis
All data were subjected to ANOVA using the GLM procedure of SAS (SAS Institute Inc., Cary, NC). All data were analysed using the age of sampling as the main effect. When significant effects were found, comparisons among multiple means were made by Duncan's multiple range test. Statistical significance was considered as p < .05.
Results
The recorded hatchability rate was 91%. The weights of embryos and newly hatched chicks are presented in Table . The CORT (Figure ) and CPN (Figure ) showed a similar trend with a 63% increase from ED14 to ED16 (p < .0001) and a 36% decline at ED18 (p = .0004). The newly hatched chicks showed a significant 33% increase in CORT (p < .0001) and 48% increase in CPN (p < .0001). The AGP levels showed a steady rise from ED14 to ED18 (p = .0074), increased by 38% and followed by a 69% decline in the newly hatched chicks (Figure ). The Hsp70 was consistently elevated across the embryos' developmental stage and remained high in the newly hatched chicks (p < .0001) (Figure ). No significant correlations could be detected between CORT and CPN, AGP, CORT, and Hsp70 (Table ). However, there was a significant positive correlation between CPN and Hsp70.
Figure 1. Changes in serum levels of corticosterone (CORT) at days 14 (ED 14), 16 (ED 16) and 18 (ED 18) of incubation, and newly hatched broiler chicks. Sampling group means (n = 16) with no common letters (a–c) differ at p < .05.
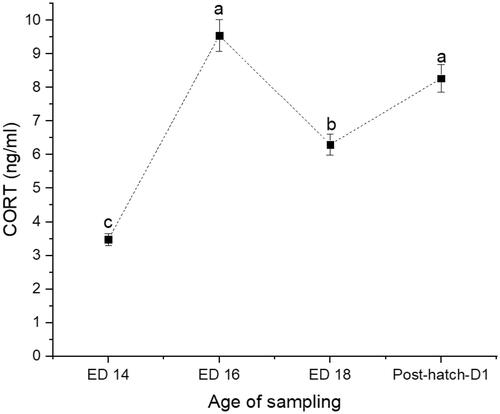
Figure 2. Changes in serum levels of ceruloplasmin (CPN) at days 14 (ED 14), 16 (ED 16) and 18 (ED 18) of incubation, and newly hatched broiler chicks. Sampling group means (n = 16) with no common letters (a–c) differ at p < .05.
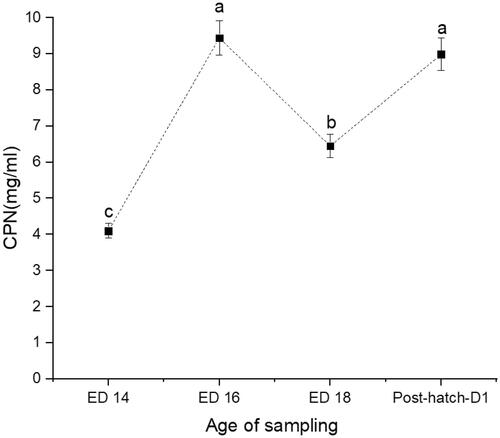
Figure 3. Changes in serum levels of alpha-1-acid glycoprotein (AGP) at days 14 (ED 14), 16 (ED 16) and 18 (ED 18) of incubation, and newly hatched broiler chicks. Sampling group means (n = 16) with no common letters (a–c) differ at p < .05.
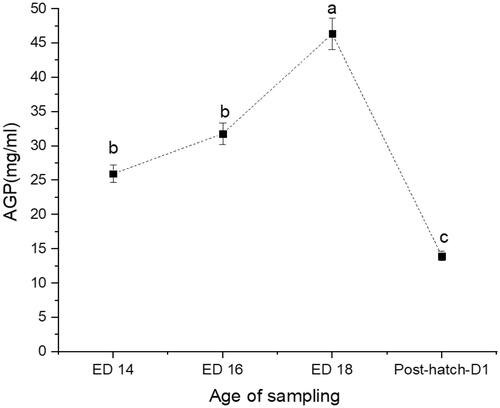
Figure 4. Changes in serum levels of heat shock protein 70 (Hsp70 at days 14 (ED 14), 16 (ED 16) and 18 (ED 18) of incubation, and newly hatched broiler chicks. Sampling group means (n = 16) with no common letters (a–c) differ at p < .05.
