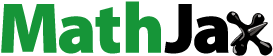
Abstract
The main goal of breeding programmes is to maximise the genetic improvement of the economic traits of farm animals. Beside the economic traits, the birds’ survival has directly influenced on the economic gain. Therefore, this study aimed to investigate the genetic relationship between survival and productive traits in Japanese quail. A total of 1854 records were collected during four generations from 2017 to 2019 in Khorasan Razavi Agricultural Research and Training Centre (KRARTC). Data were used to estimate the genetic correlations among quail survival (S) with egg weight (EW) and body weight gain (BWG) traits. Linear-threshold model was used to estimate (co)variance components, and genetic parameters of traits via the Gibbs sampling method. The heritability of EW was 0.04, and the heritability estimates for survival at different ages ranged from 0.138 to 0.399 in joint analysis with EW. The highest negative genetic correlation between EW and S0–14 (i.e. survival up to 14 d) was obtained as −0.704. The highest and lowest heritability of weight gain traits for BWG0–7 and BWG7–14 were 0.583 and 0.116, respectively. The analyses of BWG traits with survival at different ages revealed that the genetic correlations ranged from 0.015 (between BWG0–7 and S0–14) to 0.638 (between BWG0–7 and S0–7). This study showed that the genetic selection for survival at different ages could be effective. On the other hand, selection for BWG0–7 could also indirectly improve the survival in quails because of the positive and high genetic correlation between BWG0–7 with S0–7.
Genetic improvement of production traits in quail may lead to reduced survival in quail. Studies regarding on the survival of quail were yet not carried out.
Heritability of the survival at different ages showed that genetic selection for this trait could improve the survival.
Survival at different ages had a negative genetic correlation with egg weight (EW), and body weight gain (BWG) traits after 21 d of old.
Highlights
Introduction
The beginning of breeding programmes in quails has lasted for more than three decades and considerable progression has been attained in terms of product quantity. Despite the development of quail breeding, maximising quality and quail performance have received less attention. Compared with other commercial poultry species, some attributes, such as short generation interval, higher egg production rate, small body size, fast growth, early puberty and lower feed intake have been considered as the most attractive characteristics to select the quail as the animal models in breeding programmes (Okamoto et al. Citation1989). Maximising quail productivity is the main goal of breeding programmes for industry. On the other hand, the industrial productivity is a function of performance and reproductive traits while the mortality is the most important limiting factor. A lot of genetic and environmental factors may affect the mortality, in which the most effective elements are the genetic capacity of the bird, genetic abnormalities, the plan of population management and nutrition. So far, several studies have investigated the reasons of mortality and factors affecting the rate of these parameters in the poultry industry. It was reported that environmental factors have the profound impacts on the growth rate and mortality of quail chicks in early days post-hatch (Aggrey and Marks Citation2002).
In the study on quail Cotuenix c. coturnix in two regions of Italy, the average of annual mortality rate ranged from 69 to 73% (Puigcerver Citation1992), however, other experiments on liabat three research stations of Florida resulted in 54% mortality rate on average for both sexes per year (Pollock et al. Citation1989).
By definition, survival has been mentioned as the opposite of mortality. Survival in the population is the most critical issues in the breeding farms that calls more attention by scientists. Survival significantly affects the net profit of breeding farms, and increasing the population survival reduces production costs. Usually, longevity in the bovine species is evaluated not on the basis of the spontaneous animal death, but on the culling choice following a reduction in production and/or functional performance (Van Raden and Wiggans Citation1995; McCullough and DeLorenzo Citation1996).
The recording of bird survival in the farm is the difficult task and it conveniently may be recorded after culling of the animals. Therefore, the use of correlated traits with survival which are easy to record could be applicable to extend the bird’s survival. Body weight (BW) and egg weight (EW) are traits that have been studied extensively and their heritability and genetic correlations have been reported in many studies. Therefore, knowledge of the genetic correlations between BW and EW with survival can be effective in improving survival by using indirect selection.
