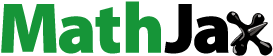
Abstract
Ruminants would suffer from gastric distension (bloat) when feeding on alfalfa (Medicago sativa L.; Alf), while tannins can relieve this problem. Thus, this study aimed to evaluate the effect of Alf mixed with paper mulberry (Broussonetia papyrifera) leaves (PML) or condensed tannins (CT) extracted from PML on in vitro fermentation performance. Seven experimental treatments based on the mass ratios of Alf and PML were set up: Alf, PML, 3A1P (Alf:PML = 3:1), 1A1P (Alf:PML = 1:1), 1A3P (Alf:PML = 1:3), A1P (Alf with 1% CT extracted from PML), A2P (Alf with 2% CT extracted from PML), and a blank. The results after fermentation were as follows. (1) The degradable dry matter (DDM) and degradable organic matter (DOM) contents increased simultaneously with the increase in the proportion of PML (p < .05). However, the DDM and DOM contents decreased with the increase in PML CT concentration. Moreover, mixing PML with ALF improved (p <.05) the concentrations of acetic (except in 3A1P, p > .05), propionic, and butyric acids compared to Alf alone. Adding CT improved the concentrations of propionic and butyric acids over the Alf treatment (p < .05). (2) The 1A1P, 1A3P, and PML treatments showed the maximum rate of gas production during fermentation when compared with the other treatments (p < .05). The asymptotic gas volumes of the treatments with added CT were less than the Alf treatment (p < .05). It can be concluded that a mixture of Alf and PML after in vitro fermentation for 96 h could improve the DDM and DOM contents, reduce gas production, and produce more short-chain fatty acids.
Adding paper mulberry leaves to Alfalfa could improve the degradable dry matter and degradable organic matter.
Adding paper mulberry leaves to Alfalfa could reduce gas production, and produce more short-chain fatty acids than Alfalfa.
Adding condensed tannin extracted from paper mulberry leaves to Alfalfa could reduce gas production and the maximum rate of substrate digestion, thereby preventing bloat.
Highlights
Introduction
Alfalfa (Medicago sativa L., Alf) is one of the most prevalent forage legumes grown worldwide. Known as ‘the king of forage’ (Ma et al. Citation2016), alfalfa is widely used for beef and dairy production because it is high in yield, palatable, and rich in nutrients (Phelan et al. Citation2015; Zhang et al. Citation2015; Kulkarni et al. Citation2018).
However, the consumption of Alf is associated with a few challenges. For example, ruminants feeding on large quantities of Alf in a short time are likely to suffer from gastric distension (bloat) due to the rapid digestion of Alf producing a lot of gas. When the gas mixes with soluble proteins from digested plant cells, it forms a stable frothy structure in the rumen (Makoni et al. Citation1994; Min et al. Citation2005), which is difficult to discharge and may even result in ruminant death. Therefore, ruminants are allowed to graze on forage legumes such as clover or Alf only as components of grass–legume mixtures to avoid the above-mentioned issue (Phelan et al. Citation2015). Alternatively, bloat may be prevented by adding tannins to animal feed. Many studies have suggested that tannins could resolve the problem of the degradation of soluble proteins (Li et al. Citation1996; Jonker and Yu Citation2016; Kapp-Bitter et al. Citation2020). Tannins are effective in inhibiting bloat in ruminants because they precipitate plant proteins in the rumen (Tabacco et al. Citation2006). When ruminants were fed on a moderate (2–5% DM) amount of tannin-containing feed, the amount of degradable protein in the rumen was reduced (Grainger et al. Citation2009; Jayanegara et al. Citation2012). In addition, the degradation rate of soluble proteins by rumen microorganisms was also reduced, which in turn reduced the gas release rate in the rumen (Alves et al. Citation2017). Low to moderate tannin concentrations can regulate the rate of organic matter (OM) degradation by protecting the OM from fast ruminal degradation while enhancing utilisation through the post-ruminal gut (Soltan et al. Citation2021). However, whether and how those effects occur depends on the tannins’ source, type, structure, and concentration (Huang et al. Citation2018).
