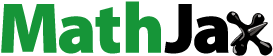
Abstract
This study investigated the effects of maternal linseed supplementation during gestation and lactation on muscle, brain and liver tissues composition and fatty acid (FA) profile in lambs. In a 2 × 2 factorial design, a total of 36 Sarda dairy ewes were fed a control diet (CON, n = 18) or a diet containing linseed (LIN, n = 18) during the last 8 weeks of gestation. After lambing, 9 ewes per group changed to the other diet, moving from CON to LIN and vice-versa. The single-born lambs (n = 36) were reared exclusively on milk and were slaughtered at 4 weeks of age and samples of muscle, brain, and liver tissues were collected. Data were analysed with a general linear model to test the effects of mothers’ gestation and lactation diets, their interaction and the effect of lamb sex. Experimental results evidenced that lambs from mothers fed LIN diet during lactation had a greater content of almost all C18:1 and conjugated linoleic acid (CLA) isomers, both in muscle (P < .01) and in the liver (P < .05), than those from mothers fed CON. Linseed supplementation during gestation generally increased the content of C22:5n3 (P < .01) and C22:6n3 in the brain. In conclusion, experimental results evidenced that the supplementation of ewes’ diet with linseed during lactation strongly affected the muscle and liver FA profile of lambs. The effect of linseed was effective also during gestation, especially on brain tissue, but to a minor extent.
Results of the present work confirm the large impact of maternal diet on the fatty acid composition of lamb tissues
Linseed supplementation during gestation generally increased the content of PUFA n3 in the brain of lambs
Linseed supplementation of mothers during lactation affects the muscle and liver FA profile of sucking lambs
Highlights
Introduction
The quality of the fatty acids (FA) profile of livestock products (milk and meat) is commonly improved by the inclusion of lipid sources in the diet of animals. In particular, the main goal is to increase the proportion of FA considered beneficial for human health, i.e. polyunsaturated FA (PUFA), vaccenic and rumenic acids. Among the lipid sources, linseed is one of the main ones because of its richness in C18:3n3 (alpha-linolenic acid, ALA) (Nudda et al. Citation2020) which increases the content of omega-3 FA in meat and milk (Wachira et al. Citation2002; Doreau and Ferlay Citation2015), since it contains about 40% of oil with 55% of 18:3n3 (Cheng et al. Citation2001; Petit Citation2010; Kouba and Mourot Citation2011). Although several studies investigated the genetic background of milk and meat FA profile in sheep and its improvement through genetic and genomic selection (Rovadoscki et al. Citation2018; Cesarani et al. Citation2019), the manipulation of the diet during pregnancy and lactation appears as an attractive way to program offspring performance in livestock, especially through foetal programming. In fact, there are no doubts about the role of early life environment on programming post-natal life (Tarry-Adkins and Ozanne Citation2011). As pointed out by Barker (Citation1999) in the “fetal programming hypothesis”, also called the “Barker hypothesis”, each adaptation in adult life originates as a consequence of metabolic, cardiovascular and endocrine foetus adaptation to stimuli from foetal environment that prepare itself for postnatal life (Barker Citation1999). Some of these adaptations can be reversible if occur during the period of “plasticity” (Barker Citation1999; Vieau Citation2011) others lead to irreversible body changes that persist in life whenever the intrauterine environmental change persists (Gluckman et al. Citation1990; Gluckman and Hanson Citation2005).
Among ruminants, sheep have been amply studied to investigate the effect of maternal nutrition during early and mid-gestation (Zhou et al. Citation2008; Roque-Jimenez et al. Citation2020; Oviedo-Ojeda et al. Citation2021), end of pregnancy (Elmes et al. Citation2004; Capper et al. Citation2006; Carranza-Martin et al. Citation2018; Coleman et al. Citation2018) or at lambing (Capper et al. Citation2007; Radunz et al. Citation2009; Fisher-Heffernan et al. Citation2015) on the development of foetus and on the programming of offspring performance.
Concerning the role of nutrition on FA composition of milk and meat, particular attention has been posed to the role of some individual components of the maternal diet (omega-3 PUFA, ALA and n-3 long-chain PUFA (LCPUFA), eicosapentaenoic acid (EPA) and docosahexaenoic acid (DHA); Roque-Jiménez et al. Citation2021); which all play a crucial role in several metabolic pathways, being a component of the phospholipids membrane and storing triacylglycerols. The LCPUFA ensure the plasticity and the fluidity of neural cells, some of which, like DHA and 20:4n6 (arachidonic acid, ARA), when included as a supplement in the early life diet of mother and offspring, positively affect the development of the central nervous system (Bazinet and Layé Citation2014; Madore et al. Citation2014).
Maternal nutrition during foetal life is essential, also, for the development of skeletal muscle and hence for the hyperplasic increase in the number of muscular fibres (Zhu et al. Citation2004; Du et al. Citation2010) which represents the goal of meat production; in fact, during postnatal growth, the development of skeletal muscle stops and depends only on the hypertrophic increase of size in native muscle fibre number (Karunaratne et al. Citation2005; Du et al. Citation2010). However, during uterine life, the development of skeletal muscle is particularly vulnerable (Zhu et al. Citation2006) and nutrients are mainly driven to the energy-demanding organs like the brain, heart, and liver (Du et al. Citation2010). In addition to the development, also the composition of the muscle, in terms of muscle FA profile, is affected by the maternal diet (Coleman et al. Citation2018; Shao et al. Citation2020). Indeed, maternal grass or linseed-based diet during pregnancy and lactation improves meat FA profile, in terms of LCPUFA and total conjugated linoleic acid (CLA), in lamb meat (Fusaro et al. Citation2019; Mateo et al. Citation2021), with FA composition similar to that of their mother’s milk (Fusaro et al. Citation2019).
Nutrition during foetal life may have long-term effects on hepatic functions, especially on lipid accumulation, glucose and lactate release and the synthesis of cholesterols (Khanal and Nielsen Citation2017). In the liver, blood non-esterified fatty acids (NEFA) can be oxidised completely to provide energy in form of adenosine triphosphate (ATP) or, partially, leading to the formation of beta-hydroxybutyric acid or cholesterol. Furthermore, FA can also be accumulated in the liver after conversion of NEFA into triglycerides. In addition, there are other important lipid-related metabolic pathways, such as the synthesis of cholesterol and phospholipids. Under mother undernutrition conditions or during the transition period, characterised by a negative energy balance, body fat mobilisation is essential to provide energy. Producing more ATP, through the NEFA oxidation, and reducing the triglyceride synthesis is critical for the health of mother and offspring. In terms of lipid accumulation, some studies evidenced that early life nutrition can modulate the ability of offspring to metabolise excess fat when exposed to a high-fat diet (Hyatt et al. Citation2011). A maternal late pregnancy 18:2n6 diet increased (40%) the 20:4n6 content in lamb foetal liver (Elmes et al. Citation2004). Other studies observed that maternal undernutrition in early and mid-gestation increased the concentration of 20:5n3 and 22:5n3 in liver lamb tissues (Zhou et al. Citation2008). In early gestating ewes, the supplementation with EPA and DHA increased the content of EPA and DHA in lamb liver foetus (Roque-Jimenez et al. Citation2020). Previous works reported an important elongation-desaturation pathway in the liver that leads to the formation of LCPUFA from the substrates linoleic and alpha-linolenic acids, proved by the high concentrations of ARA, docosapentaenoic acid (DPA) and EPA in this organ (da Costa et al. Citation2014; He et al. Citation2015).