The body weight gain (BWG) and EW are the continuous traits that can be used in the best linear unbiased prediction (BLUP) methodology to predict breeding values for those traits with normal distribution. In contrast, the survival is the discontinuous traits (i.e. discrete) and (co)-variance components of discontinuous traits, such as survival could be estimated by threshold methods (Gianola and Foulley Citation1983; Hagger and Hofer Citation1990). Evaluating both continuous and threshold traits simultaneously resulted in high accuracy of selection, especially for the threshold traits (Riggio et al. Citation2005; Cloete et al. Citation2009; Hatcher et al. Citation2010).
The most of the genetic improvement studies have focussed on the number of early eggs, BW and growth traits at fixed ages (Kaplan et al. Citation2016, Finco et al. Citation2016; Mohammadi-Tighsiah et al. Citation2018; Gotuzzo et al. Citation2019; Ozsoy Citation2019a, Citation2019c) Aggrey and Marks (Citation2002) evaluated the risk factors with the occurrence of mortality in divergently selected lines and controls of Japanese quail. To our knowledge, there are no studies regarding the estimation of survival variance components in quail and this study is the first report on this topic. Although there are few reports on the correlation of BWG and other traits including feed conversion ratio in the chickens (N’Dri et al. Citation2006), feed residual in the chickens (Aggrey et al. Citation2010), feed conversion ratio in Pekin duck (Zhang et al. Citation2017) and humoral immunity in quail (Mohammadi-TighSiah et al. Citation2018), there is no report on the correlation between BWG and survival in quail. Therefore, this study aimed to analyse survival and its relationship with EW and BWG from hatch to 42 d in Japanese quail, considering the time range for survival trait using linear-threshold model via Gibbs sampling method.
Material and methods
A total of 1854 records related to the 3916 birds from the mating of 243 sires and 243 dams were collected in four generations from 2017 to 2019 by the Khorasan Razavi Agricultural Research and Training Centre (KRARTC). The parents (one male and one female) were reared in the individual breeder cages (30 × 30 × 30 cm). The produced eggs from each cage were numbered separately and kept in the separate combs at 15 °C and 70% humidity until incubation. The produced eggs were transferred to the incubator at 5 or 7 d intervals. On hatching day, all quail chicks were identified by wing bands and the hatching weight was recorded. BW of the birds was recorded by a digital scale (accuracy of 0.01 g) in a seven days period till 42 d of age. The BWGs were calculated as the average growth performance of the birds in a 7 d period. A 20 h light programme was applied from the hatch to the end of the experiment. Diets were formulated based on the recommendations of the National Research Association (NRC Citation1994) and all birds had ad libitum access to the water and feed throughout the experiment. The chickens were weighed weekly and culling date of the birds was recorded. Six components of survival in quails from hatch up to d 7 (S0–7), d 14 (S0–14), d 21 (S0–21), d 28 (S0–28), d 35 (S0–35) and d 42 (S0–42) were considered binomially. The code one was assigned to birds that were in the population at the mentioned ages, otherwise, code zero was used. Accordingly, EW and BWGs at different age periods were as the hatch to d 7 (BWG0–7), 7–14 d (BWG7–14), 14–21 d (BWG14–21), 21–28 (BWG21–28), 28–35 d (BWG28–35) and 35–42 d (BWG35–42). Descriptive statistics of EW, BWGs and survival are shown in Table .
Table 1. Descriptive statistics of studied traits.