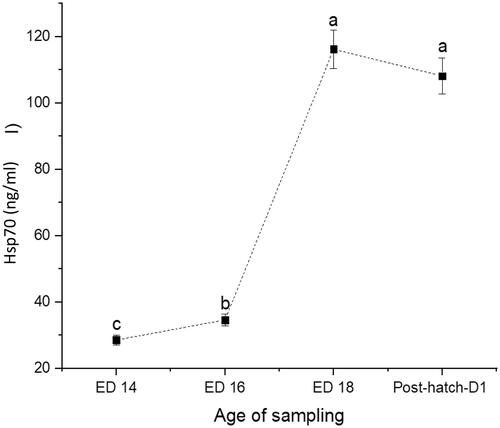
Table 1. Mean (±SEM) body weights of embryos at various stages and newly hatched chicks.
Table 2. Pearson’s correlation coefficients between CORT, CPN, AGP and Hsp70 in embryos and newly hatched chicks.
Discussion
Elevation in circulating corticosterone concentrations is a hallmark of stress in avian species (Blas Citation2015). One of the earliest studies on detecting corticosterone in chicken embryos was by Pedernera (Citation1972). Based on in vitro studies, the author reported that the adrenals of chick embryos could secrete corticosterone from 8 d of incubation onward, and the adrenal glands from 5-day embryos can also secrete glucocorticoids but only if stimulated with exogenous adrenocorticotropic hormone (ACTH) (Pedernera Citation1972). In vivo work by Wise and Frye (Citation1973) showed detectable plasma corticosterone in chicken embryos at 10 d of incubation. The present data concur with those of Wise and Frye (Citation1973), and Scott et al. (Citation1981) that CORT was dramatically increased from ED14 to ED16. The significantly higher CORT noted on ED16 could be associated with the maturation and establishment of a functional adrenohyphopheseal adrenal axis (Woods et al. Citation1971; Wise and Frye Citation1973; Kalliecharan and Hall Citation1974, Citation1976). Working with hypophysectomised embryos, Woods et al. (Citation1971) concluded that the pituitary-adrenal axis was established at 13.5 d of incubation.
Previous work (Wise and Frye Citation1973; Kalliecharan and Hall Citation1974; Scott et al. Citation1981), more than four decades ago, showed that circulating levels of corticosterone in chicken embryos did not vary significantly from ED15 to ED20. On the contrary, we noted a significant decline in CORT from ED16 to ED18. There is no clear explanation for the phenomenon although in the current study we used a modern Cobb 500™ commercial broiler strain. It has been reported that genetic differences may influence the development and physiological status of poultry embryos (Tona et al. Citation2004; Nangsuay et al. Citation2016).
Scott et al. (Citation1981) reported that the basal plasma levels of corticosterone increased gradually during the second half of incubation and reached a peak around the time of hatching. In the present study, the newly hatched chicks were not subjected to ‘stressful’ procedures such as debeaking and vaccination. The noted elevated CORT in the newly hatched chicks could be attributed to the seemingly eventful experience of initial emergence (Scott et al. Citation1981). Jacobs et al. (Citation2017) showed that longer transit duration increased CORT in day-old chicks, and thus, it appears that newly hatched chicks were able to respond to stressful stimuli.
It is well established that Hsps are produced when cells are exposed to noxious stimuli (Vinoth et al. Citation2015). However, some Hsps have multiple housekeeping chaperone roles linked to the folding and intracellular transport of newly synthesised proteins and multiprotein complexes during normal embryonic development under non-stress conditions (Nagai et al. Citation2000; Voss et al. Citation2000). Thus, in the present study, it is possible that the dramatic elevation in Hsp70 from ED16 to ED18 is associated with the chaperoning activity which is required in several different embryonic pathways (Evans et al. Citation2005).