At present, alfalfa availability in China is insufficient. Thus, there is a need to develop unconventional forage to meet the production demand. Paper mulberry (Broussonetia papyrifera, PM) is a deciduous tree belonging to the mulberry family that is widely distributed in most parts of China (Li et al. Citation2021). Tu et al. (Citation2009) reported that the crude protein (CP) content in paper mulberry leaves (PML) was 26.1% (air-dried basis) and that there was higher ether extract (EE) and calcium in PML than that found in Alf hay but lower neutral detergent fibre (NDF) and acid detergent fibre (ADF). Earlier research showed that the content of CP increased and the content of crude fibre (CF) decreased in PM after it was ensiled (Zhang et al. Citation2008). Thus, PML can improve ruminant diets when fed along with legumes and grasses due to its nutritive value. Recently, PM has been widely used as a high protein forage source for livestock (Lin Citation2019; Liu Citation2019). Si et al. (Citation2018) indicated that feeding Holstein cows with a total mixed ratio (TMR) that included different proportions of PM silage could significantly improve the immunological parameters and antioxidant biomarkers in serum indexes of dairy cows. However, Lin et al. (Citation2018) reported that the apparent digestibility of dry matter (DM), NDF, and EE decreased with increases in the proportion of crossbred PM silage in the diet. Li et al. (Citation2018) observed that adding hybrid PM silage instead of Alf silage to a TMR increased the proportions of lactic acid and NH3-N in the diet. Meanwhile, the PML cultivated in China contained more than 0.2 mg/g tannins on average (Zuo et al. Citation2018), a level that could protect the rumen from excessive fermentation. Thus, PML may be a potential supplement to regulate the degradation rate of Alf. There are few reports on the fermentation of Alf mixed with PML. Therefore, the objective of this study was to better understand the effect of Alf mixed with PML or condensed tannins (CT) extracted from PML on in vitro rumen fermentation.
Materials and methods
Forage collection and CT extraction
The Alf and PML forages used in this study were planted at the Zhuozhou Experimental Station (E115°86′, N39°48′) of China Agricultural University, Baoding, China. The annual average sunshine is 2 560 h, and the annual average rainfall is 399.3 mm; the daily average temperature is between 8 °C and 19 °C. The Alf seeds were planted in October in rows about 40 cm apart. The land area used in the experiment measured 4 × 4 m in each plot, and the seeding rate was 1 kg ha−1. The soil was watered when precipitation was insufficient. The Alf was mowed in the early flowering stage to a stubble height of about 5 cm. PM seeds were planted in April in rows about 25 cm apart and were grown under natural conditions. The PML were collected when plant height was about 100 cm.
The fresh Alf and PML samples were collected, cut into 0.5–1 cm pieces, freeze dried, crushed, and stored for later use. The samples were milled (Wiley mill, Thomas Scientific, Swedesboro, NJ, USA) to 1 mm before the experiment began.
CT was isolated from PML using the methods of Zhang et al. (Citation2021), and its concentration in PML samples was determined according to the method described by Grabber et al. (Citation2013).
Experimental design
The seven treatments consisted of different mass ratios of Alf and PML or PML CT: Alf only, 3A1P (Alf:PML = 3:1), 1A1P (Alf:PML = 1:1), 1A3P (Alf:PML = 1:3), PML only, A1P (Alf with 1% CT extracted from PML), and A2P (Alf with 2% CT extracted from PML). In this study, three runs with three replications in each run were conducted, and each replication included a blank that contained buffer and rumen fluid but no substrate.
Ruminal fluid and buffer medium preparation
The donor Angus cow handling was conducted according to Utah State University Institutional Animal Care and Use Committee protocol #2834. Ruminal fluid was collected approximately 4 h after a meal of Alf hay. The rumen fluid was collected from various locations within the rumen (Mauricio et al. Citation1999; Eun et al. Citation2007a) of one ruminally fistulated female Angus cow (24 months, 538 kg, Zhang et al. Citation2021). The rumen fluid completely filled a pre-warmed thermal flask for transportation to the laboratory. There it was strained through four layers of cheesecloth, placed under a constant flow of CO2 and held at 39 °C in a water bath (Theodorou et al. Citation1994; Eun et al. Citation2007b). The mean pH of the ruminal fluid was measured using a pH metre (HI991002, Hanna Instruments, Woonsocket, RI, USA), and it was found to be 5.76. After fermentation, the mean pH of all samples was 6.76, and the mean pH of blanks was 6.81.
The buffer medium was prepared according to the procedure described by Menke et al. (Citation1979).
In vitro fermentation measurements
Approximately 0.4 g of substrate sample was taken and placed in 125-mL glass serum bottles (223748, Wheaton, Boston, MA, USA). Forty millilitres of the buffer medium were dispensed into the serum bottles and flushed with CO2, and 20 mL of ruminal fluid was added immediately afterward. The bottles were sealed with a 20 mm butyl rubber stopper and an aluminium crimp cap. The blank consisted of the buffer and the ruminal fluid only. The incubation time was 96 h in an incubator (BK6160, THERMO, USA), at a temperature of 39 °C.
