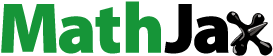
Abstract
The objective of this study was to evaluate nutrient digestibility, nitrogen balance, and purine derivative excretion from growing sheep fed on ryegrass silage (S) or ryegrass hay (H) cut at two maturity stages (21 and 35 d). In an in vivo trial, 32 Suffolk × Merino growing sheep (22 ± 2 kg) were used in a completely randomised design with eighth replications in a 2 × 2 factorial arrangement. Four diets were used containing 75% forage (S at 21 or 35 d and H at 21 or 35 d) and 25% concentrate. In an in vitro trial, a completely randomised design was used for gas production parameters. Contents of N and NDF were higher in H (30 and 674 g/kg DM) than in S (26 and 411 g/kg DM). Animals fed on S had higher digestibility (P < .008) of DM and OM (661 and 715 g/kg) than H at 35 d (557 and 596 g/kg). In vitro gas production was lower (P < .04) in H at 35 d. In vitro DM disappearance at 96 h was lower (P < .03) for H at 35 d. Excretion of allantoin (mmol/day) in urine was higher (P < .001) in S (7.91 and 3.95) than H (5.01 and 3.03) at 21 and 35 d respectively. Overall, compared to ryegrass hay, ryegrass silage cut at 21 d can be an advantageous feeding strategy for growing sheep without negative effects in nutrient intake, N-balance, and purine derivatives.
Contents of N and NDF were higher in hay than in silage form ryegrass.
Animals fed on silage had higher digestibility of DM and OM.
In vitro gas production was lower in hay cut at 35 days.
Highlights
Introduction
Forage conservation allows the preservation of nutritional characteristics of forages for prolonged periods and the main roughage feedstuffs for ruminants during drought periods are silages and hay (Penchev et al. Citation2016; Obraztsov et al. Citation2020; Kijak et al. Citation2017). However, in both forage preservation procedures, the nutrient composition is affected (Givens and Rulquin Citation2004; Dohme et al. Citation2007). In this regard, plant maturity is an important factor affecting the nutritional quality of preserved forages which decreases after flowering (Kallah et al. Citation1997).
The chemical composition of pasture herbage is a dynamic feature governed by the sum of physiological responses of plants and their interaction with growing conditions and management (Van Soest, Citation1994). As the chemical fractionation of CP is an option to quantify CP degradation in the rumen, a deeper description of CP fractions of high-quality herbage is required to increase N efficiency use (Kirchhof et al. Citation2010).
Harvesting delays can result in an increase in neutral detergent fibre (NDF) and acid detergent fibre (ADF) concentrations, and a decrease in crude protein (CP) and organic matter (OM) digestibility due to grass advanced growth (Jančík et al. Citation2011). On the other hand, silage fermentation quality can affect dry matter intake (Huhtanen et al. Citation2007) altering rumen fermentation and site of nutrient digestion. Silage quality is closely related to factors that influence the extent of digestion and passage rate including in vivo apparent digestibility, rumen degradability, and concentrations of fibre and nitrogen fractions (Steen et al. Citation1998).
The Cornell net carbohydrate and protein system (CNCPS) has been widely used to estimate nutrient composition and supply to animals fed with diverse feedstuffs and forages (Lanzas et al., Citation2008). Several studies have reported the effects of agricultural management on CP fractions in perennial ryegrass (Bryant et al. Citation2012; Loaiza et al. Citation2017). It has been reported that timing and harvest frequencies significantly affected plant maturity and consequently extractable CP contents and protein concentrate yields (Nielsen et al. Citation2021). However, there are few studies that evaluate the effect of the plant's maturity time (Nielsen et al. Citation2021).
Ryegrass (Lolium perenne L.) has the highest nutritive value of temperate grasses, with a metabolisable energy concentration of 12 MJ kg/DM (Turner et al. Citation2006). In finishing lambs, the use of perennial ryegrass has been shown to improve product performance, however, it is known that its stage of growth at harvesting will determine its nutritional quality (Fraser et al. Citation2004). Several studies have been carried out to demonstrate the nutritional quality of ryegrass in ruminant diets (McCormick et al. Citation1998; Chilibroste et al. Citation2000; Chaves et al. Citation2006). However, in Latinamerica, studies focussed on the most appropriate physiological stage at which forages should be cut to obtain the best nutritional characteristics for forage conservation either as hay or silage is scarce. Särkijärvi et al. (Citation2012), when evaluating Thimoty and Festuca grasses at different stages of maturity, observed that the interval of one week between cuts increases NDF content and decreases CP, affecting its intake and digestibility in sheep. Also, to our knowledge, no study has been done on the effects of different cutting times and conservation methods using concurrently in vitro and in vivo approaches in growing lambs. Thus, the objective of the present study was to determine chemical composition, N fractionation scheme, nutrient digestibility, N-balance, and purine derivative excretion from growing sheep fed on ryegrass silage (S) or ryegrass hay (H) cut at two maturity stages (21 and 35 d). Additionally, in this study, excretion of purine derivatives (allantoin and uric acid) in urine was an indirect method for estimating microbial crude protein (Balcells et al. Citation1992).
Material and methods
The present study was carried out in the Animal Science farm of the School of Veterinary Medicine and Animal Science of the Universidad Autónoma del Estado de México, Project UAEM 1748.
Forage collection and conservation
The soil from the study site had a clay soil texture composed of 62% sand, 10% silt, and 28% clay. The land had a slope of 2 to 6%. The dominant rocks were of volcanic and clastic types. The soils were pelic vertisol type and haplic phaeozem and were characterised by being very compact and clayish. Likewise, soil exhibited wide and deep cracks during drought season and showed a layer of tepetate between 10 and 50 cm of depth. Soils had 5.8 of pH, 23.8 cmol+kg−1 of the capacity of cationic interchange, 0.31% of total nitrogen, 7.56% of organic matter and 0.8 dS m−1 of electrical conductivity (Vaca García et al. Citation2018).
A 2000-square-meter plot of ryegrass (Lolium perenne L.) was used. This plot was established on the 28th of October 2018. It was irrigated with side roll irrigation every 20 days and fertilised with 44 kg N/ha (FIMSA and ACIFEX, 44%N) and KCl 60 kg/ha, 45 days prior to first harvest. The plot was divided into two sections (A and B), and one cut per section was made (New Holland tractor, 3-5 cm) at 21 days (18th November 2018 in plot A) with a height of 16 cm (vegetative stage: two leaves) and at 35 days (2nd December 2018 in plot B) with a height of 45 cm with a uniform plant maturity (pulling stage).