The present study is the first to report the baseline values and preliminary patterns of APPs during embryogenesis in broiler chickens, namely CPN and AGP. These values reflect the developing profile of APPs in maintaining homeostasis and metabolic activities in typical healthy embryonic broiler chickens. The functional roles of positive APPs included opsonisation and trapping of micro-organisms and their products, inactivating complement, in binding cellular remnants like nuclear fractions, in neutralising enzymes, scavenging free haemoglobin and radicals, and in modulating the host’s immune response (Gruys et al. Citation2005). Thus, APPs have a significant role in protecting the developing embryos from infections and noxious stimuli. It is interesting to note that CPN but not AGP showed a similar pattern with CORT at ED14, ED16 and ED18 and in the newly hatched chicks. Zulkifli et al. (Citation2014) noted dramatic elevation in AGP and CPN in broilers following four and seven days of exogenous corticosterone administration, respectively. Work in lactating and non-lactating rabbits showed that serum cortisol levels were not significantly related to circulating concentrations of APPs C-reactive protein and serum amyloid A (Argente et al. Citation2014). In the present study, there was no significant correlation between CORT, CPN, and AGP. Thus, it appears that corticosterone, unlike in juvenile broilers, is not the underlying cause of changes in CPN, and AGP in the embryos and newly-hatched chicks under ‘nonstress’ environment.
Increases in circulating levels of corticosterone and expression of heat shock proteins when chick embryos experienced stress have been reported (Henriksen et al. Citation2011). In the present study, the embryos and newly hatched chicks were not exposed to stress and we noted a lack of significant correlation between serum CORT and Hsp70 concentrations. It is unknown whether CORT may modulate APP reaction in stressed chicken embryos. Serum concentrations of APPs have been used as non-specific biomarkers of physiological stress response in juvenile chickens (Shakeri et al. Citation2014; Zulkifli et al. Citation2014; Najafi et al. Citation2015, Citation2016, Citation2018). Although the noted AGP and CPN may reflect normal immunological events that take place during the perinatal and neonatal stages, interactions between both normal physiological and stress responses may have accounted for the changes in the levels of the proteins. It is interesting to note a significant positive correlation between CPN and Hsp70 in ‘nonstressed’ embryos and newly hatched chicks. Hence, there is a possibility that CPN and Hsp70 are similar in terms of the stimuli for their production and the kinetics of their synthesis and bioavailability. Najafi et al. (Citation2018) reported that stress attributed to feeding deprivation without a concurrent increase in CORT had a negligible effect on serum levels of APPs and brain Hsp70 expression. Based on the findings of Najafi et al. (Citation2018), it can be concluded that corticosterone is the modulator for both APPs and Hsp70 responses in stressed chickens. Work in hypertensive human patients suggested a significant positive correlation between circulating Hsp70 gene expression values and c-reactive protein (an acute-phase protein) (Srivastava et al. Citation2016).
Conclusions
This study shows, for the first time, the CPN and AGP in the serum of embryonic chicks and newly hatched chicks. The CPN and AGP changed with the age of the embryos. The physiological relevance of circulating APPs and their interactions with CORT, and Hsp70 in poultry embryos and neonates have yet to be elucidated and further studies monitoring these analytes in stressful conditions are undoubtedly required. However, in light of the present findings, it seems reasonable to propose that APPs are of physiological relevance for the developing embryos due to their unspecific antimicrobial effects (Murata et al. Citation2004) and association with restoring homeostasis (Cray et al. Citation2009). As in juvenile and adult chickens, APPs are potential biomarkers for physiological and health status in embryos and newly hatched chicks. These propositions have yet to be proved, yet these findings are intriguing.
Disclosure statement
No potential conflict of interest was reported by the author(s).
Data availability statement
The authors confirm that the data supporting the findings of this study are available within the article.
Additional information
Funding
References
- Al-Aqil A, Zulkifli I. 2009. Changes in heat shock protein 70 expression and blood characteristics in transported broiler chickens as affected by housing and early age feed restriction. Poult Sci. 88(7):1358–1364.
- Andrewartha SJ, Tazawa H, Burggren WW. 2011. Embryonic control of heart rate: examining developmental patterns and temperature and oxygenation influences using embryonic avian models. Respir Physiol Neurobiol. 178(1):84–96.
- Argente MJ, García M, de la L, Birlanga V, Muelas R. 2014. Relationship between cortisol and acute phase protein concentrations in female rabbits. Vet J. 202(1):172–175.
- Blas J. 2015. Stress in birds. In: Scanes CG, editor. Sturkie’s avian physiology. 6th ed. London, UK: Academic Press; p. 769–810.
- Ceciliani F, Pocacqua V. 2007. The acute phase protein alpha1-acid glycoprotein: a model for altered glycosylation during diseases. CPPS. 8(1):91–108.