Head-space gas pressure readings were obtained using a USB output pressure transducer (PX409-015GUSBH, Omega Engineering, Inc., Stanford, CT, USA) at 1, 2, 4, 6, 8, 10, 12, 18, 24, 36, 48, 72, and 96 h (Theodorou et al. Citation1994). The transducer was connected to a disposable hypodermic needle (23 mm × 15 mm) inserted through the butyl rubber stopper; this enabled the reading to be displayed on a computer screen. After taking the reading, the transducer was unplugged from the needle, which remained inserted into the rubber stopper until the head-space gas pressure returned to the ambient pressure as indicated by a zero reading on the display unit. The bottles were swirled after each gas production reading and returned to their respective positions in the incubator. Pressure was measured at the same rate and order as the injection of rumen liquor. Fermentation was stopped by cooling the serum bottles to 4 °C in a walk-in freezer. Following a 96-h fermentation, undigested residues were collected in ANKOM in situ bags, rinsed thoroughly with distilled water, and dried to constant weight at 60 °C to obtain the dry mass.
Pressure was corrected for the quantity of substrate organic matter (OM) incubated, and gas released from the blanks were used to correct the gas volume of each replication. The gas volume was calculated using the Equation V = 5.3407*P, where V represents the head-space gas volume (mL), and P the pressure (psi) obtained using the transducer (Frutos et al. Citation2002). In addition, a single-phase model (EquationEq. (1)(1)
(1) ) for cumulative gas volume (G) was used to calculate the fermentation kinetics:
(1)
(1)
where A (mL/g OM) is the asymptotic maximum gas volume; B (h) is the incubation time (t) at which half the maximum amount of gas was formed, and C is a constant describing the sharpness of the switching characteristic of the cumulative gas curve (Groot et al. Citation1996).
The maximum rate of substrate digestion (RMax) and the time at which the maximum rate of substrate digestion occurred () were calculated from A, B, and C using the following equations (Groot et al. Citation1996):
(2)
(2)
(3)
(3)
Short-chain fatty acid determination
The fermentation fluid filtrates were collected with ANKOM in situ bags in order to measure the content of the short-chain fatty acids (SCFA) after fermentation had stopped. Fermented fluid filtrates were homogenised by adding 0.1 mL to 0.9 mL deionised water (Ward et al. Citation2017), and the solution was centrifuged at 10 000 r/min for 20 min to determined SCFA (acetic acid, propionic acid, n-butyric acid, i-butyric acid, i-valeric acid, n-valeric acid, and n-caproic acid). The supernatant was transferred to a gas chromatograph (GC, GC-2010, Shimadzu, Kyoto, Japan) vial for analysis. Based on a previous method (Anele et al. Citation2014), fatty acid analyses were carried out with a free fatty acid phase (FFAP) column (30 m × 0.53 mm × 1 μm). Hydrogen was used as the carrier gas at a column flow rate of 14.4 mL/min. The temperatures of the flame ionisation detector (FID) and injector were 350 °C and 220 °C, respectively. The injected sample volume was 1 µL under the split mode. The initial oven temperature of 60 °C was maintained for 1 min, raised to 260 °C at 17 °C/min, and finally held at 260 °C for 8 min. The run time for each analysis was 20.76 min.
Chemical analyses
Samples were analysed according to the standard methods of the Association of Official Analytical Chemists (AOAC Citation1995) for DM, CP, ash, and EE. The water-soluble carbohydrate (WSC) was determined by the method of Chen et al. (Citation2017). The NDF and ADF were determined according to Van Soest et al. (Citation1991). The OM was defined as DM minus ash (Table ).
Table 1. Content of chemical composition in each group (DM basis).
The degradability of DM was determined by drying the residues at 60 °C for 48 h, and that of OM was determined by ashing the dried residues at 550 °C for 6 h.
Statistical analysis
Cumulative fermentation gas kinetics parameters (A, B, C) were estimated using nonlinear regression (Graphpad Prism 8) with a mixed model in which run was the random factor (three runs and three repetitions each run) and treatments with their interactions were the fixed effects. Pairwise differences among the least squares means of the kinetics parameters ( and RMax) were tested with the Tukey–Kramer multiplicity adjustment. The differences in the means of the treatments were obtained using a Tukey multiple range test by one-way ANOVA using SPSS 25.0. The data were expressed as mean and SEM, and the tests were considered significant at the level of p < .05.
Results
Effect of Alf mixed with PML or CT extracted from PML on digestibility
The degradable DM (DDM) and degradable OM (DOM) for each treatment are shown in Table . As the proportion of PML in treatments increased, DDM and DOM presented an increasing trend. PML had the highest DDM and DOM and differed from other treatments (p < .05). The treatments with added CT had less DDM (p > .05) and DOM (p < .05) compared with Alf, and the greater the amount of CT, the lower the DDM and DOM. The DDM and DOM of the Alf-PML mixture treatments were higher than for the Alf, A1P, and A2P treatments.
Table 2. Characteristics of forages following in vitro fermentation for 96 h (DM basis).