After sorting data, multivariate analysis on EW and BWG traits was employed for each bird along with survival using the linear-threshold model. Two multivariate analyses (i.e. S traits + EW and S traits + each of BWG traits) were performed for estimation of genetic parameters of traits. The following model (1) was used for mentioned traits:
(1)
(1)
where yi is the vector of the observation (linear traits including EW and BWG and survival as the threshold trait), Xi is the incidence matrix associating observations to the fixed effects (sex, generation and hatch number), Zi is the incidence matrix associating observation to the additive genetic effect, bi is the vector of fixed effects for the ith trait, ai is the vector of additive genetic and ei is the residual random effects for the ith trait. These analyses assumed a multivariate linear model for EW and BWGs and the threshold model for the bird’s survival. The prior distribution for fixed effects was assumed to be flat. The prior multivariate normal distribution and inverted Wishart distribution were assumed for additive genetic effects and (co)variance matrix of genetic and residual, respectively. Therefore, assumptions were
where G, A and R are the genetic covariance matrix, the numerator relationship matrix, and residual covariance matrix, respectively;
and
are the prior values and degree of freedoms of inverted Wishart distributions for genetic and residual (co)variance matrices, respectively.
In this research, the equations and assumptions have been based on an extension of the equations for a multivariate threshold-linear animal model by Varona et al. (Citation1999). THRGIBBS3F90 program was used to simultaneously fit a threshold model for the survival and a linear model for EW and BWG traits. A single chain of 2,000,000 samples with a burnt-in of the first 200,000 samples and a sampling interval of 100 were generated. Every 100th samples were stored to estimate the posterior mean and posterior standard deviation using POSTGIBBSF90 (Misztal et al. Citation2002).
Result and discussion
Genetic parameters for EW and survival
The variance components and heritability of survival and EW traits are shown in Table . The heritability of EW in the studied population was estimated 0.040, which indicates that genetic selection for this trait in the short term cannot cause genetic improvement, and environmental effects must be considered to improve this trait. EW depends on diet, age at first egg, BW, age, genotype and lighting programme. In fact, any condition that reduces feed intake resulted in EW reduction. Heritability of EW in Japanese quail was reported in the range of 0.25–0.59 (Khaldari et al. Citation2010; Daikwo et al. Citation2013; Momoh et al. Citation2014) that was higher than the result of this study. The heritability of EW in both native chickens of Urmia and Fars was reported as 0.01, which was lower than our finding (Aghazadeh Bokat et al. Citation2014). Ghorbani et al. (Citation2013) reported that the heritability of the first and peak EW in Fars native chickens were 0.33 and 0.64, respectively.
Table 2. Variance components and heritability ± standard deviation of survival and egg weight traits.
In the joint analysis of EW and survival, the heritability of survival at different ages is presented in Table . The lowest and highest heritability were estimated for S0–28 (0.138) and S0–7 (0.399), respectively, suggesting that genetic selection for mentioned traits at different age categories, especially for early days post-hatching (S0–7), could be the effective tool.
The genetic correlation between EW and survival at different ages is shown in Table . All genetic correlations between EW with survival had the negative values, except for S0–7. The highest genetic correlation was estimated between EW and S0–14 (−0.704), while the lowest value was found between EW and S0–7 (0.087). Negative estimations of genetic correlation indicate that an increase in one traits will lead to decrease in another trait and a portion of additive variance is in common between the two traits was done as antagonistic. The survival at different ages may be influenced by different genes, resulting in low response to direct selection. The improvement in survival could be achieved through the management strategies and selection based on direct breeding values without consideration of non-genetic factors affecting the survivability in quail.
Table 3. Genetic correlation of egg weight with the birds’ survival at different ages.
Ideal eggs for hatching and proper EW lead to the production of chickens with suitable body conditions, and this suitable physical condition of the chicken probably reduce the mortality rate of chickens in the first weeks. On the other hand, the survival may be affected by the pre-hatch physical conditions, so that a low correlation between EW and survival at the first week of age was found in this study (Table ). In fact, there was no expectation of a significant association between EW with survival in older ages. However, the results of this study confirm the relationship between EW and survival from post-hatching up to the 6th week of age, and despite the changes, this effect seems to continue until the end of 6 weeks. The genetic correlation between EW and survival at different ages is negative after the first week. The negative correlations decreased from the second to fourth week and increased again after the fourth week. This trend of change remains a question in this study. Some of these changes might be related to the physiological transition of the bird to the maturity and development of genital tract between the second and fourth week. In this case, the selection for EW could be against the survival due to the negative correlations and vice versa.