For silage making, fresh ryegrass was chopped from each cut (21 days and 35 days), placed and compacted in 12 hermetically sealed plastic bags (100 × 120 cm; n = 6 per cut), and no additives were used. Each bag was kept in a dark room at 25 °C. After 40 days, silages were opened and pH was determined (Conductronic model pH130). The rest of the plant material was used for haymaking. For this, fodder was sun-dried for 4 days in the field to reach 85% of DM and then stored.
In vivo trial: animals, housing and diets
Lamb management and all procedures in the present study were performed in accordance with the guidelines of the National Council for Animal Control and Experimentation (Olaiz Citation2015). This study was approved by the Ethics Committee on Animal Experimentation of the School of Veterinary Medicine and Animal Science of the Universidad Autonoma del Estado de Mexico (Protocol ID UAEM 1748).
Thirty-two Suffolk × Merino growing male lambs were used [22 ± 2 kg LW (average ± SD)]. Animals were assigned randomly to four treatments. Dietary treatments consisted of forage [ryegrass silage (S) or ryegrass hay (H) cut at 21 (S21 and H21) or 35 (S35 and H35) days of maturity] and concentrate (30% corn grain and 70% soybean meal) supplemented with vitamins and minerals (Multitec of Malta®; Celaya; Mexico). Lambs were fed at a feeding rate of 20 g/kg LW0.75 of concentrate and forage was supplied ad libitum (Table ). Forage and concentrates were manually mixed in each individual trough. Animals were kept in a roofed pen with individual metabolic cages (1.0 × 1.2 × 1.2 m) with slatted floor, hay bedding and had free access to water. Dietary treatments were divided into two meals and offered daily at 08h00 and 16h00.
Table 1. Chemical composition (g/kg DM) and protein fractions according to the Cornell Net Carbohydrate and Protein System (CNCPS) of ryegrass hay (H) and ryegrass silage (S) at two maturity stages (21 or 35 days) and a concentrate supplement (CS).
The study lasted 21 days in which 14 days were used for diet adaptation. Feed and orts during the last 7 days were recorded daily to determine nutrient intake. Individual daily samples of feed, orts, faeces and urine were taken at 08h00. Urine was collected in a sulphuric acid solution (10%; pH < 3). Only 10% of the total sample collected for faeces and urine was used for analysis. Individual body weights were measured at the end of the experimental period.
In vitro gas prodution and rumen fermentation parameters
Animal care and procedures for extraction of rumen inoculum were approved by the Ethics Committee for Animal Experimentation (Protocol ID UAEM 1748). Three rumen-fistulated sheep (Suffolk × Merino; LW 40 ± 1.0 kg) were used to obtain rumen fluid for in vitro fermentation incubations. Sheep were fed a maintenance diet based on alfalfa hay (Medicago sativa) and barley straw (Hordeum vulgare) (50:50) with 2% of a vitamin-mineral premix (Gold line Hitec-nutrition; Multitec Malta Cleyton; Celaya, Mexico). Animals were fed twice a day (08h00 and 16h00) and had free access to water.
In vitro gas production was determined using the technique proposed by Theodorou et al. (Citation1994). Hay and silage were weighed (0.800 g dry matter). Each sample was analysed in triplicate and incubated in glass flasks (125 mL) with 90 mL of buffer solution and 10 mL of ruminal fluid, and three incubation runs were performed. The buffer solution was prepared according to Menke and Steingass (Citation1988), where 0.800 g DM of each ingredient and each diet mixture were incubated in glass bottles of 125 ml. Details on buffer solution composition have been described previously (Vargas-Bello-Pérez et al. Citation2020)
To determine ruminal fermentation kinetics, three incubation runs of 96 h were carried out. In each run, three glass flasks per sample were used and three glass flasks as blanks (ruminal fluid and buffer only) were included. Rumen fluid samples were extracted and filtered in a triple layer of cheesecloth gauze and homogenised with CO2 for 5 min. Then, filtered samples were mixed and used as inoculum. Flasks were incubated in a water bath at 39 °C. Gas volume was recorded at 0, 3, 6, 9, 12, 24, 36, 48, 72, and 96 h of incubation using a Delta pressure transducer (Model 8804 HD; Padova, Italy). At 96 h of incubation, samples were filtered, washed under tap water, and dried (65 °C, 48 h) until analysis.
Silage and hay chemical composition
Silages were opened after 40 days and were used for lamb feeding. Three samples per bag were taken from each treatment (n = 36 samples) for DM determination (Haigh and Hopkins Citation1977). Feed and faeces samples were analysed for DM (using a forced-air oven at 60 °C for 48 h; AOAC method 934.01), ash (incineration at 550 °C for 3 h; AOAC method 942.05), nitrogen (Kjeldahl N; AOAC method 954.01) and ether extract (AOAC method 920.39) according to the AOAC (1997). Neutral detergent fibre (NDF, Van Soest et al. Citation1991), acid detergent fibre (ADF) and lignin (ADL) (AOAC, 1997; 973.18) analyses were performed using an ANKOM200 Fibre Analyser Unit (ANKOM Technology Corporation, Macedon, NY, USA). The neutral detergent fibre was assayed with alpha-amylase. Nitrogen fractions of herbage were determined in duplicate according to CNCPS (Sniffen et al. Citation1992). Buffer-soluble (BS), insoluble neutral detergent (NDIN), and acid detergent N (ADIN) were measured (Licitra et al. Citation1996). Protein fractions as a percentage of total protein were determined as follows (Sniffen et al. Citation1992): The fractions A, B1, and B2 were soluble in neutral detergent solution, whereas B3 was considered soluble in acid detergent solution. Fraction A was determined by subtracting trichloroacetic acid (TCA)-precipitated proteins from TN. Fraction B1 was determined by subtracting the borate phosphate buffer insoluble N from the TCA insoluble N. Fraction B2 was estimated by subtracting the neutral-detergent insoluble N (NDIN) from the borate-phosphate buffer insoluble N. Determination of NDIN was based on an NDF assay where 50 μl of heat-stable amylase was added to the sample, following the procedure described by Van Soest et al. (Citation1991). The insoluble protein fraction B3 was determined by subtracting the acid-detergent insoluble N (ADIN) from NDIN. Fraction C was equal to ADIN. Fresh silage samples were analysed for pH (Conductronic model pH130, Puebla, Mexico), and concentrations of lactic, acetic, and butyric acids according to Moon et al. (Citation1981).