The feeding regime in gestation and lactating periods is really important in shaping the FA profile, especially the omega-3 LCPUFA, of brain, liver, and muscle fat. Thus, the aim of this work was to investigate how the supplementation of maternal diet with linseed as the omega-3 source, during feeding in gestation and/or lactation, can affect the fatty acid profile of different tissues in the suckling lambs.
Materials and Methods
All procedures were carried out in accordance with the European Union legislation for the protection of animals used for scientific purposes (2010/63/EU Directive). For this study, no animals were killed for purposes other than slaughter - as such, University ethics approval was also not required. The lambs (i.e. the experimental units of this work) came from a commercial farm and were killed in a commercial slaughterhouse. Animal husbandry and handling procedures were performed in accordance with the local ethics committee, in agreement with the Guidelines for the Use of Laboratory Animals issued by the Italian Ministry of Health.
Experimental design, animals and feeding
All animal procedures (experimental design, animals and feeding) used in this work were previously described by Nudda et al. (Citation2015). Briefly, the experiment was carried out on a private farm located in the north of Sardinia (Italy) and involved 36 multiparous Sarda dairy ewes. The dietary treatments started at 8 weeks before lambing and lasted for the first 4 weeks of lactation. During the last 8 weeks of gestation, pregnant ewes with one foetus were fed a control diet (CON; 18 ewes) or a diet containing linseed (LIN; 18 ewes). After lambing, 9 ewes of each group continued to receive the same diet (i.e. CON or LIN), whereas the other 9 ewes within each group were fed the other diet. Throughout the trial, animals were kept in two groups, with 18 ewes each, according to the diet they were eating (CON and LIN). The graphical representation of the 2 × 2 factorial design is shown in Figure .
Figure 1. Graphical representation of the 2 × 2 experimental design. A total of 36 Sarda dairy ewes were fed a control diet (CON, n = 18) or a diet containing linseed (LIN, n = 18) during the last 8 weeks of gestation. During the first 4 weeks of lactation, 9 ewes changed diet, moving from CON to LIN group and vice-versa.
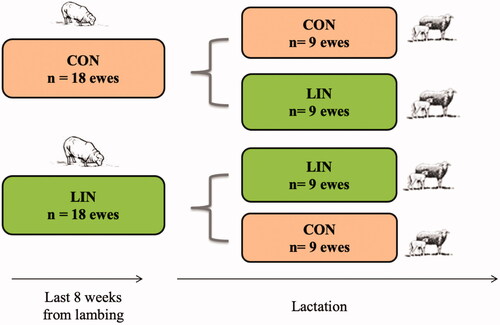
The CON and LIN diets were formulated to meet the energy and protein requirements of late-gestating and lactating ewes using the Small Ruminant Nutrition Model (Tedeschi et al. Citation2010) and consisted of 2 isolipidic, isonitrogenous and isoenergetic pelleted concentrates (Table ). The pelleted concentrate contained the following ingredients: CON = wheat bran, alfalfa meal, distilled from wheat, sunflower extraction meal, corn germ cake, dried sugar beet pulp, calcium carbonate, soybean meal, maize, sodium chloride and sodium bicarbonate, magnesium oxide; LIN = soybean hulls, extruded linseed, wheat bran, sunflower seeds flour, alfalfa meal, dried sugar beet pulp, corn gluten feed, calcium carbonate, soybean meal, maize, sodium chloride, sodium bicarbonate, magnesium oxide. Based on the objective of the study, the two pelleted concentrates differed mainly in terms of FA composition. In the CON diet the most abundant FA was palmitic (16:0), stearic (18:0) and linoleic acid (LA, 18:2n6), being the 31, 27 and 23% of total FA methyl esters (FAME), respectively. Due to the presence of 150 g extruded linseed per kg of the pelleted, in the LIN diet the most abundant FA was the ALA, showing a concentration of 34,2% of FAME, followed by LA and oleic (18:1 cis9) fatty acids (26,3 and 20,6% of FAME, respectively). The daily dose of 150g of extruded linseed was used to supply about 50 g/d of fat per head from this lipid source (Table ).
Table 1. Chemical composition and fatty acid profile of the pelleted concentrates used in the two experimental diets: control (CON) and extruded linseed (LIN).
During both late-gestating and lactating periods, except for the hay (mixed hay fed within the group), all the other ingredients of the diet were individually fed and were completely eaten by the ewes: 1 kg of pelleted concentrate was offered individually (333 g x 3 times per day) with mixed hay and water available ad libitum (the DM content was 910 g/kg, those of CP, NDF, ADL, EE and ash were 80, 550, 180, 16, 80 g/kg of DM, respectively). Water was available ad libitum. As previously reported by Nudda et. (2015), the average individual daily hay intake was estimated at 250 and 1100 g of dry matter (DM) for the last month of gestation and early lactation, respectively. In addition, in order to support the increased energy requirements for foetal growth, 150 g/d of cornflour was supplied during the last 4 weeks of gestation.
At birth, the average lamb body weights were 3.61 and 3.5 for lambs born from ewes fed CON or LIN during gestation, respectively (Nudda et al. Citation2015). The lambs were reared by their dams and therefore ingested exclusively milk until slaughter at d 28 of age.
Tissues sampling
On the day of slaughter, lambs were weighed and then transferred to an authorised commercial abattoir to be slaughtered according to the guidelines of the Directive 2010/63/EU on the protection of animals used for scientific purposes. After exsanguination, the whole Longissimus dorsi (LD) muscles were excised from the right side of each carcase, and the liver and brain were collected. All samples were immediately cooled with liquid nitrogen and then stored at −80 °C until analysis.
Determination of brain, liver, and muscle proximate composition
Moisture, ash, total protein, and fat contents were determined for the considered lamb tissues. About 50 g of each tissue, after 72 h of freeze-drying, were used to determine moisture. Total ash and crude protein (CP) contents were determined according to the Association of Official Analytical Chemists (AOAC) method (Citation2000), whereas total fat content according to Folch et al. (Citation1957).