Effect of Alf mixed with PML or CT extracted from PML on SCFA production
The SCFA concentrations of the different treatments following in vitro fermentation for 96 h are shown in Table . The SCFA concentrations of PML were significantly higher than those of treatments that included Alf (p < .05). The acetic acid concentrations of 1A1P and 1A3P were higher than those of Alf and 3A1P (p < .05), but there was no significant difference in the acetic acid concentration among the Alf, 3A1P, A1P, and A2P treatments (p > .05). The concentration of acetic acid increased with increase in the PML concentration (p < .05). The concentrations of propionic and butyric acids showed an increasing trend with increase in the proportion of PML (p < .05), which in A1P and A2P treatments were also higher than in Alf (p < .05). The concentrations of isobutyric and valeric acids in PML were higher than those of the other treatments (p < .05). The concentration of isovaleric acid of Alf did not differ from those of 3A1P, 1A1P, or 1A3P. However, the concentrations of isovaleric acid in A1P and A2P were significantly lower than in Alf (p < .05). No significant difference was found in the concentration of caproic acid or the ratio of acetic to propionic acids (A/P) among treatments (p > .05).
Table 3. Characteristics of short-chain fatty acids following in vitro fermentation for 96 h (mmol/L).
Effect of alf mixed with PML or CT extracted from PML on fermentation performance in vitro
In this study, the 1A1P and the PML treatments exhibited the highest RMax value and did not differ from that exhibited by the A13P treatment (p > .05); however, it significantly differed from that exhibited by Alf (p < .05). The RMax values of the A1P and A2P treatments were lower than that of Alf. Except for 1A1P, the values of the other treatments were higher than that of Alf (p < .05). Furthermore, there was a delay in
proportional to the increase in the concentration of CT (Table ).
Table 4. RMax and values of forages as a function of OM, following in vitro fermentation.
The asymptotic gas volume (parameter A) of Alf was higher than that of the other treatments (p < .05). Parameter A was greater when isolated PML CT was added to Alf than when Alf was mixed with PML (p > .05). Parameter B was also highest for the A1P and A2P treatments and differed from those for the other treatments (p < .05). The parameter showed a decreasing trend with the increase in the proportion of PML. Parameter C was the least for Alf and did not differ from the 1A1P, A1P, and A2P treatments (p > .05). There was also no significant difference in the values of parameter C among the 3A1P, 1A3P, PML, A1P, and A2P treatments (Table ).
Table 5. Kinetics of cumulative gas production from in vitro fermentation of forage.
Figure shows the trend of cumulative gas production (mL/g OM). All treatments showed a similar trend of a rapid rise within 15 h and a subsequent change to a more gradual rise. Alf had the highest cumulative gas production (Parameter A) and the Alf plus PML CT treatments were greater than PML or treatments where PML was added to Alf.
Discussion
Effect of alf mixed with PML or CT extracted from PML on digestibility
The digestion of feed by rumen microorganisms is affected by the chemical composition of the feed. The concentration of NDF is the key factor that limits the digestibility and therefore the intake of forage in ruminants (Mertens Citation1987, Citation2009). In this study, the NDF of Alf was greater than in PML, resulting in DDM and DOM increasing with the proportion of PML. High WSC indicates a high amount of fermentable substrates (Seo et al. Citation2013), and the degradability and fermentation of substrates are correlated with available carbohydrates and protein for microbial growth (Salama et al. Citation2020). Thus, the PML-containing treatments had higher DDM and DOM, demonstrating the synergy of mixtures compared with Alf and supporting the observation that mixing Alf and PML enhanced digestibility.
The present study indicates that digestibility could be increased when Alf is mixed with other crops (Chen et al. Citation2017; Bai et al. Citation2018; Chen et al. Citation2019). There are some studies suggesting that CT could link with plant proteins and fibre (Tabacco et al. Citation2006; Jayanegara et al. Citation2012), thereby reducing their rumen digestibility. In this study, the DOM of A1P and A2P treatments were less than in the Alf treatment, decreasing as the concentration of CT increased. As the ratio of PML to ALF increased, the content of CT also increased, but the DDM and DOM in the group of treatments where Alf was mixed with PML increased rather than decreasing. These differences between the group of treatments where PML was added compared with the group where isolated PML CT was added may result from the isolated PML CT combining effectively with the protein and fibre in Alf, causing fibre and protein to become less available for microbial digestion.