A positive and almost moderate genetic correlation (in the range of 0.1–0.58) between EW and BW traits was observed in Japanese quails and some poultry species (Ojo et al. Citation2010; Ozsoy and Aktan Citation2011; Kamanli Citation2019). Selection for higher EW in three strains of Tswana chickens improved the egg quality traits (Kgwatalala et al. Citation2016). The high correlation in these studies indicates that the BW and EW may be controlled by many similar genes.
There are few studies on EW and its association with mortality. Skoglund et al. (Citation1952) observed that post-hatching chicks from small eggs tended to die but did not find a significant difference in mortality caused by EW. Other studies also found no association between EW and mortality in broiler chickens (Skoglund and Tomhave Citation1949; Wiley Citation1950). To our knowledge, there were no reports of genetic and phenotypic correlations between EW and survivability.
Genetic parameters for BWG and survival
The variance components and heritability of survival and BWG traits obtained by the multivariate analysis (Table ). The highest heritability was estimated for BWG0–7 (0.583) and followed by BWG14–21, BWG21–28, BWG28–35 and BWG35–42 and BWG7–14.The corresponding values of heritability were 0.583, 0.116, 0.257, 0.171, 0.138 and 0.122, respectively. These low values of heritability indicate that the selection of these traits does not result in any improvement in a short time, and environmental conditions are the determinants to improve mentioned traits. However, the high heritability at early ages (i.e. BWG0–7) could be due to the maternal additive genetic effects, which are reflected in the additive genetic variance and consequently in heritability.
Table 4. Variance components and heritability of survival and body weight gain traits.
In general, the estimated values for heritability of BWG traits were low in a variety of studies, which were in parallel of our findings in this study (Varkoohi et al. Citation2011; Momoh et al. Citation2014; Caetano et al. Citation2017; Mohammadi-Tighsiah et al. Citation2018; Ozsoy Citation2019b). However, the heritability of BWG in the population of Korean native chickens was similar to our estimations (Manjula et al. Citation2018). Some differences in the reported values for heritability of BWG traits could be related to the differences in management and environmental conditions of the populations and also models used for analysis.
In a bivariate analysis of BWG0–7 with the birds’ survival, the heritability of BWG0–7 and S0–7 had the highest values (0.583 and 0.413, respectively), and S0–28 had the lowest value (0.133). S0–21 had the highest heritability (0.300) in analysis with BWG7–14. Moreover, this trait had the highest heritability in analysis with BWG14–21 which was the highest heritability among all studied traits. After 21 d of age, the heritability of BWG and the birds’ survival was decreased. Decreased heritability of BWGs and the birds’ survival after 21 d and low genetic correlation between 21 and 28 d weight gain with S0–28 indicate the fourth week of life is a stressful period and sensitive to quails. The development of the female reproductive system has a direct effect on BW in females, and the increase in weight varies depending on the environmental and genetic conditions of the female could be reasons for this issue. A few factors may attribute to the decline in heritability during the 4th week of age, including sexing, removing surplus chicks (e.g. resulting in small size of population), transferring the chicks to the cage. The chicks in the cage faced with the new spatial and feeding conditions and variation in habituation depends on genotypes. Overall, variation in the estimation of genetic parameters of BW traits could be affected by management systems, nutritional and environmental conditions and incredibly random effects (i.e. chick sample) in the model.
Despite controlling environmental conditions during the incubation period, such as humidity, temperature and ventilation, variation in BW decreases on hatch day. However, environmental variance of the population increases with increasing age and reaches to the highest value at slaughter, resulting in raising the heritability of the BWG0–7 (Daikwo et al. Citation2013; Ebrahimi et al. Citation2019). Moreover, the maternal variance such as egg size could increase the heritability of hatch BW before egg production (Aggrey and Cheng Citation1992).