Fatty acid analysis of the brain, liver and muscle
Lipids were extracted according to Folch et al. (Citation1957) from the freeze-dried liver, brain and LD samples. The FA composition was determined by gas chromatography as previously described by Nudda et al. (Citation2015). Fatty acids were expressed as a proportion of total FA (% of total FA). Groups of FA were calculated considering the same FA profile for all samples: saturated FA (SFA), the sum of the individual saturated FA; monounsaturated FA (MUFA), the sum of the monounsaturated FA; PUFA, the sum of the polyunsaturated FA; PUFAn6, the sum of the PUFA belonging to the n-6 family; PUFAn3, the sum of the PUFA belonging to the n-3 family; other PUFA, the difference between PUFA and the sum of PUFAn3 + PUFAn6; trans-FA (TFA), the sum of the trans-FA, except CLA isomers and t11-C18:1; odd- and branched-chain FA (OBCFA), the sum of odd- and branched-chain FA. In addition, the n-6 to n-3 ratio and the atherogenic (AI) and thrombogenic (TI) indices according to Ulbricht and Southgate (Citation1991) and Nudda et al. (Citation2013) were calculated as follow:
hypocholesterolemic to a hypercholesterolemic ratio (h/H) was calculated according to Fernández et al. (Citation2007) as follows:
In order to estimate the desaturase and elongase activity, some indices were calculated using the product/precursor ratio of the percentage of individual FA as follows: delta-9 desaturase activity (16:1cis9/16:0, D16; 18:1cis9/18:0, D18; C18:2cis-9trans-11/C18:1trans-11, D-CLA); delta-5 desaturase activity (C20:4n6/C20:3n6 D5) and delta-6 desaturase activity (C22:6n3/C22:5n3 D6). The elongase (EL) activity was calculated as EL2n6 = AdA/ARA (C22:4n6/C20:4n6) and EL2n3 = DPA/EPA(C22:5n3/C20:5n3).
In order to estimate elongase and desaturase activities on the C18:3n3 the following ratio were calculated: EPA/ALA = C20:5n3/C18:3n3; DPA/ALA = C22:5n3/C18:3n3; DHA/ALA = C22:6n3/C18:3n3; DHA/EPA = C22:6n3/C20:5n3. The elongase and desaturase activity on the C18:2n6 (LA) was calculated as follow: ARA/LA = C20:4n6/C18:2n6.
Statistical analysis
Each investigated variable (proximate composition and FA) was analysed within tissue using the following 2 × 2 factorial linear model:
where y was the independent variable (i.e. the FA); μ was the general mean; GEST was the fixed effect of the gestation diet of the ewe (2 levels, CON and LIN); LACT was the fixed effect of the lactation diet of the ewe (2 levels, CON and LIN); GEST*LACT was the interaction between GEST and LACT effects; s was the fixed effect of the sex of the lamb (2 levels, male and female); ε was the residual error. Differences in means were declared significant when the P-value of ANOVA test was lower than .05. According to Murtaugh (Citation2014), P-value higher than .05 and lower than .10 were considered suggestive, even if inconclusive. When the diet effect was found to be significant, least-squares means were separated using the Tukey honestly significant difference test (significance declared for P < .05).
A principal component analysis (PCA) was carried out in R software (R Core Team 2020) using prcomp function applied to all samples together (n = 108, 36 lambs × 3 tissues). According to the number of considered FA (twenty-eight sums and ratio), a total of 28 principal components (PC) were extracted. Variables were scaled to have unit variance before the analysis.
Results and discussion
Details about the effect of experimental diets on growth performance and carcase traits of lambs and milk and muscle (LD) FA profile were previously reported by Nudda et al. (Citation2015). Briefly, the bodyweight of lambs at slaughter (∼9–10 kg) did not differ between groups and was almost three times greater than the weight at birth (∼3.5 kg). In addition, lambs born from mothers fed a linseed-based diet during pregnancy showed suggestive lower daily gain and cold carcase weight (Nudda et al. Citation2015).
Proximate composition
The effects of the experimental diets during gestation and lactation and their interaction on the proximate composition of the LD muscle, liver and brain are reported in Table . The inclusion of extruded linseed in the diet of mothers did not affect (P > .05) the chemical composition of lambs’ tissues during gestation. Compared with the CON, LIN supplementation during lactation decreased moisture and increase protein content in muscle (Table ). The sex of lambs did not affect the lamb’s tissues composition (P > .05).
Table 2. Proximate composition of tissues (muscle, brain and liver) of lambs born from Sarda ewes fed a control diet (CON) or a diet containing linseed (LIN) during the last 8 weeks of gestation and for the first 4 weeks of lactation.
Fatty acid composition of Longissimus dorsi muscle
Linseed supplementation during lactation strongly affected the FA profile of LD (Table ). The effect of linseed was effective also during gestation, even though it was significant for a minor number of FA compared to the lactation. Only one minor FA (C18:2cis-9,trans-12) was affected by sex, with larger values found for female lambs (data not shown).
Table 3. Fatty acid composition (% of total fatty acids) in muscle (Longissimus dorsi; LD) of lambs born from Sarda ewes fed a control diet (CON) or a diet containing linseed (LIN) during the last 8 weeks of gestation and for the first 4 weeks of lactation.
In general, the FA composition of the lamb muscles was similar to that of maternal milk, as confirmed by several experiments (Valvo et al. Citation2005; Nudda et al. Citation2015; Fusaro et al. Citation2019). Linseed supplementation during lactation lowered (P < .01) the concentration of C16:0 and C16:1cis9. An opposite pattern was observed for the concentration of most C16:1, C18:1 and the CLA isomers. In particular, the concentration of C18:1trans11 (vaccenic acid, VA) and C18:2cis-9,trans-11 (rumenic acid, RA) increased (P < .01) in muscles of lamb suckling milk from mothers fed linseed during lactation. These FA are mainly derived from the biohydrogenation of dietary FA occurring in the rumen, in particular on C18:3n3 (Chilliard et al. Citation2007). The increase of dietary C18:3n3 leads to a rumen accumulation of its biohydrogenation intermediates, such as vaccenic acid (Correddu et al. Citation2015), that can be also retrieved in milk (Correddu et al. Citation2016) and, in turn, in the muscle of sucking lambs (Bessa et al. Citation2007). In particular, the CLA isomer C18:2cis-9,trans-11, increased in the LIN group during lactation partly because of a direct transfer from milk to muscle and partly from the endogenous synthesis in intramuscular fat by desaturation of C18:1trans11 (vaccenic acid, VA). Consistently, a strong correlation between C18:2cis-9,trans-11 and its precursor C18:1trans11 was already reported (Nudda et al. Citation2019). As expected, the concentration of C18:3n3 increased in the muscle of lambs whose mothers were fed extruded linseed, both during gestation and lactation (P < .05).