Effect of Alf mixed with PML or CT extracted from PML on SCFA production
SCFA are the most important end-products of carbohydrate digestion in ruminants (Scott et al. Citation2008) since SCFA provide energy for the various activities in animals. (Blümmel et al. Citation1997) and Sutton (Citation1985) reported that SCFA could provide more than 50% of the total degradable energy. It may be concluded that the SCFA concentration plays an important role in rumen fermentation. Other studies have suggested that the concentration of SCFA is positively correlated with the amount of substrate fermentation in the rumen (McDonald et al. Citation2011; Chen et al. Citation2019). In this study, we observed that with the increase in the proportion of PML, the DDM and DOM also increased, which in turn resulted in an increase in the concentration of SCFA. The ratio of CP to CF (P/F) is an important factor affecting the content of SCFA (Corley and Murphy Citation2004; Wang Citation2012). The greater the proportion of PML, the higher the value of P/F in the present study, resulting in higher production of SCFA.
It has been demonstrated that SCFA production is related to microbial abundance and activity for the degradation of fermentable carbohydrates (Hess et al. Citation2011) and plant fibre (Koike et al. Citation2004; Shinkai et al. Citation2010). We studied different combination ratios of paper mulberry and alfalfa on rumen bacterial diversity in Angus cows, and the results suggested that Alf mixed with PML could increase the relative abundance and diversity of bacteria such as Spirochaetes and Proteobacteria (Chen et al. Citation2020). The group of treatments where Alf was mixed with PML had more total SCFA than Alf alone, in accord with the above-cited research studies. However, SCFA concentration in Alf with added CT extracted from PML was not significantly different from Alf, suggesting that the increase in SCFA with added PML was due to greater plant nutritive value rather than the CT found in PML.
Effect of Alf mixed with PML or CT extracted from PML on fermentation performance in vitro
The asymptotic gas volume (parameter A) is the projected cumulative gas production at the end of fermentation calculated by the analysis of cumulative gas as a function of time. Gases produced in the rumen are mainly carbon dioxide and methane. It has been found that plant fibre accounts for more than 60% of gas production emissions (Niu Citation2014). In this study, the content of plant fibre in Alf was far greater than that in other treatments; this was the main reason why the gas production of Alf was higher than in other treatments. Alf had the highest NDF and contained no tannin, resulting in Parameter A of Alf being significantly higher than in other treatments. Here, treatments with more CT produced less gas. Furthermore, some relevant studies have reported a negative correlation between gas production and substrate CP content (Ndlovu and Nherera Citation1997; Cone and Van Gelder Citation1999), similar to the findings of this study. The treatments containing PML had both lower gas production and a higher concentration of CP.
In this study, the time needed to reach one-half asymptotic gas volume (parameter B) ranged from 6.14 h (PML) to 8.41 h (A1P). With the increasing proportion of PML, parameter B showed a downward trend; the value of parameter B of A1P and A2P was significantly higher than that of Alf, which was related to the maximum rate (RMax) of fermentation. The value of RMax showed an increase with the proportion of PML. However, the results for A1P and A2P were opposite. Tannins inhibit the complexation of microorganisms and feed proteins with the ions required by rumen microorganisms; concentrated tannins inhibit the bacteria that decompose proteins (Jones et al. Citation1994). Moreover, tannins may form complexes with minerals and polysaccharides, thereby retarding their fermentation (Jansman Citation1993; Smith et al. Citation2005). However, as the PML to Alf ratio increased, mixture treatments had more water-soluble carbohydrate and protein substrates for rumen microbes, and this could offset the negative effect of CT. The length of time from the beginning of fermentation until the maximum rate of substrate digestion occurred was the shortest for Alf, the only treatment without CT. As mentioned above, tannins could negatively affect the rumen bacteria content and activity, so that the treatments containing CT take longer to arrive at RMax. When alfalfa is mixed with PML in appropriate proportions, the greater DOM and DDM values appear to be more beneficial to ruminant production than the more rapid increase in fermentation rate found for a single non-tannin forage such as alfalfa.
As suggested by the Groot et al. (Citation1996) model parameter C (a constant describing the sharpness of the curves) describes the sigmoidal shape of cumulative gas production curves as the time to maximum rate changes. Parameter C in this study increased with similar to the observations made by Zhang et al. (Citation2021), indicating greater lag times with the added PML CT or added PML treatments before exponential increase in fermentation gas production occurs.
Conclusions
Alf mixed with PML had higher DDM and DOM, lower gas production, and more SCFA than Alf alone. Adding PML, which had greater protein and WSC than Alf, appeared to increase digestibility of the feed, whereas adding PML CT reduced gas production and RMax of gas production in the rumen compared with Alf. In conclusion, both PML and CT from PML could prevent gastric distension (bloat) from the rapid digestion of Alf. PML should be added to ruminant rations.
Ethical approval
Animal handling was conducted under Utah State University Institutional Animal Care and Use Committee protocol #2834.
Disclosure statement
The authors declare that the research was conducted in the absence of any commercial or financial relationships.
Data availability statement
The data used to support the findings of this study are obtainable from the corresponding author upon request.