Nitrogen and purine derivatives
Nitrogen was determined in urine samples (954.01; AOAC, 1997). Purine derivatives (PD; allantoin and uric acid) in urine were determined by high-performance liquid chromatography (Balcells et al. Citation1992). For that, urine samples were diluted 1:20 with the stock buffer solution of 0.1 M NH4H2PO4, filtered through a Millipore 0.45 pm filter (Millipore), and analysed directly.
Calculations and statistical analyses
Animal performance
Dry matter (DM) intake (kg/day and g/kg LW0.75), average daily gain (ADG, g/d) and feed conversion [(DM intake, (g/d)/AGD, (g/d)] were estimated. Intakes of organic matter (OM), crude protein (CP), and neutral detergent fibre (NDF) were estimated and expressed as g/kg LW0.75.
For performance and average daily gain (ADG), animals were weighed individually in the morning (07h30) before the first daily meal at the beginning and at the end of the experiment. Feed efficiency was determined as ADG divided by average DM intake, whereas ADG was calculated by subtracting final body weight from initial body weight and dividing the result by the number of days in the experimental period. These values were expressed as g/kg. The average daily gain was the average daily gain (g/kg LW0.75) of lambs during the 21 days.
Metabolisable protein (MP) intake (g/kg LW0.75) (Luo et al. Citation2004a) and metabolisable energy (ME) intake (kJ/kg LW0.75) were estimated according to Luo et al. (Citation2004b): MP intake (g/kg LW0.75/d) = 2.55 + [0.441 x ADG (g/kg LW0.75)] and ME intake (kJ/kg LW0.75/d) = 533 + [43.2 x ADG (g/kg LW0.75)].
In vitro gas production
In vitro gas production (GP) kinetics were determined by adjusting the model proposed by Krishnamoorthy et al. (Citation1991) using the following formula:
where b represents the total production of gas (ml gas/g initial DM); c the rate of degradation in relation to time (h−1); and t time (h−1). After in vitro incubation periods, dry matter disappearance (IVDMD, mg/100 mg) was determined. Samples were filtered and dried (48 h, 60 °C) and then organic matter disappearance (OMd, mg/100 mg) was determined (4 h 550 °C). Gas yield production was determined at 24 h (GY24), with the gas volume (ml g/g DM) produced after 24 h of incubation divided by the amount of IVDMD (g) calculated as follows: Gas production (GY24) = [(ml gas 24 h/g DM)/g IVDMD].
Relative gas production (RGP, ml gas 96 h/g IVDMD 96 h) was calculated according to González-Ronquillo et al. (Citation1998). Short-chain fatty acids concentration (SCFA) was calculated according to Getachew et al. (Citation2002) as: SCFA (mmol/200 mg DM) = 0.0222 GP − 0.00425. Where: GP is the 24 h net gas production (mL/200 mg DM).
Microbial biomass production (MP) was calculated according to Blümmel et al. (Citation1997) as: MP (mg/g DM) = mg IVDMD – (ml gas × 2.2 mg/mL). Where 2.2 mg/ml is a stoichiometric factor, which expresses mg of C, H, and O required for the SCFA gas associated with the production of one ml of gas (Blümmel et al. Citation1997).
Statistical analysis
In vivo and in vitro data were analysed using a completely randomised factorial arrangement 2 × 2, with the factors being the conservation method (n = 2), maturity stage (n = 2), and their interaction. The statistical model was:
where yij is the dependent variable, μ is the general average, Mi is the conservation method, Cj is harvest time, Mi × Cj is the interaction, and eijk the error term. The analyses were carried out by SAS statistical software (SAS Citation1999); the comparison of measures of each variable was carried out through the Tukey method (Steel et al. Citation1997). Mean differences were considered significant at P < .05. Standard errors of the mean were calculated from the residual mean square in the analysis of variance.
Results
Chemical composition
The chemical composition of ryegrass hay and ryegrass silage cut at different maturity stages is shown in Table . Crude protein content was higher in the hay, while acid detergent lignin was lower in silage. In both, hay and silage, NDF and ADF increased as ryegrass matured.
Intake and apparent in vivo digestibility
Hay-fed lambs had similar DM intake (P > .1) compared to those fed silage (Table ). Dry matter digestibility and OM digestibility were higher (P < .001) in S21. Neutral detergent fibre intake of forage and total diet DM was lower (P < .001) for S35 than the rest of the treatments. In S21, the average daily gain was higher (P = .009), metabolisable energy was higher (P = .010) and metabolisable protein intake was higher (P = .010).
Table 2. Growth performance, nutrient intake, and digestibility in lambs fed on ryegrass hay or ryegrass silage cut at 21 and 35 d.
Nitrogen balance and excretion of purine derivatives
Nitrogen intake was similar between treatments (Table ). Nitrogen excretion in faeces was higher for hay (H21 and H35) than for silage (S21 and S35). In urine, N excretion was higher in S21 (P < .001) than in H21 and in H35. Nitrogen balance was similar between treatments (P > .1).
Table 3. Nitrogen (N) balance (g/day) and concentration of purine derivatives (mmol/day) in urine in lambs fed on ryegrass hay or ryegrass silage cut at 21 and 35 d.
Allantoin (Table ) was the predominant purine derivative in urine, being higher (P < .001) in silage than in hay. S21 showed the highest excretion and H35 the lowest. Uric acid excretion was higher (P < .036) for silage S21 (P < .036) than for S35 and H35. Total purine derivative excretion was higher (P < .001) for silage than for hay, and S21 was higher (P < .001), with hay at H35 being the lowest purine derivative excretion.
In vitro gas production
Table showed differences (P < .05) for in vitro gas production (b), being lower in hay at 35 d compared with the rest of the treatments. The cutting stage affected GP being higher (P < .04) at 21 than at 35 days. In vitro IVDMD (mg/100 mg DM, 96 h) had lower degradation (P < .03) for hay at 35 d compared with the rest of the treatments.
Table 4. In vitro gas production (mL gas/g DM) of ryegrass hay (H) and ryegrass silage (S) at two maturity stages (21 or 35 days).