Fatty acid profile of muscle was less affected when extruded linseed was included in the mother diet during gestation. The transfer of FA from mother to foetus is regulated by the permeability of the placenta, and a crucial role is played by the fatty acid-binding protein ( FABPpm; Campbell et al. Citation1994; Larqué et al. Citation2013; Jones et al. Citation2014; Desantadina et al. Citation2018). Environmental or animal factors affecting the expression and activity of FABPpm could explain differences among studies. As an example, feeding late gestating dairy cows a diet supplemented with oilseed reduced placental fatty acid transporters expression, likely due to changes in fatty acid-activated transcription factors, such as peroxisome proliferator-activated receptors (PPARs; Salehi and Ambrose Citation2017).
Even though the effect of linseed offered during gestation appeared to be less evident than that of lactation, it was observed a positive effect on the content of C18:1t4 and C18:1c12 and C18:1c14, increased. Among LCPUFAn3, the C20:5n3 (EPA) was increased in muscle by linseed supplementation during gestation (P < .01) and showed a suggestive greater value (P < .10) with linseed supplementation during lactation. On the other hand, the linseed supplementation during gestation reduced or showed a suggestive reduction of the concentration of those belonging to the n-6 family C22:2n6 (P < .01), C22:4n6 (P < .01). The C20:4n6 (ARA; P < .10) was reduced by linseed supplementation only during lactation. It appears that the supplementation of extruded linseed during gestation produced an overall inverse relationship between C18:3n3 and PUFAn6 derivatives. The opposite relationship, between C18:2n6 and PUFAn3 derivatives, has been observed on prepartum cows supplemented with linoleic acid source (Garcia et al. Citation2014). This relationship could be partially explained with a competition among C18:2n6 and C18:3n3 for the Δ6-desaturase in the long chain PUFA conversion pathway in different species.
Fatty acid composition of brain
The inclusion of extruded linseed in the diet during gestation and/or lactation had a weak effect on the fatty acid composition of the lamb’s brain (Table ). Almost all FA in the brain were not influenced by dietary treatments, except for the important LCPUFA and minor FA (C24:0, C16:1t9, and C18:1t10). In particular, the concentrations of C20:3n6, C22:5n3 (DPA) and C22:6n3 (DHA) were increased, whereas C24:0 was decreased in a group with LIN supplementation during gestation. A positive effect of linseed on DPA and DHA was observed also during lactation, suggesting a synergic effect on the brain of lambs whose mothers were fed linseed during both gestation and lactation. This is very important from a nutritional point of view because it highlights and confirms the importance of an adequate supply of essential FA (e.g. ALA and LCPUFAn3) during gestation in animals and humans as well (Allen and Harris Citation2001; Niculescu et al. Citation2011). Indeed, there is an important accumulation of LCPUFA during the last trimester of pregnancy to support the rapid growth and brain development of children (Gibson et al. Citation2011; Harris and Baack Citation2015).
Table 4. Fatty acid composition (% of total fatty acids) of the brain of lambs born from Sarda ewes fed a control diet (CON) or a diet containing linseed (LIN) during the last 8 weeks of gestation and for the first 4 weeks of lactation.
The ARA and DHA make up a considerable portion (more than 70%) of the LCPUFA in the lamb brain tissues (about 30 and 40%, respectively). As pointed out by Rajion et al. (Citation1985), considering their low concentrations, at trace levels, in maternal blood and milk lipid fractions (Nudda et al. Citation2015), they are unlikely to be derived directly from the maternal unesterified FA. Then, these FA are more likely to be synthesised, through intense elongase and desaturase activities, from linoleic (C18:2n6) or linolenic (C18:3n3) acid either in the placental or foetal tissues, or both, as suggested by the studies of Shand and Noble (Citation1979, Citation1981). The increase in DHA concentration and the lack of effect on ARA, are consistent with the greater supply of C18:3n3 in lambs nutrition, due to the inclusion of extruded linseed in the diet of mothers.
Although the diet was not able to affect the concentration of OBCFA (P > .05), the high concentration of these FA in brain tissues was quite surprising. Indeed, the mean values of the sum of OBCFA were 3.91 ± 0.32, in the brain, mainly ascribed to the concentration of a fatty acid that has been identified as isoC16:0 (3.18 ± 0.61, mean ± SD). In humans branched-chain fatty acids could be considered valuable markers of dairy fat intake. In fact, OBCFA is mainly of microbial origin and their concentrations in milk have often been used as a proxy of the ruminal microbial activity, also in response to different diets (Vlaeminck et al. Citation2006). However, suckling lambs can be considered functionally non-ruminant (Lane et al. Citation2000; Osorio et al. Citation2007), and the concentration could be partly derived from suckled milk. The OBCFA can be also produced endogenously by mammals, when propionyl-CoA and methyl-malonyl-CoA are used as substrates for the FA synthesis instead of acetyl-CoA and malonyl-CoA, respectively (Dewulf et al. Citation2019). Accumulation of OBCFA in sheep tissues, including the brain, has been related to the Cobalt-Vitamin B12 deficiency (Kennedy et al. Citation1994), often associated with a Cobalt-deficient diet. Considering that the concentration of OBCFA in the lamb brains was not affected (P > .05) by the mother diet, we can exclude that our nutritional treatment was involved. Then, further investigation should be conducted to highlight the reasons for the high concentration of these FA in the brain (in particular of isoC16:0) retrieved in the present work.
Few FA were affected by sex, with C17:1cis9 and C18:1cis9 higher in males, whereas C18:1t4, C18:3n4, C20:4n6, C22:4n6, and C24:0 higher in females (data not shown). In general, the effect of sex on FA composition differs greatly among works (Tejeda et al. Citation2008), making difficult the interpretation of the results. Even if molecular mechanisms have still not been completely elucidated, differences are likely attributable to the sex hormones that can influence the fatty acid metabolism and the related gene expression (Starčević et al. Citation2017).
Fatty acid composition of liver
Regarding the FA profile of the liver (Table ), it was possible to observe that linseed supplementation during lactation strongly affected the liver FA profile of lambs. The effect was more evident compared to that on muscle FA profile. In general, the effect of linseed offered during gestation was weaker than that of lactation. In addition, only two minor FA were affected by the sex of the lambs: C18:1c12 and C20:2n6, which were both higher in females (data not shown). The linseed supplementation during lactation did not affect the concentration of short-chain fatty acids (SCFA) and medium-chain fatty acids (MCFA), except for that C16:0 that decreased (P < .01) and for some isomers of C16:1, especially the trans-palmitoleic acid (C16:1 trans9), that exhibited an opposite trend. The positive effect of linseed on C16:1 trans9 could be of great interest, for promising health benefits highlighted recently by Guillocheau et al. (Citation2020). The linseed offered during lactation affected also the odd C17:0 and branched anteisoC17:0 that increased (P < .01). The amount of almost all the identified trans and cis C18:1 isomers increased with linseed supplement. In particular, the linseed fed during lactation increased the proportion of VA (value in LIN 2–3-fold greater than CON group), confirming the results observed in muscle samples. Also, almost all identified isomers of CLA were greater in groups receiving linseed during lactation, with the c9,t11-CLA being the most abundant isomer in both groups. From these results, it appears clear that the variation of these liver FA in lambs responds to the diet of mothers during the suckling period. Interestingly the high differences compared to the control group were observed when LIN was included in the diet of the mothers only post-partum. It should be noticed that some effects can be ascribed to the different forage to concentrate ratios between the diets offered during the prenatal or postnatal period, as reported in the previous work (Nudda et al. Citation2015).