Additional information
Funding
References
- Alves TP, Dall-Orsoletta AC, Ribeiro-Filho HMN. 2017. The effects of supplementing Acacia mearnsii tannin extract on dairy cow dry matter intake, milk production, and methane emission in a tropical pasture. Trop Anim Health Prod. 49(8):1663–1668.
- Anele UY, Refat B, Swift ML, He ZX, Zhao YL, McAllister TA, Yang WZ. 2014. Effects of bulk density, precision processing and processing index on in vitro ruminal fermentation of dry-rolled barley grain. Anim Feed Sci Technol. 195:28–37.
- AOAC. 1995. Official methods of analysis. 16th ed. Arlington, VA, USA Association of Official Analytical Chemists.
- Bai S, Cao ZJ, Cao BB, Yang HJ, Li SL, Liu JX. 2018. Effects of different forage combinations in total mixed rations on in vitro gas production kinetics, ruminal and milk fatty acid profiles of lactating cows. Anim Sci J. 89(9):1261–1270.
- Blümmel M, Makkar HPS, Becker K. 1997. In vitro gas production: a technique revisited. J Anim Physiol Anim Nutr. 77(1-5):24–34.
- Chen L, Dong Z, Li J, Shao T. 2019. Ensiling characteristics, in vitro rumen fermentation, microbial communities and aerobic stability of low-dry matter silages produced with sweet sorghum and alfalfa mixtures. J Sci Food Agric. 99(5):2140–2145.
- Chen L, Guo G, Yuan XJ, Zhang J, Wen AY, Sun XH, Shao T. 2017. Effect of ensiling whole crop oat with lucerne in different ratios on fermentation quality, aerobic stability and in vitro digestibility on the Tibetan plateau. J Anim Physiol Anim Nutr. 101(5):e144–e153.
- Chen LJ, Li DJ, Zhang YH. 2020. Effects of different combination ratios of paper mulberry and alfalfa on rumen bacterial diversity in Angus cows. Pratacultural Science. 37:1579–1587.
- Chen L, Li J, Dong Z, Shao T. 2019. Effects of lactic acid bacteria inoculants and fibrolytic enzymes on the fermentation quality, in vitro degradability, ruminal variables and microbial communities of high‐moisture alfalfa silage. Grassl Sci. 65(4):216–225.
- Cone JW, Van Gelder AH. 1999. Influence of protein fermentation on gas production profiles. Anim Feed Sci Technol. 76(3-4):251–264.
- Corley RN, Murphy MR. 2004. An in vitro technique for measuring the production rate of volatile fatty acids in the rumen under dynamic conditions. Small Ruminant Res. 54(3):219–225.
- Eun JS, Beauchemin KA, Schulze H. 2007a. Use of exogenous fibrolytic enzymes to enhance in vitro fermentation of alfalfa hay and corn silage. J Dairy Sci. 90(3):1440–1451.
- Eun JS, Beauchemin KA, Schulze H. 2007b. Use of an in vitro fermentation bioassay to evaluate improvements in degradation of alfalfa hay due to exogenous feed enzymes. Anim Feed Sci Technol. 135(3–4):315–328.
- Frutos P, Hervás G, Ramos G, Giraldez FJ, Mantecon AR. 2002. Condensed tannin content of several shrub species from a mountain area in northern Spain, and its relationship to various indicators of nutritive value. Appl Environ Microbiol. 95:215–226.
- Grabber JH, Zeller WE, Mueller HI. 2013. Acetone enhances the direct analysis of procyanidin- and prodelphinidin-based condensed tannins in lotus species by the butanol-HCl-iron assay. J Agric Food Chem. 61(11):2669–2678.
- Grainger C, Clarke T, Auldist MJ, Beauchemin KA, McGinn SM, Waghorn GC, Eckard RJ. 2009. Potential use of Acacia mearnsii condensed tannins to reduce methane emissions and nitrogen excretion from grazing dairy cows. Can J Anim Sci. 89(2):241–251.
- Groot JCJ, Cone JW, Williams BA, Debersaques FMA, Lantinga EA. 1996. Multiphasic analysis of gas production kinetics for in vitro fermentation of ruminant feeds. Anim Feed Sci Technol. 64(1):77–89.
- Hess M, Sczyrba A, Egan R, Kim T-W, Chokhawala H, Schroth G, Luo S, Clark DS, Chen F, Zhang T, et al. 2011. Metagenomic discovery of biomass-degrading genes and genomes from cow rumen. Science. 331(6016):463–467.
- Huang Q, Liu X, Zhao G, Hu T, Wang Y. 2018. Potential and challenges of tannins as an alternative to in-feed antibiotics for farm animal production. Anim Nutr. 4(2):137–150.
- Jansman AJM. 1993. Tannins in feedstuffs for simple-stomached animals. Nutr Res Rev. 6(1):209–236.