Discussion
Chemical composition
In this study, silage and hay from ryegrass exhibited different chemical compositions and nutritional value which agrees with previous reports on silage and hay from grasses (Mapato and Wanapat Citation2018). However, grass maturity and harvesting time are known to be important factors for biomass quality (Butkutė et al. Citation2013; Solati et al. Citation2017). Fibre was the principal component of ryegrass, with high concentrations of NDF and ADF even at an early growth stage (21d, NDF and ADF ≥ 509 and 333 g/kg DM, respectively). This is typical of perennial ryegrass species (Chaves et al. Citation2006; Ulyatt, Citation1984), which differ from pastures that contain clovers and weed species (Celis-Alvarez et al. Citation2021). Mambrini et al. (Citation1994) and Dohme et al. (Citation2007) described a reduction in the CP and organic matter digestiblity as ryegrass maturity advanced which partly agrees with results from the present study.
Silage quality refers to a combination of chemical factors related to ensiling pH and the concentrations of fermentation end products that together indicate how efficiently nutrients have been preserved during the fermentation and storage phases (Cherney and Cherney Citation2003; Naoki and Yuji Citation2008). Silage DM content was similar to that reported in timothy and meadow fescue sward silage (Rinne et al. Citation1997), but CP content was lower. In this study, S21 and S35 resulted to be well-preserved silages, as indicated by 4.2 pH, a lactate-to-acetate ratio greater than 2:1; and a concentration of butyrate of 22 mol/100 mol (Buxton and O’Kiely Citation2003). In this regard, our results coincide with Winichayakul et al. (Citation2020). The main factors influencing the fat content and composition of forages are already known (Khan et al., Citation2009). The reduction in fat content due to ensiling in our experiments was similar to Glasser et al. (Citation2013). During the overall ensiling process, two major processes affect lipids and fatty acids: (1) During the aerobic field-wilting phase, reductions in total fatty acid content and polyunsaturated fatty acids concentrations occur. (2) During anaerobic fermentation, lipolysis of membrane lipids is extensive, and this yields to the majority of fatty acids in silage occurring as free fatty acids, while fatty acid composition is largely unaffected.
In the hay, CP was similar to that reported by Huhtanen et al. (Citation2002) and Agbossamey et al. (Citation1998) in perennial ryegrass, but higher than that described by Shingfield et al. (Citation2001) from timothy and meadow fescue swards. In this study, CP was reduced with maturity in hay and that agreed with Chaves et al. (Citation2006), who associated CP changes to increases in NDF and ADF fibre fractions. Several authors have reported that N from chemical fertilisation increases the content of CP in DM by increasing soluble N compounds such as amino acids, nitrates, and peptides and, consequently, enhancing the synthesis of soluble protein in the plant (Loazia et al., 2017; Solati et al. Citation2017), which is higher in silages compared to hays. Plant nitrogen is mainly stored as protein in the chloroplast (Peoples and Dalling Citation1988), and hence located in the form of true proteins within leaves. It has further been shown, that leaf-protein contents decrease with plant maturity as a reaction to senescence and subsequent lignification (Sanderson and Wedin Citation1989), contributing to a substantial increase in total plant lignin (Butkutė et al. Citation2013) but this study was based on plant material with less than one week of maturity. Our results coincides with Särkijärvi et al. (Citation2012), who evaluated Thimoty and Festuca grasses at different stages of maturity, and reported that the interval of one week between cuts increases the content of NDF and decreases CP. These constant alterations with increasing plant development facilitate the bounding of proteins to cell walls, and hence increase the content of insoluble protein fractions B3 and C according to the CNCPS method, while the soluble protein fractions B1 and B2 decrease for both hay and silages at 21 and 35d.
Regardless of fraction A, it was expected that forages at 21 d had the greatest values of fraction A, which coincides with Henriques et al. (Citation2007). This is due to the nitrate absorbed by the plant and reduction to ammonia, which is a substrate for amino acid and protein synthesis, increasing plant NPN contents (Gomes et al. Citation2018) at early stages.
True protein tends to be extensively degraded in the rumen, contributing to the N supply of the ruminal microorganisms and incorporation into carbon skeletons (Sniffen et al. Citation1992). However, rapid rumen proteolysis of this fraction can lead to peptide construction and escape into the intestine, as the use of these components is limiting protein degradation. Fraction B1 + B2, which represents true proteins, increased in young forages (Table ), since it increased N compounds of tissues and the protein synthesis of plants, being important in the formation and development of tillers (Johnson et al. Citation2001). The present results coincide with Nielsen et al. (Citation2021), who demonstrated that the allocation of plant protein between CNCPS fractions was affected by harvest date, underlining the importance of plant maturity.
Chilibroste et al. (Citation2000) reported a linear increase in NDF concentration of ryegrass over 30 days of growth from 446 to 532 g/kgDM at 6–30 days of re-growth, respectively. The NDF is the predominant fibre fraction in ryegrass, even at vegetative stages and the increase with maturation occurs before the appearance of the stem or inflorescence (Ulyatt et al. Citation1988). With regard to nutritional quality, Roth et al. (Citation2017) suggested that a suitable silage for dairy cattle has low NDF (51%) and ADF (32.9%) but high CP (16.8%) contents, similar to that from McCormick et al. (Citation1998) and Simionatto et al. (Citation2019) who reported that ryegrass silage has a CP content of more than 19%. NFC is among the most digestible nutrients, consisting of organic acids (a breakdown product of carbohydrates), sugars, starches, pectins, and any carbohydrate soluble in a neutral detergent solution. Together with digestible NDF, NFC comprises the main energy sources available in the rumen, and these decreased as the age of the plant increased (21 vs 35 d) (Table1), impacting on a lower consumption (P = .008) as the age of the plant increased (Table ). However, enhancing the content of one nutritional component results in changes in others. In fact, our results showed a 57% decrease in NFC for H35 compared with H21, but only 16% for 35 compared with S21 ryegrass.
Intake and apparent in vivo digestibility
Roughages play an important role in rumen fill and therefore affect DM intake (Liu et al. Citation2005; Lechartier and Peyraud Citation2010; da Silva et al. Citation2015). In this study, hay-fed and silage-fed lambs had similar DM intakes. This finding differs from previous reports, for example, silage-based diets allow a greater adhesion of concentrate particles minimising the selection of ingredients by the animal (Ma et al. Citation2014; Huelva et al. Citation2017). Similarly, Castells et al. (Citation2012) worked with different types of forage when feeding dairy calves and observed that supplying hay as the main roughage promotes the animal’s ability to choose only those preferred feedstuffs. Contrary to our study, De O. Nascimento et al. (Citation2020) reported that the roughage type influenced intake of DM, CP, and NDF, with the highest contents in diets containing more hay than silage.