Table 5. Fatty acid composition (% of total fatty acids) of the liver of lambs born from Sarda ewes fed a control diet (CON) or a diet containing linseed (LIN) during the last 8 weeks of gestation and for the first 4 weeks of lactation.
The concentration of C18:3n3 in lactation was almost doubled (P < .05) in the LIN compared to the CON group, whereas no effect on LCPUFAn3 was detected. Feeding lambs with protected linseed enhanced both liver concentrations of C18:3n3 and LCPUFAn3 (Demirel et al. Citation2004; Kim et al. Citation2007), whereas supplementing Australian prime lambs with linseed oils did not change C18:3n3 but increased LCPUFAn3 concentration (Nguyen et al. Citation2017). Differences between studies could be related to intake of linseed directly from lambs rather than conveyed through mothers' milk, as in our trial. Consequently, the intake of C18:3n3 by lambs belonging to the different experiments should be very different. Considering an average of 1.2 kg/day of suckled milk with 4.1% of fat and 2.36% of C18:3n3 on total fat (Nudda et al. Citation2015), the estimated 1.2 g/d of C18:3n3 intake was markedly lower than 25 g/d in the experiment of Nguyen et al. (Citation2017). In the present study, the low hepatic ALA concentration (0.71 g/100g FA) and the relatively high LCPUFAn3 concentration (2.99 g/100g FA) were in line with a previous study on cattle (da Costa et al. Citation2014) and could be explained by highly active desaturation and elongation activities on ALA acid occurring in this organ, that can be considered important for the biosynthesis of LCPUFAn3.
Multivariate analysis comparison of tissues
Multivariate statistical analysis has been demonstrated to be a useful approach to reduce the complexity of data; for this reason, this statistical analysis has shown interesting applications in the study of the FA composition in different matrices. In particular, PCA is used to reduce the variance of the system in a smaller number of new variables (extracted principal components), and it is particularly useful to cluster observations based on the correlation with the new variables (Correddu et al. Citation2021). Application of PCA on the FA profile of lamb meat allowed to differentiate lambs reared in different forage systems (Ye et al. Citation2020). Recently, in cattle, PCA allowed a clear separation among tissues, based exclusively on their FA profile and contributed to highlighting differences in lipid metabolism (da Costa et al. Citation2014).
Results of the principal component analysis carried out in the present work are reported in Table and Figure . The first 5 extracted PC accounted for 90% of the total variance (Table ). PC1, which explained 45% of the total variance, showed a clear separation between the brain, at negative values, and muscle and liver, at positive values (Figure ). The meaning of the distinction between brain samples and that of liver and muscle, highlighted by the PC1 scores, should be searched in the separation operated by the same PC on the original variables (Table and Figure ). In particular, PC1 showed larger negative values for the elongase and desaturase indices and, among FA groups, for PUFAn3 and OBCFA; on the contrary, PC1 showed the largest positive values for n6/n3 and MUFA. These clusterizations are associated with the different values of these variables in the considered tissues. Brain tissue showed a lower value of n6/n3 compared to both liver and muscle. On the contrary, for the variables with the most negative loadings for PC1 (i.e. ARA/LA and DHA/EPA) brain showed larger values compared to the other two considered tissues. Thus, variables with positive loadings for PC1 (i.e. n6/n3) showed low values for the brain and high for liver and muscle, whereas the variables with negative loadings for PC1 (i.e. ARA/LA and DHA/EPA) showed the opposite pattern. This was confirmed also by the correlation between PC1 scores and the amount of the considered variables: n6/n3 had a positive correlation (0.86), whereas ARA/LA and DHA/EPA had negative correlations (both −0.94). In general, these results indicate that the largest differences among tissues are related to the metabolism of the FA, specifically by the elongase and desaturase activities. In particular, the highest activity of these enzymatic pathways can be retrieved in the brain, whereas the lowest (or no activity) in muscle, with the liver being intermediate. This result is in accordance with the typical high concentration of LCPUFA and, in addition, to the low content of alpha-linolenic acid in brain tissue and agrees to a previous report on brain FA composition in lambs (Celik et al. Citation1999).
Figure 2. The plot of the scores (A) and loadings (B) for the first and second principal components. The plot of the scores (C) and loadings (D) for the first and third principal components. Different shapes indicate samples belonging to different tissues . TFA = sum of the individual trans-FA, except CLA isomers and t11-C18:1; SFA = sum of the individual saturated FA; MUFA = sum of the individual monounsaturated FA; PUFA, sum of the individual polyunsaturated FA; PUFAn3, sum of the PUFA belonging to the n3 family; PUFAn6 sum of the PUFA belonging to the n6 family; OBCFA = sum of individual odd- and branched-chain FA; n6/n3 = ratio between PUFAn6 and PUFAn3; AI = aterogenic index; TI trombogenic index; HPPUFA: high polyunsaturated PUFA; h/H hypocholesterolemic to hypercholesterolemic ratio; delta-5ARA = index of delta-5 desaturase activity calculated as: ARA/DGLN (arachidonic acid, C20:4n6/dihomo-gamma-linolenic acid, C20:3n6); delta-9 C16 = index of delta-9 desaturase activity calculated as: C16:1cis9/C16:0; delta-9 C18 = index of delta-9 desaturase activity calculated as: C18:1cis9/C18:0; delta-9 CLA = index of delta-9 desaturase activity calculated as: C18:2cis-9trans-11/C18:1trans-11; EL2n6 = elongase activity of elovl2 calculated as: AdA/ARA (adrenic acid, C22:4n6/arachidonic acid, C20:4n6); EL2n3 = elongase activity of elovl2 calculated as: DPA/EPA (docosapentaenoic acid, C22:5n3/eicosapentaenoic acid, C20:5n3); ALA = alpha-linolenic acid; DHA = docosahexaenoic acid (C22:6n3); delta-6 DHA = delta-6 desaturase activity calculated as: DHA/DPA (docosahexaenoic acid, C22:6n3/docosapentaenoic acid, C22:5n3); LA = linoleic acid.