- Jayanegara A, Leiber F, Kreuzer M. 2012. Meta-analysis of the relationship between dietary tannin level and methane formation in ruminants from in vivo and in vitro experiments. J Anim Physiol Anim Nutr (Berl)). 96(3):365–375.
- Jones GA, McAllister TA, Muir AD, Cheng KJ. 1994. Effects of Sainfoin (Onobrychis viciifolia Scop.) condensed tannins on growth and proteolysis by four strains of ruminal bacteria. Appl Environ Microbiol. 60(4):1374–1378.
- Jonker A, Yu P. 2016. The role of proanthocyanidins complex in structure and nutrition interaction in alfalfa forage. IJMS. 17(5):793.
- Kapp-Bitter AN, Dickhoefer U, Suglo E, Baumgartner L, Kreuzer M, Leiber F. 2020. Graded supplementation of chestnut tannins to dairy cows fed protein-rich spring pasture: effects on indicators of protein utilization. J Anim Feed Sci. 29(2):97–104.
- Koike S, Pan J, Suzuki T, Takano T, Oshima C, Kobayashi Y, Tanaka K. 2004. Ruminal distribution of the cellulolytic bacterium Fibrobacter succinogenes in relation to its phylogenetic grouping. Anim Sci J. 75(5):417–422.
- Kulkarni KP, Tayade R, Asekova S, Song JT, Shannon JG, Lee JD. 2018. Harnessing the potential of forage legumes, alfalfa, soybean, and cowpea for sustainable agriculture and global food security. Front Plant Sci. 9:1314.
- Li RR, Jiang D, Tian PJ, Zhang XY, Xu CC. 2018. Effect on fermentation characteristics in TMR by replacing alfalfa ensilage with Broussonetia papyrifera. Prataculture Anim Husb. 000:58–60.
- Li RR, Zheng ML, Jiang D, Tian PJ, Zheng MH, Xu CC. 2021. Replacing alfalfa with paper mulberry in total mixed ration silages: effects on ensiling characteristics, protein degradation, and in vitro digestibility. Animals. 11(5):1273.
- Li YG, Tanner G, Larkin P. 1996. The DMACA-HCl protocol and the threshold proanthocyanidin content for bloat safety in forage legumes. J Sci Food Agric. 70(1):89–101.
- Lin M, Zheng A, Liu Y, Liu X, Ma X, Qiang Z. 2018. Effect of silage crossbred Broussonetia papyrifera as protein feed replacer on manure discharge and apparent digestibility in mutton sheep. China Herbivore Science. 38:33–35.
- Lin YL. 2019. Effect of different processing methods on nutritive value of Broussonetia. Changchun: Jilin Agricultural University.
- Liu KL. 2019. Effects of different water content and additives on quality of hybrid paper mulberry silage. Jinzhong: Shanxi Agricultural University.
- Ma QG, Li T, Wei RR, Liu WM, Sang ZP, Song ZW. 2016. Characterization of chalcones from Medicago sativa L. and their hypolipidemic and antiangiogenic activities. J Agric Food Chem. 64(43):8138–8145.
- Makoni NF, Shelford JA, Fisher LJ. 1994. Initial rates of degradation of protein fractions from fresh, wilted, and ensiled alfalfa. J Dairy Sci. 77(6):1598–1603.
- Mauricio RM, Mould FL, Dhanoa MS, Owen E, Channa KS, Theodorou MK. 1999. A semi-automated in vitro gas production technique for ruminant feedstuff evaluation. Anim Feed Sci Technol. 79(4):321–330.
- McDonald P, Edwards RA, Greenhalgh JFD, Morgan CA, Sinclair LA, Wilkinson RG. 2011. Animal nutrition. 7th ed. Harlow, UK: Prentice Hall/Pearson.
- Menke KH, Raab L, Salewski A, Steingass H, Fritz D, Schneider W. 1979. The estimation of the digestibility and metabolizable energy content of ruminant feedingstuffs from the gas production when they are incubated with rumen liquor in vitro. J Agric Sci. 93(1):217–222.
- Mertens DR. 1987. Predicting intake and digestibility using mathematical models of ruminal function. J Anim Sci. 64(5):1548–1558.
- Mertens DR. 2009. Maximizing forage use by dairy cows. In: WCDS Adv Dairy Technol. 21:303–319.
- Min BR, Attwood GT, McNabb WC, Molan AL, Barry TN. 2005. The effect of condensed tannins from Lotus corniculatus on the proteolytic activities and growth of rumen bacteria. Anim Feed Sci Technol. 121(1–2):45–58.
- Ndlovu LR, Nherera FV. 1997. Chemical composition and relationship to in vitro gas production of Zimbabwean browsable in digenous tree species. Anim Feed Sci Technol. 69(1–3):121–129.