The genetic correlation of BWG with survival at different ages is shown in Table . The highest genetic correlation was estimated between BWG0–7 with S0–7 (0.638), and the lowest value was attributed to the BWG0–7 with S0–14 (0.015). All correlations were negative except for genetic correlations between BWG0–7 and BWG7–14 with survival. The genetic correlation between BWG0–7 with survival ranged from 0.015 to 0.638. The high value of the positive genetic correlation between BWG0–7 with S0–7 indicates that the selection for BWG in the first week of age could improve the survival. In addition, the genetic correlation between BWG7–14 with the survival ranged from 0.148 to 0.362, in which the highest values were attributed to the BWG7–14 and S0–35 (0.355) and S0–42 (0.362).
Table 5. Genetic correlation of daily weight gain with the birds’ survival at different ages.
Genetic correlations between BWG14–21, BWG21–28, BWG28–35 and BWG35–42 with survival were low and negative, indicating that the genes controlling BWG and survival are different after 14 d of age and may reduce the survival when the selection was accomplished based on BWG. It should be noted that environmental factors could increase the metabolic activity of the chickens resulting in high growth rate and ascites, including low temperature, feeding high-energy diets and increasing feed intake with pelleted diets. Ascites are the metabolic disorder that could be resulted in low survivability (Moghadam et al. Citation2005).
Conclusion
Very low heritability of EW in the studied population indicates that short-term genetic selection for this trait may not be effective on the genetic improvement. Negative estimates of the genetic correlation between EW and survival showed that the transfer of EW genes is different from that of survival genes and selection performed for EW could reduce the survival. Heritability of the survival at different ages showed that genetic selection for this trait at early days post-hatching (i.e. S0–7) could improve the survival. A short-term genetic selection for BWG traits would not applicable to improve the BWG because of low heritability.
Ethical approval
Animal handling and experimental procedures of the study were approved by the Research Animal Committee of the Research Institute at the University of Zabol, Zabol, Iran.
Acknowledgements
The authors thank the Khorasan Razavi Agricultural Research and Training Center (KRARTC) for cooperation in performing this research.
Disclosure statement
We agree that this article is original, is not being considered for publication elsewhere, and it is approved by all authors. There is no conflict of interest.
Data availability statement
The data that support the findings of this study are available from the corresponding author, [Mohammad Rokouei], upon reasonable request.
References
- Aggrey S, Karnuah AB, Sebastian B, Anthony NB. 2010. Genetic properties of feed efficiency parameters in meat-type chickens. Genet Sel Evol. 42:25.
- Aggrey SE, Marks HL. 2002. Analysis of censored survival data in Japanese quail divergently selected for growth and their control. Poult Sci. 81(11):1618–1620.
- Aggrey SE, Cheng KM. 1992. Estimation of genetic parameters for body weight traits in squab pigeons. Genet Sel Evol. 24(6):553–559.
- Aghazadeh Bokat M, Alijani S, Janmohammadi H, Rafat SA, Alizadeh A, Bostanchi P. 2014. Genetic study of egg production trait of Azerbaijan's native chickens by multi-trait and random regression models. Livest Res Rural Dev. 26 (5):1–11.
- Caetano G. d C, Mota RR, da Silva DA, de Oliveira HR, Viana JMS, de Siqueira OHGBD, Freitas PHF, e Silva FF. 2017. Bayesian estimation of genetic parameters for individual feed conversion and body weight gain in meat quail. Livest Sci. 200:76–79.
- Cloete SWP, Misztal I, Olivier JJ. 2009. Genetic parameters and trends for lamb survival and birth weight in a Merino flock divergently selected for multiple rearing ability. J Anim Sci. 87(7):2196–2208.
- Daikwo SI, Dim NI, Momoh OM. 2013. Inheritance of egg quality traits in Japanese quail. Int J Poult Sci. 12 (3):153–156.