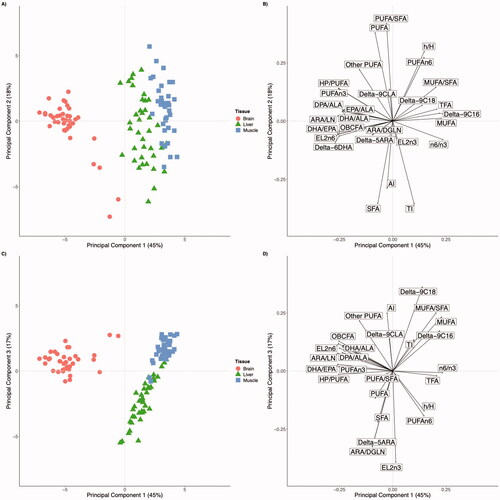
Table 6. Loadings of the first 5 principal components (PC) extracted from the 30 considered variables. Standard deviations, proportion of variance (i.e. eigenvalue) and cumulative proportion of reported PC
PC2 accounted for 18% of the total variance; SFA, TI and AI showed the lowest value, whereas PUFA and PUFA/SFA were the highest ones (Table ). Thus, PC2 can be considered associated with the quality of FA composition from a nutritional point of view. Indeed, a high concentration of PUFA and high values of PUFA/SFA and h/H ratios are associated with a healthier fatty acid profile. No clear pattern can be observed among tissues according to PC2 scores (Figure ), in agreement with the slight differences observed in the amount of these FA in the three considered tissues. However, it could be argued that this PC may be related to the different diets involved in this study: PC2 scores of lambs born from ewes fed CON or LIN during lactation were significantly different with Tukey HSD test (P < .05), whereas no differences were observed in gestation. Moreover, the pseudo-correlation between GEST diet and PC2 scores (i.e. the correlation between observed scores and predicted ones) was 0.26. Based on these considerations, it can be pointed out that, irrespective of the tissue, lambs from mother fed LIN during lactation showed a better FA profile (i.e. the greater amount of PUFA, greater ratio PUFA/SFA, and lower values of AI and TI) compared to that from CON group. This was expected and in agreement with the large literature existing on the positive effects of the linseed dietary inclusion on the ruminant’s tissues FA profile (Petit Citation2010; Nudda et al. Citation2020).
PC3, which explains 15% of the total variance (Table ), had large positive values for PUFA, MUFA, AI, MUFA/SFA and delta-9C18; large negative values for PC3 were observed for SFA, ARA/DGLN Delta-5ARA and EL2n3 (Table ). The PC3 was able to separate brain and muscle (with positive scores) from liver (with negative scores) tissue samples (Figure ).
An interesting result can be observed by looking at the plot of the scores (Figure ) and loadings (Figure ) of the PC1 and PC3 that allows separating the three tissues: the brain has negative values for PC1 and positive for PC3, muscle has positive values for both PC1 and PC3, and liver has positive values for PC1 and negative for PC3. In general, it appears that the liver differs from muscle and brain for a larger amount of SFA, but also for a greater elongase activity of elovl2 (in particular on the PUFAn3), calculated as a ratio between DPA/EPA (C22:5n3/C20:5n3), and delta-5 desaturase activity calculated as ARA/DGLA (C20:4n6/C20:3n6) ratio; muscle differs from the other tissues for the greater delta-9 desaturase activity on the C16:0 and C18:0 to produce their corresponding monounsaturated cis-9 isomers (C16:1cis-9 and C18:1cis-9, respectively) and consequently showed high values for MUFA concentration and for a greater MUFA/SFA ratio. On the other hand, as above described for PC1, the brain differs from the other tissues for the greater activity of elovl2 (in particular on the PUFAn6), calculated as a ratio between adrenic acid (AdA) and ARA (C22:4n6/C20:4n6), OBCFA and PUFAn3 concentration, and for the greater activity of the desaturase and elongase activity occurring on the alpha-linolenic acid (calculated as EPA/ALA, DHA/ALA, DPA/ALA).
Conclusions
Results of the present work confirm the large impact of maternal diet on the fatty acid composition of lamb tissues, and the large effect of extruded linseed fed during lactation in improving the concentration of beneficial fatty acids at muscle and liver tissues level. The effect of linseed was effective also during gestation, especially on brain tissue. The “memory effect” of linseed supplementation during gestation was particularly evident in the fatty acid composition of lamb brain, which showed, compared to the control group, increased values of long-chain polyunsaturated fatty acids. The principal component analysis allowed to differentiate the three considered tissues, based on the metabolism occurring on the polyunsaturated fatty acids. Brain differs from the other tissues for the high elongase and desaturase activities on linoleic (C18:2n6) or linolenic (C18:3n3) acids. The liver differs from muscle and brain for a larger amount of SFA, but also for a greater elongase activity on PUFAn3, and delta-5 desaturase activity; muscle differs from the other tissues for the greater delta-9 desaturase activity. Furthermore, irrespective of the tissues, lambs whose mothers were fed extruded linseed both during gestation and lactation periods evidenced a better FA profile compared to the control.
Acknowledgments
The authors thank Mrs. Antonio Fenu and Roberto Rubattu for their technical assistance. The authors gratefully acknowledge Cargill (Animal Nutrition Division, Milan, Italy) for providing the dietary ingredients for this study.
Disclosure statement
The authors declare that there is no conflict of interest associated with the paper.
Data availability statement
Data used for this study are available from the corresponding author upon reasonable request.
Additional information
Funding
References
- Allen KG, Harris MA. 2001. The role of n-3 fatty acids in gestation and parturition. Exp Biol Med. 226(6):498–506.
- Association of Official Analytical Chemists (AOAC) 2000. Meat and meat products, in official methods of analysis, 17th edition. Gaithersburg, MD, USA: AOAC.
- Barker DJ. 1999. Fetal origins of cardiovascular disease. Ann Med. 31( sup1):3–6.
- Bazinet RP, Layé S. 2014. Polyunsaturated fatty acids and their metabolites in brain function and disease. Nat Rev Neurosci. 15(12):771–785.
- Bessa RJ, Alves SP, Jerónimo E, Alfaia CM, Prates JA, Santos‐Silva J. 2007. Effect of lipid supplements on ruminal biohydrogenation intermediates and muscle fatty acids in lambs. Eur J Lipid Sci Technol. 109(8):868–878.
- Campbell FM, Gordon MJ, Dutta-Roy AK. 1994. Plasma membrane fatty acid-binding protein (FABPpm) of the sheep placenta. Biochim Biophys Acta, Lipids Lipid Metab. 1214(2):187–192.
- Capper JL, Wilkinson RG, Mackenzie AM, Sinclair LA. 2006. Polyunsaturated fatty acid supplementation during pregnancy alters neonatal behavior in sheep. J Nutr. 136(2):397–403.
- Capper JL, Wilkinson RG, Mackenzie AM, Sinclair LA. 2007. The effect of fish oil supplementation of pregnant and lactating ewes on milk production and lamb performance. Animal. 1(6):889–898.