- Cray C, Zaias J, Altman NH. 2009. Acute phase response in animals: a review. Comp Med. 59(6):517–526.
- Evans TG, Yamamoto Y, Jeffery WR, Krone PH. 2005. Zebrafish Hsp70 is required for embryonic lens formation. Cell Stress Chaperones. 10(1):66–78.
- Fournier T, Medjoubi NN, Porquet D. 2000. Alpha-1-acid glycoprotein. Biochimica et Biophysica Acta (BBA) – Biomembranes. 1482(1–2):157–171.
- Givisiez PEN, Da Silva MM, Mazzi CM, Ferro MIT, Ferro JA, Gonzales E, Macari M. 2001. Heat or cold chronic stress affects organ weights and Hsp70 levels in chicken embryos. Can J Anim Sci. 81(1):83–87. 049
- Gomez-Laguna J, Salguero JF, Pallarés JF, Rodríguez-Gómez MI, Barranco I, Carrasco L. 2011. Acute phase proteins as biomarkers in animal health and welfare. Acute phase proteins as early non-specific biomarkers of human and veterinary diseases.
- González FHD, Tecles F, Martínez-Subiela S, Tvarijonaviciute A, Soler L, Cerón JJ. 2008. Acute phase protein response in goats. J Vet Diagn Invest. 20(5):580–584.
- Gruys E, Toussaint MJM, Niewold TA, Koopmans SJ. 2005. Acute-phase reaction and acute-phase proteins. J Zhejiang Univ Sci B. 6(11):1045–1056.
- Henriksen R, Rettenbacher S, Groothuis TGG. 2011. Prenatal stress in birds: pathways, effects, function and perspectives. Neurosci Biobehav Rev. 35(7):1484–1501.
- Itoh H, Tamura K, Izumi M, Motoi Y, Funayama Y. 1993. Characterization of serum alpha 1-acid glycoprotein in fetal and newborn calves during development. Am J Vet Res. 544:591–595.
- Jacobs L, Delezie E, Duchateau L, Goethals K, Ampe B, Buyse J, Tuyttens FAM. 2017. Impact of transportation duration on stress responses in day-old chicks from young and old breeders. Res Vet Sci. 112:172–176.
- Jain S, Gautam V, Naseem S. 2011. Acute-phase proteins: as diagnostic tool. J Pharm Bioallied Sci. 3(1):118–127.
- Jezierska B, Ługowska K, Witeska M. 2009. The effects of heavy metals on embryonic development of fish (a review). Fish Physiol Biochem. 35(4):625–640.
- Kalliecharan R, Hall BK. 1974. A developmental study of the levels of progesterone, corticosterone, cortisol, and cortisone circulating in plasma of chick embryos. Gen Comp Endocrinol. 24(4):364–372.
- Kalliecharan R, Hall BK. 1976. A developmental study of progesterone, corticosterone, cortisol, and cortisone in the adrenal gland of the embryonic chick. Gen Comp Endocrinol. 30(4):404–409.
- Kregel KC. 2002. Heat shock proteins: modifying factors in physiological stress responses and acquired thermotolerance. J Appl Physiol. 92(5):2177–2186.
- Leandro NS, Gonzales E, Ferro JA, Ferro MI, Givisiez PE, Macari M. 2004. Expression of heat shock protein in broiler embryo tissues after acute cold or heat stress. Mol Reprod Dev. 67(2):172–177.
- Shakeri M, Zulkifli I, Soleimani AF, O’Reilly EL, Eckersall PD, Anna AA, Kumari S, Abdullah FFJ. 2014. Response to dietary supplementation of l-glutamine and l-glutamate in broiler chickens reared at different stocking densities under hot, humid tropical conditions. Poult Sci. 93(11):2700–2708.
- Murata H, Shimada N, Yoshioka M. 2004. Current research on acute-phase proteins in veterinary diagnosis: An overview. Vet J. 168(1):28–40.
- Nagai N, Hosokawa M, Itohara S, Adachi E, Matsushita T, Hosokawa N, Nagata K. 2000. Embryonic lethality of molecular chaperone HSP47 knockout mice is associated with defects in collagen biosynthesis. J Cell Biol. 150(6):1450–1499.