Huhtanen and Jaakkola (Citation1993) did not find differences in digestibility of H or S at the same growth stage. However, our results coincide with Agbossamey et al. (Citation1998) and Mapato and Wanapat (Citation2018). In those studies, lambs were fed with H or S, and reported differences in DM and CP digestibility, being higher in S than in H and S was more degradable, showing a lower quantity of cellulose than H (Agbossamey et al. Citation1998; Özelçam et al. Citation2015).
In this study, H35 had the lowest DM and OM digestibility (P < .001). It is considered that the maturity stage of the pastures is the main element that affects their chemical composition and nutritional quality. In this regard, as the cutting age increases NDF and ADF contents, and DM digestibility decrease. Interestingly, in the present study, H35 had the lowest IVDMD compared with S21, which may be due to plant enzyme activity during silage (Ribeiro et al. Citation2014). The filling effect of mature grass (or higher dietary fibre content) is due to resistance to particle size reduction during rumination or chewing (Dulphy et al. Citation1989; Dado and Allen Citation1995). Ruminants often have high intakes that result in the passage of particles at a similar rate as digestion rates of fibre (Mertens Citation1992). However, some fibre is not digested and will bypass rumen before microbial fermentation is completed.
Nitrogen balance and excretion of purine derivatives
In the current study, compared with silage-fed lambs, those fed on hay consumed the same amount of DM and N. This response can be attributed to the physical characteristics of ryegrass fodder. Hay is a feedstuff with a high DM content (>90%); therefore, when hay is fed with concentrated feed, which is also high in DM but denser in energy, animals often choose concentrated feeds first. On the contrary, because silage has about 76% of moisture, it can allow a higher adhesion of concentrate particles to its structure and minimise the selection of ingredients by the animal in the trough. This partly explains the similar DM and N intakes of diets containing hay and silage, without being affected by cutting age. Animals fed H35 had lower ADG, possibly due to lower fermentation and lower IVDMD, and this was reflected in lower PD excretion. Allantoin was the predominant PD (89.6%) and that level coincides with previous reports on rumen microbial-nitrogen production in sheep (Pérez et al. Citation1996). Allantoin and total PD excretion were different for all treatments, being higher in S21. If we were to supply an excess of CP in the diet, we may obtain a positive response related to MCP synthesis, which could be due to improved synchronisation of energy use from S, in contrast, the rapid release of soluble N with possible asynchrony with dietary carbohydrates could be the main cause of the low efficiency of MCP synthesis when H is used (Siddons et al. Citation1985) and with the lowest excretion of allantoin and PD in the urine.
In vitro gas production
To evaluate the influence of hay or silage fermentation, we measured total gas production (mL gas/gDM) from in vitro rumen incubations. With regard to hay, H35 showed reductions in total gas (approximately 27% less for H35 compared with H21), compared with silages (S21 and S35). Total gas production (mL g/DM) was lower for H35 compared with H21. Harvesting time affected (P < .001) the in vitro dry matter digestiblity of forages (Navarro-Villa et al. Citation2011). This was generally being highest in the early harvest from the primary growth and lowest in the late harvest from the primary growth (P < .05), which coincides with the present results. No significant differences were detected in in vitro dry matter digestiblity between ryegrass silages (P < .05).
Dohme et al. (Citation2007) evaluated prairies composed of grasses (L. perenne, Phleum pratense) and clover (Trifolium repens, T. pratense) at two maturity stages. Their results were similar to the present study, where S presented a higher rate of degradation compared to H, and it was higher in young prairies in relation to the maturity stage of the prairie. Consequently, S had higher IVDMD in relation to H and this was probably due to a previous fermentation (cause by the silage fermentation process), and this allowed a higher IVDMD compared with H.
Wang et al. (Citation2020) evaluated different ryegrass cultivars, reporting positive relationships with NFC/N and NFC/NDF with IVDMD, which agrees with the fact that NDF content has a negative relationship with digestibility (Stergiadis et al. Citation2015; Wang et al. Citation2020). As shown in Table , H35 had higher NDF (574 g/kg DM) and lower NFC (52 g/kgDM) than the rest of the treatments, showing lower IVDMD (52.4 mg/100 mg).
In this paper, we discussed the potential use of ryegrass preserved as hay or silage to enhance forage feeding value in growing lambs. Further studies should consider a completely randomised design with more animals per treatment, and extend the growing performance trial and the cutting maturity age in order to see a greater effect of plant maturity state on animal performance. Under our conditions, we opted for a completely randomised factorial arrangement design that would allow us to evaluate above all nutrient intake and digestibility, however, a longer (i.e. 60-90 days) performance trial would confirm our results.
Conclusions
Overall, compared with ryegrass hay, ryegrass silage had a higher in vitro degradation. There was a higher intake of ryegrass cut at 21 d than at 35 d, and microbial crude protein synthesis was higher in silage-fed lambs than those fed with hay. Hay cut at 35 d had the lowest in vitro gas production, DM and OM disappearance, and microbial protein production. Ensiling at 21 d could be a convenient option for preserving ryegrass as a locally produced feed and could be recommended for increasing nutrient intake and improving in vitro fermentation kinetics, N balance, and purine derivatives when fed to growing lambs.
Acknowledgements
Lizbeth Esmeralda Robles-Jiménez and Jose Romero were awarded a Conacyt-Mexico scholarship during their Ph.D. program at the Agricultural Sciences and Natural Resources at the Universidad Autonoma del Estado de México.
Disclosure statement
No potential conflict of interest was reported by the authors.
Data availability statement
Data and materials that support the results and analyses presented in this study are freely available upon request.
Additional information
Funding
References
- Agbossamey YR, Petit HV, Seoane JR, St-Laurent GJ. 1998. Performance of lambs fed either hay or silage supplemented with canola or fish meals. Can J Anim Sci. 78(1):135–141.
- AOAC. 1990. Official methods of analysis. 15th Ed. Arlington (VA): Association of Official Analytical Chemists.
- Balcells J, Guada JA, Peiró JM, Parker DS. 1992. Simultaneous determination of allantoin and oxypurines in biological fluids by high-performance liquid chromatography. J Chrom B: Bio Sci Appl. 575(1):153–157.
- Blümmel M, Steingaβ H, Becker K. 1997. The relationship between in vitro gas production, in vitro microbial biomass yield and 15N incorporation and its implications for the prediction of voluntary feed intake of roughages. Br J Nutr. 77(6):911–921.
- Bryant RH, Gregorini P, Edwards GR. 2012. Effects of N fertilisation, leaf appearance and time of day on N fractionation and chemical composition of Lolium perenne cultivars in spring. Anim Feed Sci Tech. 173(3–4):210–219.