- Niu W. 2014. Research progress on the production of methane in ruminants and methane inhibitors. Anim Husb Feed Sci. 35:43–45.
- Phelan P, Moloney AP, McGeough EJ, Humphreys J, Bertilsson J, Riordan EG, Kiely P. 2015. Forage legumes for grazing and conserving in ruminant production systems. Crit Rev Plant Sci. 34(1–3):281–326.
- Salama HSA, El-Zaiat HM, Sallam SMA, Soltan YA. 2020. Agronomic and qualitative characterization of multi-cut berseem clover (Trifolium alexandrinum L.) cultivars. J Sci Food Agric. 100(10):3857–3865.
- Scott KP, Duncan SH, Flint HJ. 2008. Dietary fibre and the gut microbiota. Nutr Bull. 33(3):201–211.
- Seo JK, Kim MH, Yang JY, Kim HJ, Lee CH, Kim KH, Ha JK. 2013. Effects of synchronicity of carbohydrate and protein degradation on rumen fermentation characteristics and microbial protein synthesis. Asian-Australas J Anim Sci. 26(3):358–365.
- Shinkai T, Ueki T, Kobayashi Y. 2010. Detection and identification of rumen bacteria constituting a fibrolytic consortium dominated by Fibrobacter succinogenes. Anim Sci J. 81(1):72–79.
- Si B, Tao H, Zhang X, Guo J, Cui K, Tu Y, Diao Q. 2018. Effect of Broussonetia papyrifera L. (paper mulberry) silage on dry matter intake, milk composition, antioxidant capacity and milk fatty acid profile in dairy cows. Asian-Australas J Anim Sci. 31(8):1259–1266.
- Smith AH, Zoetendal E, Mackie RI. 2005. Bacterial mechanisms to overcome inhibitory effects of dietary tannins. Microb Ecol. 50(2):197–205.
- Soltan Y, Abdalla A, Berenchtein B. 2021. Replacing maize with low tannin sorghum grains: lamb growth performance, microbial protein synthesis and enteric methane production. Anim Prod ScI. 61(13):1348–1355.
- Sutton JD. 1985. The digestion and absorption of energy substrate in the lactating cow. J Dairy Sci. 68(12):3376–3393.
- Tabacco E, Borreani G, Crovetto GM, Galassi G, Colombo D, Cavallarin L. 2006. Effect of chestnut tannin on fermentation quality, proteolysis, and protein rumen degradability of alfalfa silage. J Dairy Sci. 89(12):4736–4746.
- Theodorou MK, Williams BA, Dhanoa MS, McAllan AB, France J. 1994. A simple gas production method using a pressure transducer to determine the fermentation kinetics of ruminant feeds. Anim Feed Sci Technol. 48(3–4):185–197.
- Tu Y, Diao QY, Zhang R, Yan GL, Xiong W. 2009. Analysis on the feed nutritive value of hybrid Broussonetia papyrifera leaf. Pratacultural Science. 26:136–139.
- Van Soest PJ, Robertson JB, Lewis BA. 1991. Symposium: Carbohydrate methodology, metabolism and nutritional implications in dairy cattle. Methods for dietary fiber, neutral detergent fiber and non-starch polysaccharides in relation to animal nutrition. J Dairy Sci. 74(10):3583–3597.
- Wang LI. 2012. The effect and influence factors of rumen volatile fatty acid. Chin J Anim Sci. 7:63–66.
- Ward RE, Benninghoff AD, Healy BJ, Li MH, Vagu B, Hintze KJ. 2017. Consumption of the total Western diet differentially affects the response to green tea in rodent models of chronic disease compared to the AIN93G diet. Mol Nutr Food Res. 61(4):1600720.
- Zhang J, Yin B, Xie Y, Li J, Yang Z, Zhang G. 2015. Legume-cereal intercropping improves forage yield, quality and degradability. PLOS One. 10(12):e0144813.
- Zhang YH, MacAdam JW, Villalba JJ, Dai X. 2021. In vitro digestibility of mountain-grown irrigated perennial legume, grass and forb forages is influenced by elevated non-fibrous carbohydrates and plant secondary compounds. J Sci Food Agric. 101(1):334–340.
- Zhang YM, Yu HS, Zhang YZ, Wang DS. 2008. Changes of nutritional content in the Broussonetia papyrifera leaf fermented feedstuff. Feed Industry. 29:54–55.
- Zuo X, Chen Z, Xie Q, Zhai S, Wang H, Zhong S, Zhu Y, Wang W, Yang L. 2018. Determination of nutrients and metabolic energy of geese in Broussonetia papyrifera leaves powder and Broussonetia papyrifera twig leaves powder from different areas. Chin J Anim Nutr. 30:2823–2830.