- Ebrahimi K, Dashab GH, Faraji-Arough H, Rokouei M. 2019. Estimation of additive and non-additive genetic variances of body weight in crossbreed populations of the Japanese quail. Poult Sci. 98(1):46–55.
- Finco EM, Marcato SM, Furlan AC, Rossi RM, Grieser D. d O, Zancanela V, Oliveira T. M M d, Stanquevis CE, Universidade Estadual de Maringá, Brazil. 2016. Adjustment of four growth models through Bayesian inference on weight and body nutrient depositions in laying quail. R Bras Zootec. 45(12):737–744.
- Ghorbani S, Tahmoorespur M, Maghsoudi A, Abdollahi-Arpanahi R. 2013. Estimates of (co)variance components for production and reproduction traits with different models in Fars native fowls. Livest Sci. 151(2–3):115–123.
- Gianola D, Foulley JL. 1983. Sire evaluation for ordered categorical data with a threshold model. Genet Sel Evol. 15(2):201–224.
- Gotuzzo AG, Piles M, Della-Flora RP, Germano JM, Reis JS, Tyska DU, Dionello NJL. 2019. Bayesian hierarchical model for comparison of different nonlinear function and genetic parameter estimates of meat quails. Poult Sci. 98(4):1601–1609.
- Hagger C, Hofer A. 1990. Genetic analysis of calving traits in the swiss black and white Braunvieh and Simmental breed by REML and MAPP procedures. Livest Prod Sci. 24(2):93–107.
- Hatcher S, Atkins KD, Safari E. 2010. Lamb survival in Australian merino sheep: a genetic analysis. J Anim Sci. 88(10):3198–3205.
- Kamanli S. 2019. Estimation of genetic parameters for some performance traits in a selected Barred Rock line. Ankara Üniv Vet Fak Derg. 66:391–396.
- Kaplan S, Narinc D, Gürcan EK. 2016. Genetic parameter estimates of weekly body weight and Richard’s growth curve in Japanese quail. Europ Poult Sci. 80:1–10.
- Kgwatalala PM, Molapisi M, Thutwa K, Sekgopi B, Selemoge TP, Nsoso SJ. 2016. Egg quality characteristics and phenotypic correlations among egg quality traits in the naked neck, normal and dwarf strains of Tswana chickens raised under intensive management system. Int J Environ Agr Res. 2(8):96–105.
- Khaldari M, Pakdel A, Mehrabani Yegane H, Nejati Javaremi A, Berg P. 2010. Response to selection and genetic parameters of body and carcass weights in Japanese quail selected for 4-week body weight. Poult Sci. 89(9):1834–1841.
- Manjula P, Park HB, Seo D, Choi N, Jin S, Sung Jin Ahn SJ, Kang Nyeong Heo K, Bo Seok Kang BS, Jun-Heon Lee JH. 2018. Estimation of heritability and genetic correlation of body weight gain and growth curve parameters in Korean native chicken. Asian-Austral J Anim Sci. 31(1):26–31.
- McCullough DA, DeLorenzo MA. 1996. Effects of price and management level on optimal replacement and insemination decisions1. J Dairy Sci. 79(2):242–253.
- Misztal I, Tsuruta S, Strabel T, Auvray B, Druet T, Lee DH. 2002. BLUPF90 and related programs (BGF90). 7th World Congress on Genetics Applied to Livestock Production; Aug 19-23; Montpellier, France: Institut National de la Recherche Agronomique (INRA).
- Moghadam HK, McMillan I, Chambers JR, Julian RJ, Tranchant CC. 2005. Heritability of sudden death syndrome and its associated correlations to ascites and body weight in broilers. Br Poult Sci. 46(1):54–57.
- Mohammadi-Tighsiah A, Maghsoudi A, Bagherzadeh-Kasmani F, Rokouei M, Faraji-Aroughm H. 2018. Bayesian analysis of genetic parameters for early growth traits and humoral immune responses in Japanese quail. Livest Sci. 216:197–202.