- Butkutė B, Lemežienė N, Cesevičienė J, Liatukas Ž, Dabkevičienė G. 2013. Carbohydrate and lignin partitioning in switchgrass biomass Panicum virgatum L. as a bioenergy feedstock. Zemdirbyste-Agriculture. 100(3):251–260.
- Buxton DR, O’Kiely P. 2003. Preharvest plant factors affecting ensiling. Silage Sci. Technol. 42:199–250.
- Castells L, Bach A, Araujo G, Montoro C, Terré M. 2012. Effect of different forage sources on performance and feeding behavior of Holstein calves. J Dairy Sci. 95(1):286–293.
- Celis-Alvarez MD, López-González F, Arriaga-Jordán CM, Robles-Jiménez LE, González-Ronquillo M. 2021. Feeding forage mixtures of ryegrass (Lolium spp.) with clover (Trifolium spp.) supplemented with local feed diets to reduce enteric methane emission efficiency in small-scale dairy systems: a simulated study. Animals. 11(4):946.
- Chaves AV, Waghorn GC, Brookes IM, Woodfield DR. 2006. Effect of maturation and initial harvest dates on the nutritive characteristics of ryegrass (Lolium perenne L.). Anim Feed Sci Tech. 127(3–4):293–318.
- Cherney J, Cherney D. 2003. Assessing silage quality. Silage Sci Technol. 42:141–198.
- Chilibroste P, Tamminga S, Boer H, Gibb MJ, den Dikken G. 2000. Duration of regrowth of ryegrass (Lolium perenne) effects on grazing behavior, intake, rumen fill, and fermentation of lactating dairy cows. J Dairy Sci. 83(5):984–995.
- Dado RG, Allen MS. 1995. Intake limitations, feeding behavior, and rumen function of cows challenged with rumen fill from dietary fiber or inert bulk. J Dairy Sci. 78(1):118–133.
- De O. Nascimento C, Santos SA, dos S. Pina D, Tosto MSL, Pinto LFB, Eiras DN, de Ssis DYC, Perazzo AF, de Araujo MLGML, Azevedo JAG, et al. 2020. Effect of roughage-to-concentrate ratios combined with different preserved tropical forages on the productive performance of feedlot lambs. Small Rumin Res. 182:15–21.
- Dohme F, Graf CM, Arrigo Y, Wyss U, Kreuzer M. 2007. Effect of botanical characteristics, growth stage and method of conservation on factors related to the physical structure of forage – an attempt towards a better understanding of the effectiveness of fibre in ruminants. Anim Feed Sci Tech. 138(3–4):205–227.
- Dulphy JP, Faverdin P, Jarrige R. 1989. Feed intake: the fill unit systems. In: Jarrige R, editor. Ruminant nutrition. recommended allowances and feed tables. Paris: INRA. p. 61–71.
- Fraser MD, Speijers MHM, Theobald VJ, Fychan R, Jones R. 2004. Production performance and meat quality of grazing lambs finished on red clover, lucerne or perennial ryegrass swards. Grass and Forage Sci. 59(4):345–356.
- Getachew G, Makkar HPS, Becker K. 2002. Tropical browses: contents of phenolic compounds, in vitro gas production and stoichiometric relationship between short chain fatty acid and in vitro gas production. J Agric Sci. 139(3):341–352.
- Givens DI, Rulquin H. 2004. Utilisation by ruminants of nitrogen compounds in silage-based diets. Anim Feed Sci Tech. 114(1–4):1–18.
- Glasser F, Doreau M, Maxin G, Baumont R. 2013. Fat and fatty acid content and composition of forages: a meta-analysis. Anim Feed Sci Tech. 185(1–2):19–34.
- Gomes FK, Oliveira MDBL, Homem BGC, Boddey RM, Bernardes TF, Gionbelli MP, Lara MAS, Casagrande DR. 2018. Effects of grazing management in brachiaria grass-forage peanut pastures on canopy structure and forage intake. J. Anim. Sci. 96(9):3837–3849.
- González-Ronquillo M, Fondevila M, Barrios Urdaneta A, Newman Y. 1998. In vitro gas production from buffel grass Cenchrus ciliaris L. fermentation in relation to the cutting interval, the level of nitrogen fertilization and the season of growth. Anim Feed Sci Tech. 72(1–2):19–32.
- Haigh PM, Hopkins JR. 1977. Relationship between oven and toluene dry matter in grass silage. J Sci Food Agric. 28(6):477–480.
- Henriques LT, da Silva JC, Detmann E, Vásquez HM, Pereira OG. 2007. Fractions of nitrogenous compounds of four tropical grasses in the different cutting ages and nitrogen fertilizer levels. Arq Bras Med Vet Zootec. 59(3):740–748.
- Hintz RW, Mertens DR, Albrecht KA. 1996. Effects of sodium sulfite on recovery and composition of detergent fiber and lignin. J Aoac. 79(1):16–22.
- Huelva RM, Fenosa RMA, Gonzalez PR, Casado GP, Alcaide ME. 2017. Can byproducts replace conventional ingredients in concentrate of dairy goat diet. J Dairy Sci. 100:4500–4512.
- Huhtanen P, Jaakkola S. 1993. The effects of forage preservation method and proportion of concentrate on digestion of cell wall carbohydrates and rumen digesta pool size in cattle. Grass and Forage Sci. 48(2):155–165.
- Huhtanen P, Khalili H, Nousiainen JI, Rinne M, Jaakkola S, Heikkilä T, Nousiainen J. 2002. Prediction of the relative intake potential of grass silage by dairy cows. Livest Prod Sci. 73(2-3):111–130.
- Huhtanen P, Rinne M, Nousiainen J. 2007. Evaluation of the factors affecting silage intake of dairy cows: a revision of the relative silage dry-matter intake index. Animal. 1(5):758–770.
- Jančík F, Rinne M, Homolka P, Čermák B, Huhtanen P. 2011. Comparison of methods for forage digestibility determination. Anim Feed Sci Tech. 169(1-2):11–23.
- Johnson CR, Reiling BA, Mislevy P, Hall MB. 2001. Effects of N fertilization and harvest date on yield, digestibility, fiber, and protein fractions of tropical grasses. J Anim Sci. 79(9):2439–2448.
- Kallah MS, Baba M, Alawa JP, Muhammad IR, Tanko RJ. 1997. Ensiling quality of columbus grass Sorghum almum grown in northern Nigeria. Anim Feed Sci Tech. 68(1–2):153–163.