- Carranza-Martin AC; Coleman DN, Garcia LG, Furnus CC, Relling AE. 2018. Prepartum fatty acid supplementation in sheep. III. Effect of eicosapentaenoic acid and docosahexaenoic acid during finishing on performance, hypothalamus gene expression, and muscle fatty acids composition in lambs. J Anim Sci. 96(12):5300–5310.
- Celik S, Yilmaz Ö, Aşan T, Naziroğlu M, Cay M, Aksakal M. 1999. Influence of dietary selenium and vitamin E on the levels of fatty acids in brain and liver tissues of lambs. Cell Biochem Funct. 17(2):115–121.
- Cesarani A, Gaspa G, Correddu F, Cellesi M, Dimauro C, Macciotta NPP. 2019. Genomic selection of milk fatty acid composition in Sarda dairy sheep: effect of different phenotypes and relationship matrices on heritability and breeding value accuracy. J Dairy Sci. 102(4):3189–3203.
- Cheng Z, Robinson RS, Pushpakumara PGA, Mansbridge RJ, Wathes DC. 2001. Effect of dietary polyunsaturated fatty acids on uterine prostaglandin synthesis in the cow. J Endocrinol. 171(3):463–474.
- Chilliard Y, Glasser F, Ferlay A, Bernard L, Rouel J, Doreau M. 2007. Diet, rumen biohydrogenation and nutritional quality of cow and goat milk fat. Eur J Lipid Sci Technol. 109(8):828–855.
- Coleman DN, Rivera-Acevedo KC, Relling AE. 2018. Prepartum fatty acid supplementation in sheep I. Eicosapentaenoic and docosahexaenoic acid supplementation do not modify ewe and lamb metabolic status and performance through weaning. J Anim Sci. 96(1):364–374.
- Correddu F, Cesarani A, Dimauro C, Gaspa G, Macciotta NP. 2021. Principal component and multivariate factor analysis of detailed sheep milk fatty acid profile. J Dairy Sci. 104(4):5079–94.
- Correddu F, Gaspa G, Pulina G, Nudda A. 2016. Grape seed and linseed, alone and in combination, enhance unsaturated fatty acids in the milk of Sarda dairy sheep. J Dairy Sci. 99(3):1725–1735.
- Correddu F, Nudda A, Battacone G, Boe R, Francesconi AHD, Pulina G. 2015. Effects of grape seed supplementation, alone or associated with linseed, on ruminal metabolism in Sarda dairy sheep. Anim Feed Sci Technol. 199:61–72.
- da Costa ASH, Bessa RJB, Pires VMR, Rolo EA, Pinto RMA, Fontes CMGA, Prates JAM. 2014. Is hepatic lipid metabolism of beef cattle influenced by breed and dietary silage level? BMC Vet Res. 10:65.
- Demirel G, Wachira AM, Sinclair LA, Wilkinson RG, Wood JD, Enser M. 2004. Effects of dietary n-3 polyunsaturated fatty acids, breed and dietary vitamin E on the fatty acids of lamb muscle, liver and adipose tissue. Br J Nutr. 91(4):551–565.
- Desantadina R, Quintana S, Recavarren MI, Relling AE. 2018. Effect of time of gestation on fatty acid transporters mRNA expression in bovine placenta. Biosci J. 34:180–185.
- Dewulf JP, Gerin I, Rider MH, Veiga-da-Cunha M, Van Schaftingen E, Bommer GT. 2019. The synthesis of branched-chain fatty acids is limited by enzymatic decarboxylation of ethyl- and methylmalonyl-CoA. Biochem J. 476(16):2427–2447.
- Doreau M, Ferlay A. 2015. Linseed: a valuable feedstuff for ruminants. OCL. 22(6):D611.
- Du M, Tong J, Zhao J, Underwood KR, Zhu M, Ford SP, Nathanielsz PW. 2010. Fetal programming of skeletal muscle development in ruminant animals. J Anim Sci. 88(13 Suppl):E51–E60.
- Elmes M, Tew P, Cheng Z, Kirkup SE, Abayasekara DRE, Calder PC, Hanson MA, D. Claire Wathes, Burdge GC. 2004. The effect of dietary supplementation with linoleic acid to late gestation ewes on the fatty acid composition of maternal and fetal plasma and tissues and the synthetic capacity of the placenta for 2-series prostaglandins. Biochim Biophys Acta. 1686(1–2):139–147.
- Fernández M, Ordóñez JA, Cambero I, Santos C, Pin C, de la Hoz L. 2007. Fatty acid compositions of selected varieties of Spanish dry ham related to their nutritional implications. Food Chem. 101(1):107–112.
- Fisher-Heffernan, Rebecca E, Or'Rashid, Mamun M, AlZahal, Ousama, Quinton, Margaret, Boermans, Herman J, McBride, Brian W, Regnault, Timothy R H, Karrow, Niel A. 2015. Fishmeal supplementation during ovine pregnancy and lactation protects against maternal stress-induced programming of the offspring immune system. BMC Vet Res. 11:266.
- Folch J, Lees M, Stanley GS. 1957 A simple method for the isolation and purification of total lipides from animal tissues. J Biol Chem. 226(1):497–509.
- Fusaro I, Giammarco M, Chincarini M, Odintsov Vaintrub M, Palmonari A, Mammi LME, Formigoni A, Di Giuseppe L, Vignola G. 2019. Effect of ewe diet on milk and muscle fatty acid composition of suckling lambs of the protected geographical origin Abbacchio Romano. Animals. 10(1):25.
- Garcia, M, Greco, L F, Favoreto, M G, Marsola, R S, Martins, L T, Bisinotto, R S, Shin, J H, Lock, A L, Block, E, Thatcher, W W, Santos, J E P. 2014. Effect of supplementing fat to pregnant nonlactating cows on colostral fatty acid profile and passive immunity of the newborn calf. J Dairy Sci. 97(1):392–405.
- Gibson RA, Muhlhausler B, Makrides M. 2011. Conversion of linoleic acid and alpha‐linolenic acid to long‐chain polyunsaturated fatty acids (LCPUFAs), with a focus on pregnancy, lactation and the first 2 years of life. Matern Child Nutr. 7:17–26.
- Gluckman PD, Breier BH, Oliver M, Harding J, Bassett N. 1990. Fetal growth in late gestation – a constrained pattern of growth. Acta Paediatr. 79(s367):105–110.
- Gluckman P, Hanson M. 2005. The foetal matrix: evolution, development and disease. Cambridge University Press: Cambridge.
- Guillocheau E, Legrand P, Rioux V. 2020. Trans-palmitoleic acid (trans-9-C16:1, or trans-C16:1 n-7): Nutritional impacts, metabolism, origin, compositional data, analytical methods and chemical synthesis. A review. Biochimie. 169:144–160.
- Harris WS, Baack ML. 2015. Beyond building better brains: bridging the docosahexaenoic acid (DHA) gap of prematurity. J Perinatol. 35(1):1–7.