- Momoh OM, Gambo D, Dim NI. 2014. Genetic parameters of growth, body, and egg traits in Japanese quails (Cotournix cotournix japonica) reared in southern guinea savannah of Nigeria. J App Bioscience. 79(1):6947–6954.
- N’Dri AL, Mignon-Grasteau S, Sellier N, Tixier-Boichard M, Beaumont C. 2006. Genetic relationships between feed conversion ratios, growth curve and body composition in slow growing chickens. Br Poult Sci. 47(3):273–280.
- National Research Council. 1994. Nutrient requirement of poultry. 9th ed. Washington (DC): National Academy Press.
- Ojo V, Ayorinde KL, Fatoki HO. 2010. Relationship between body weight and some egg production trait in the Japanese quail (Coturnix coturnix japonica). Niseb J. 11(1):89–94.
- Okamoto S, Kobayashi S, Matsuo T. 1989. Feed conversion to body weight gain and egg production in large and small Japanese quail lines selected for 6-Week body weight. Jpn Poult Sci. 26 (4):227–234.
- Ozsoy AN. 2019a. Egg and chick quality characteristics of meat type Japanese quail (Coturnix coturnix japonica) line by canonical correlation analysis. Fresen Environ Bull. 28(4):2582–2588.
- Ozsoy AN. 2019b. The genetic parameters of weight gain and feed efficiency of Japanese quails (Coturnix coturnix japonica) under Tenebrio molitor L and control nutritional environments. Fresen Environ Bull. 28(3):2115–2120.
- Ozsoy AN. 2019c. Genetic parameter estimations of Bayesian hierarchical linear and nonlinear growth curves in Japanese quails. Fresen Environ Bull. 28 (9):6883–6889.
- Ozsoy AN, Aktan JS. 2011. Estimation of genetic for body weight and egg weight trait in Japanese quail. Trend Anim Vet Sci J. 2(1):17–20.
- Pollock KH, Moore CT, Davidson WR, Kellogg FE, Doster GL. 1989. Survival rates of bobwhite quail based on band recovery analyses. J Wildl Manage. 53(1):1–6.
- Puigcerver M, Gallego S, Rodriguez-Teijeiro JD, Senar JC. 1992. Survival and mean life span of the quail Coturnix coturnix. Bird Study. 39(2):120–123.
- Riggio V, Bishop C, Finocchiaro R. 2005. Genetic analysis of early lamb survival in extensively reared lambs. Ital J Anim Sci. 4(2):73–75.
- Skoglund WC, Tomhave AE. 1949. Relationship between egg weight, initial chick weight and subsequent broiler growth. Delaware Agri Exp Sta Bui. 278.
- Skoglund WC, Seegar KC, Ringrose AT. 1952. Growth of broiler chicks hatched from various sized eggs when reared in competition with each other. Poult Sci. 31(5):796–799.
- Van Raden PM, Wiggans GR. 1995. Productive life evaluations: calculation, accuracy and economic value. J Dairy Sci. 78(3):631–638.
- Varkoohi S, Pakdel A, Moradi Shahr Babak M, Nejati Javaremi A, Kause A, Zaghari M. 2011. Genetic parameters for feed utilization traits in Japanese quail. Poult Sci. 90(1):42–47.
- Varona L, Misztal I, Bertrand JK. 1999. Threshold-linear versus linear-linear analysis of birth weight and calving ease using an animal model: I. Variance component estimation. J Anim Sci. 77(8):1994–2002.
- Wiley WH. 1950. The influence of egg weight on the pre-hatching and post-hatching growth rate of the fowl. 1. Egg weight-embryonic development ratios. Poult Sci. 29(4):570–574.
- Zhang Y, Guo ZB, Xie M, Zhang Z, Hou S. 2017. Genetic parameters for residual feed intake in a random population of Pekin duck. Asian-Australasian. J Anim Sci. 30(2):167–170.