- Khan NA, Cone JW, Hendriks WH. 2009. Stability of fatty acids in grass and maize silages after exposure to air during the feed out period. Anim Feed Sci Tech. 154(3–4):183–192.
- Kijak K, Pino F, Heinrichs AJ. 2017. Effect of forage to concentrate ratio with Sorghum silage as a source of forage on rumen fermentation, N balance, and purine derivative excretion in limit-fed dairy heifers. J Dairy Sci. 100:1–11.
- Kirchhof S, Eisner I, Gierus M, Sudekum KH. 2010. Variation in the contents of crude protein fractions of different forage legumes during the spring growth. Grass and Forage Science. 65(4):376–382.
- Krishnamoorthy U, Soller H, Steingass H, Menke KH. 1991. A comparative study on rumen fermentation of energy supplements in vitro. J Anim Physiology Anim Nutr. 65(1–5):28–35.
- Lanzas C, Broderick GA, Fox DG. 2008. Improved feed protein fractionation schemes for formulating rations with the Cornell Net Carbohydrate and Protein System. J Dairy Sci. 91(12):4881–4891.
- Lechartier C, Peyraud JL. 2010. The effects of forage proportion and rapidly degradable dry matter from concentrate on ruminal digestion in dairy cows fed corn silage-based diets with fixed neutral detergent fiber and starch contents. J Dairy Sci. 93(2):666–681.
- Licitra G, Hernandez TM, Van Soest PJ. 1996. Standardization of procedures for nitrogen fractionation of ruminant feeds. Anim Feed Sci Tech. 57(4):347–358.
- Liu X, Wang Z, Lee F. 2005. Influence of concentrate level on dry matter intake, N balance, nutrient digestibility, ruminal outflow rate, and nutrient degradability in sheep. Anim Feed Sci Tech. 58:55–62.
- Loaiza PA, Balocchi O, Bertrand A. 2017. Carbohydrate and crude protein fractions in perennial ryegrass as affected by defoliation frequency and nitrogen application rate. Grass Forage Sci. 72(3):556–567.
- Luo J, Goetsch AL, Nsahlai IV, Sahlu T, Ferrell CL, Owens FN, Galyean ML, Moore JE, Johnson ZB. 2004a. Metabolizable protein requirements for maintenance and gain of growing goats. Small Rumin Res. 53(3):309–326.
- Luo J, Goetsch AL, Sahlu T, Nsahlai IV, Johnson ZB, Moore JE, Galyean ML, Owens FN, Ferrell CL. 2004b. Prediction of metabolizable energy requirements for maintenance and gain of preweaning, growing and mature goats. Small Rumin Res. 53(3):231–252.
- Ma T, Deng K-D, Tu Y, Zhang N-F, Jiang C-G, Liu J, Zhao Y-G, Diao Q-Y. 2014. Effect of dietary forage-to-concentrate ratios on urinary excretion of purine derivatives and microbial nitrogen yields in the rumen of Dorper crossbred sheep. Livest Sci. 160:37–44.
- Mambrini M, Peyraud JL, Hetault H, Stephant A, Texier M. 1994. Mean retention time in digestive tract and digestion of fresh perennial ryegrass by lactating dairy cows influence of grass maturity and comparison with a maize silage diet. Reprod Nutr Dev. 34(1):9–23.
- Mapato C, Wanapat M. 2018. Comparison of silage and hay of dwarf Napier grass (Pennisetum purpureum) fed to Thai native beef bulls. Trop Anim Health Prod. 50(7):1473–1477.
- McCormick ME, Cuomo GJ, Blouin DC. 1998. Annual ryegrass stored as balage, haylage or hay for lactating dairy cows. J Prod Agric. 11(3):293–300.
- Menke KH, Steingass H. 1988. Estimation of energetic feed value obtained from chemical analyses and in vitro gas production using rumen fluid. Animal. Research and Development. 28:7–55.
- Mertens DR. 1992. Nonstructural and structural carbohydrates. In: Van Horn HH, Wilcox CJ, editor . Large dairy herd management. Champaign (IL): ADSA. p. 219–235.
- Moon NJ, Ely LO, Sudweeks EM. 1981. Fermentation of wheat, corn and alfalfa silages inoculated with Lactobacillus acidophilus and Candida sp. at ensiling. J Dairy Sci. 64(5):807–813.
- Naoki N, Yuji T. 2008. Variations in bacterial communities in laboratory-scale and big bale silos assessed by fermentation products, colony counts and denaturing gradient gel electrophoresis profiles. Lett Appl Microbiol. 46(3):283–288. -765X.2007.02306.x.
- Navarro-Villa A, O’Brien M, López S, Boland TM, O’Kiely P. 2011. In vitro rumen methane output of red clover and perennial ryegrass assayed using the gas production technique (GPT). Anim Feed Sci Tech. 168(3–4):152–164.
- Nielsen CK, Stødkilde L, Jørgensen U, Laerke PE. 2021. Effects of harvest and fertilization frequency on protein yield and extractability from flood-tolerant perennial grasses cultivated on a fen peatland. Front Environ Sci. 9(47):619258.
- Obraztsov NR, Shchedrina DI, Kadyrov SV. 2020. Festulolium seed productivity depending on sowing methods and seeding rates. In: IOP Conference Series: Earth and Environmental Science, 422(1): 0120256th
- Olaiz BG. 2015. Aspectso bioeticos de la experimentacion animal. En: Gaceta Conbioetica. 16:21–23.
- Özelçam H, Kırkpınar F, Tan K. 2015. Chemical composition, in vivo digestibility and metabolizable energy values of Caramba Lolium multiflorum cv. caramba fresh, silage and hay. Asian Austr J Anim Sci. 2810:1427–1432.
- Penchev P, Ilieva Y, Ivanova T, Kalev R. 2016. Fatty acid composition of buffalo and bovine milk as affected by roughage source – silage versus hay. Emir J Food Agric. 28(4):264–270.
- Peoples MB, Dalling MJ. 1988. The interplay between proteolysis and amino acid metabolism during senescence and nitrogen reallocation. In: Nooden LD, Leopold AC, editor. Senescence and aging in plants. San Diego (United States): Academic Press Inc.
- Pérez JF, Balcells J, Guada JA, Castrillo C. 1996. Determination of rumen microbial-nitrogen production in sheep: a comparison of urinary purine excretion with methods using 15N and purine bases as markers of microbial-nitrogen entering the duodenum. Brit J Nutr. 75:699–709.