- Najafi P, Zulkifli I, Jajuli NA, Farjam AS, Ramiah SK, Amir AA, O’Reily E, Eckersall D. 2015. Environmental temperature and stocking density effects on acute phase proteins, heat shock protein 70, circulating corticosterone and performance in broiler chickens. Int J Biometeorol. 59(11):1577–1583.
- Najafi P, Zulkifli I, Soleimani AF. 2018. Inhibition of corticosterone synthesis and its effect on acute phase proteins, heat shock protein 70, and interleukin-6 in broiler chickens subjected to feed restriction. Poult Sci. 97(4):1441–1447.
- Najafi P, Zulkifli I, Soleimani AF, Goh YM. 2016. Acute-phase proteins response to feed deprivation in broiler chickens. Poultr Sci. 95(4):760–763.
- Nakai A, Morimoto RI. 1993. Characterization of a novel chicken heat shock transcription factor, heat shock factor 3, suggests a new regulatory pathway. Mol Cell Biol. 13(4):1983–1997.
- Nangsuay A, Meijerhof R, Van Den Anker I, Heetkamp MJW, Morita VDS, Kemp B, Van Den Brand H. 2016. Effects of breeder age, broiler strain, and eggshell temperature on development and physiological status of embryos and hatchlings. Poultr Sci. 95(7):1666–1679.
- O’Reilly EL, Eckersall PD. 2014. Acute-phase proteins: a review of their function, behaviour and measurement in chickens. World’s Poultr Sci J. 70(1):27–44.
- Patel BN, Dunn RJ, Jeong SY, Zhu Q, Julien J, David S. 2002. Ceruloplasmin regulates iron levels in the CNS and prevents free radical injury. J Neurosci. 22 (15):6578–6586.
- Pedernera EA. 1972. Adrenocorticotropic activity in vitro of the chick embryo pituitary gland. General Comparative Endocrinol. 19(3):589–590.
- SAS. 2005. SAS/STAT software, version 9.4. Cary (NC): SAS Inst. Inc.
- Scott TR, Johnson WA, Satterlee DG, Gildersleeve RP. 1981. Circulating levels of corticosterone in the serum of developing chick embryos and newly hatched chicks. Poult Sci. 60(6):1314–1320.
- Soleimani AF, Zulkifli I, Omar AR, Raha AR. 2011. Neonatal feed restriction modulates circulating levels of corticosterone and expression of glucocorticoid receptor and heat shock protein 70 in aged Japanese quail exposed to acute heat stress. Poult Sci. 90 (7):1427–1434.
- Srivastava K, Narang R, Bhatia J, Saluja D. 2016. Expression of heat shock protein 70 gene and its correlation with inflammatory markers in essential hypertension. PLOS One. 11(3):e0151060.
- Tona K, Onagbesan OM, Jego Y, Kamers B, Decuypere E, Bruggeman V. 2004. Comparison of embryo physiological parameters during incubation, chick quality, and growth performance of three lines of broiler breeders differing in genetic composition and growth rate. Poultr Sci. 83(3):507–513.
- Vinoth A, Thirunalasundari T, Tharian JA, Shanmugam M, Rajkumar U. 2015. Effect of thermal manipulation during embryogenesis on liver heat shock protein expression in chronic heat stressed colored broiler chickens. J Therm Biol. 53:162–171.
- Voss AK, Thomas T, Gruss P. 2000. Mice lacking HSP90β fail to develop a placental labyrinth. Development. 127(1):1–11.
- Wise PM, Frye BE. 1973. Functional development of the hypothalamo-hypophyseal-adrenal cortex axis in the chick embryo, Gallus domesticus . J Exp Zool. 185(3):277–292.
- Wong GK, Cavey MJ. 1992. Development of the liver in the chicken embryo. I. Hepatic cords and sinusoids. Anat Rec. 234(4):555–567.
- Woods JE, De Vries GW, Thommes RC. 1971. Ontogenesis of the pituitary-adrenal axis in the chick embryo. Gen Comp Endocrinol. 17(3):407–415.
- Zulkifli I, Najafi P, Nurfarahin AJ, Soleimani AF, Kumari S, Aryani AA, O'Reilly EL, Eckersall PD. 2014. Acute phase proteins, interleukin 6, and heat shock protein 70 in broiler chickens administered with corticosterone. Poult Sci. 93(12):3112–3118.