- Ribeiro GO, Teixeira AM, Velasco FO, Faria Júnior WG, Pereira LGR, Chaves AV, Gonçalves LC, McAllister TA. 2014. Production, nutritional quality and in vitro methane production from andropogon gayanus grass harvested at different maturities and preserved as hay or silage. Asian-Australas J Anim Sci. 27(3):330–341.
- Rinne M, Jaakkola S, Huhtanen P. 1997. Grass maturity effects on cattle fed silage-based diets. 1. Organic matter digestion, rumen fermentation and nitrogen utilization. Anim Feed Sci Tech. 67(1):1–17.
- Roth GW, Ishler VA, Jones CM. 2017. From Harvest to Feed: Understanding Silage Management. Penn State Extension. https://extension.psu.edu/from-harvest-to-feed-understanding-silagemanagement.
- Sanderson MA, Wedin WF. 1989. Nitrogen in the detergent fibre fractions of temperate legumes and grasses. Grass Forage Sci. 44(2):159–168.
- Särkijärvi S, Sormunen-Cristian R, Heikkilä T, Rinne M, Saastamoinen M. 2012. Effect of grass species and cutting time on in vivo digestibility of silage by horses and sheep. Livest Sci. 144(3):230–239.
- SAS 1999. SAS/STAT software: changes and enhancements through release 8.2. Cary (NC): SAS Institute.
- Shingfield KJ, Jaakkola S, Huhtanen P. 2001. Effects of level of nitrogen fertilizer application and various nitrogenous supplements on milk production and nitrogen utilization of dairy cows given grass silage-based diets. Anim Sci. 73(3):541–554.
- Siddons RC, Nolan JV, Beever DE, Macrae JC. 1985. Nitrogen digestion and metabolism in sheep consuming diets containing contrasting forms and levels of N. Br J Nutr. 54(1):175–187.
- da Silva TC, Pereira OG, Agarussi MCN, da Silva VP, da Silva LD, Cardoso LL, Ribeiro KG, Valadares Filho SC. 2015. Stylosanthes cv. Campo Grande silage with or without concentrate in sheep diets: nutritional value and ruminal fermentation. Small Rumin Res. 126:34–39.
- Simionatto M, Maeda EM, Fluck AC, Silveira AP, Piran Filho FA, Matielo de Paula FL, Denardin Costa OA, Rothe Mayer LR, de Paulo Macedo V. 2019. Nutritional and morphostructural characterization of pre-dried winter grass silage. SCA. 40(5Supl1):2375.
- Sniffen CJ, O'Connor JD, Van Soest PJ, Fox DG, Russell JB. 1992. A net carbohydrate and protein system for evaluating cattle diets: II. Carbohydrate and protein availability. J Anim Sci. 70(11):3562–3577.
- Solati Z, Jørgensen U, Eriksen J, Søegaard K. 2017. Dry matter yield, chemical composition and estimated extractable protein of legume and grass species during the spring growth. J Sci Food Agric. 97(12):3958–3966.
- Steel RDG, Torrie JH, Dickey DA. 1997. Principles and procedures of statistics: a biomedical approach, 3rd ed. New York (NY): McGraw-Hill Book.
- Steen RWJ, Gordon FJ, Dawson LER, Park RS, Mayne CS, Agnew RE, Kilpatrick DJ, Porter MG. 1998. Factors affecting the intake of grass silage by cattle and prediction of silage intake. Anim Sci. 66(1):115–127.
- Stergiadis S, Allen M, Chen XJ, Wills D, Yan T. 2015. Prediction of nutrient digestibility and energy concentrations in fresh grass using nutrient composition. J Dairy Sci. 98(5):3257–3273.
- Theodorou MK, Williams BA, Dhanoa MS, McAllan AB, France J. 1994. A simple gas production method using a pressure transducer to determine the fermentation kinetics of ruminant feeds. Anim Feed Sci Tech. 48(3–4):185–197.
- Turner LR, Donaghy DJ, Lane PA, Rawnsley RP. 2006. Effect of defoliation management, based on leaf stage, on perennial ryegrass (Lolium perenne L.), prairie grass Bromus (willdenowii Kunth.) and cocksfoot (Dactylis glomerata L.) under dryland conditions. 2. Nutritive value. Grass Forage Sci. 61(2):175–181.
- Ulyatt MJ, Thomson DJ, Beever DE, Evans RT, Haines MJ. 1988. The digestion of perennial ryegrass (Lolium perenne cv. Melle) and white clover (Trifolium repens cv. Blanca) by grazing cattle. Br J Nutr. 60(1):137–149.
- Ulyatt MJ, Waghorn GC, John A, Reid CSW, Monro J. 1984. Effect of intake and feeding frequency on feeding behaviour and quantitative aspects of digestion in sheep fed chaffed lucerne hay. J Agric Sci. 102(3):645–657.
- Vaca García VM, Martínez Villanueva JJ, González Huerta A, Morales Rosales E, Zamudio González B, Gutiérrez RF. 2018. Compactación de un vertisol bajo tres sistemas de labranza en maíz (Zea mays L.). Remexca. 5(8):1495–1507.
- Van Soest PJ, Robertson JB, Lewis BA. 1991. Methods for dietary fiber, neutral detergent fiber, and nonstarch polysaccharides in relation to animal nutrition. J Dairy Sci. 74(10):3583–3597.
- Van Soest P J. 1994. Nutritional ecology of the ruminant. Ithaca, NY. Cornell University Press.
- Vargas-Bello-Pérez E, Robles-Jimenez LE, Ayala-Hernández R, Romero-Bernal J, Pescador-Salas N, Castelán-Ortega OA, González-Ronquillo M. 2020. Effects of calcium soaps from palm, canola and safflower oils on dry matter intake, nutrient digestibility, milk production, and milk composition in dairy goats. Animals. 10(10):1728.
- Wang C, Hou F, Wanapat M, Yan T, Kim E, Scollan N. 2020. Assessment of cutting time on nutrient values, in vitro fermentation and methane production among three ryegrass cultivars. Asian-Australas J Anim Sci. 33(8):1242–1251.
- Winichayakul S, Beechey-Gradwell Z, Muetzel S, Molano G, Crowther T, Lewis S, Xue H, Burke J, Bryan G, Roberts NJ. 2020. In vitro gas production and rumen fermentation profile of fresh and ensiled genetically modified high–metabolizable energy ryegrass. J Dairy Sci. 103(3):2405–2418.