- He M, Jia J, Yang W. 2015. Comparison on the fatty acid profiles of liver, subcutaneous fat and muscle from feedlot steers finished on diets supplemented with or without cinnamaldehyde or monensin. J Sci Food Agric. 95(3):576–582.
- Hyatt MA, Gardner DS, Sebert S, Wilson V, Davidson N, Nigmatullina Y, Chan LLY, Budge H, Symonds ME. 2011. Suboptimal maternal nutrition, during early fetal liver development, promotes lipid accumulation in the liver of obese offspring. Reproduction. 141(1):119–126.
- Jones ML, Mark PJ, Waddell BJ. 2014. Maternal dietary omega-3 fatty acids and placental function. Biol Reprod. 147:143–152.
- Karunaratne JF, Ashton CJ, Stickland NC. 2005. Fetal programming of fat and collagen in porcine skeletal muscles. J Anat. 207(6):763–768.
- Kennedy DG, Kennedy S, Blanchflower WJ, Scott JM, Weir DG, Molloy AM, Young PB. 1994. Cobalt-vitamin B12 deficiency causes accumulation of odd-numbered, branched-chain fatty acids in the tissues of sheep. Br J Nutr. 71(1):67–76.
- Khanal P, Nielsen MO. 2017. Impacts of prenatal nutrition on animal production and performance: a focus on growth and metabolic and endocrine function in sheep. J Anim Sci Biotechnol. 8:1–14.
- Kim SC, Adesogan AT, Badinga L, Staples CR. 2007. Effects of dietary n-6: n-3 fatty acid ratio on feed intake, digestibility, and fatty acid profiles of the ruminal contents, liver, and muscle of growing lambs. J Anim Sci. 85(3):706–716.
- Kouba M, Mourot J. 2011. A review of nutritional effects on fat composition of animal products with special emphasis on n-3 polyunsaturated fatty acids. Biochimie. 93(1):13–17.
- Lane MA, Baldwin RT, Jesse BW. 2000. Sheep rumen metabolic development in response to age and dietary treatments. J Anim Sci. 78(7):1990–1996.
- Larqué E, Ruiz-Palacios M, Koletzko B. 2013. Placental regulation of fetal nutrient supply. Curr Opin Clin Nutr Metab Care. 16(3):292–297.
- Madore C, Nadjar A, Delpech JC, Séré A, Aubert A, Portal C, Joffre C, Layé S. 2014. Nutritional n-3 PUFAs deficiency during perinatal periods alters brain innate immune system and neuronal plasticity-associated genes. Brain Behav Immun. 41:22–31.
- Mateo L, Ortuño J, Bañón S. 2021. Ewe's diet during gestation and lactation affects ready-to-eat meat from light lambs. Animal. 15(2):100043).
- Murtaugh PA. 2014. In defense of P values. Ecology. 95(3):611–617.
- Nguyen DV, Le VH, Nguyen QV, Malau-Aduli BS, Nichols PD, Malau-Aduli AE. 2017. Omega–3 long-chain fatty acids in the heart, kidney, liver and plasma metabolite profiles of australian prime lambs supplemented with pelleted canola and flaxseed oils. Nutrients. 9(8):893.
- Niculescu MD, Lupu DS, Craciunescu CN. 2011. Maternal α-linolenic acid availability during gestation and lactation alters the postnatal hippocampal development in the mouse offspring. Int J Dev Neurosci. 29(8):795–802.
- Nudda A, Atzori AS, Boe R, Francesconi AHD, Battacone G, Pulina G. 2019. Seasonal variation in the fatty acid profile in meat of Sarda suckling lambs. Ital J Anim Sci. 18(1):488–497.
- Nudda A, Battacone G, Bee G, Boe R, Castanares N, Lovicu M, Pulina G. 2015. Effect of linseed supplementation of the gestation and lactation diets of dairy ewes on the growth performance and the intramuscular fatty acid composition of their lambs. Animal. 9(5):800–809.
- Nudda A, Battacone G, Boe R, Manca MG, Rassu SPG, Pulina G. 2013. Influence of outdoor and indoor rearing system of suckling lambs on fatty acid profile and lipid oxidation of raw and cooked meat. Ital J Anim Sci. 12(4):e74.
- Nudda A, Cannas A, Correddu F, Atzori AS, Lunesu MF, Battacone G, Pulina G. 2020. Sheep and goats respond differently to feeding strategies directed to improve the fatty acid profile of milk fat. Animals. 10(8):1290.
- Osorio MT, Zumalacárregui JM, Figueira A, Mateo J. 2007. Fatty acid composition in subcutaneous, intermuscular and intramuscular fat deposits of suckling lamb meat: effect of milk source. Small Rumin Res. 73(1–3):127–134.
- Oviedo-Ojeda MF, Roque-Jiménez JA, Whalin M, Lee-Rangel HA, Relling AE. 2021. Effect of supplementation with different fatty acid profile to the dam in early gestation and to the offspring on the finishing diet on offspring growth and hypothalamus mRNA expression in sheep. J Anim Sci. 99(4): 1–12.
- Petit HV. 2010. Feed intake, milk production and milk composition of dairy cows fed flaxseed. Can J Anim Sci. 90(2):115–127.
- R Core Team. R: A language and environment for statistical computing. Vienna, Austria: R Foundation for Statistical Computing.
- Radunz AE, Wickersham LA, Loerch SC, Fluharty FL, Reynolds CK, Zerby HN. 2009. Effects of dietary polyunsaturated fatty acid supplementation on fatty acid composition in muscle and subcutaneous adipose tissue of lambs. J Anim Sci. 87(12):4082–4091.
- Rajion MA, McLean JG, Cahill RN. 1985. Essential fatty acids in the fetal and newborn lamb. Aust J Biol Sci. 38(1):33–40.
- Roque-Jimenez JA, Oviedo-Ojeda MF, Whalin M, Lee-Rangel HA, Relling AE. 2020. Eicosapentaenoic and docosahexaenoic acid supplementation during early gestation modified relative abundance on placenta and fetal liver tissue mRNA and concentration pattern of fatty acids in fetal liver and fetal central nervous system of sheep. PLOS one. 15(6):e0235217.
- Roque-Jiménez JA, Rosa-Velázquez M, Pinos-Rodríguez JM, Vicente-Martínez JG, Mendoza-Cervantes G, Flores-Primo A, Lee-Rangel HA, Relling AE. 2021. Role of long chain fatty acids in developmental programming in ruminants. Animals. 11(3):762.
- Rovadoscki GA, Pertile SFN, Alvarenga AB, Cesar ASM, Pértille F, Petrini J, Franzo V, Soares WVB, Morota G, Spangler ML, Pinto LFB, Carvalho GP, Lanna DPD, Coutinhoand LL, Mourão GB. 2018. Estimates of genomic heritability and genome-wide association study for fatty acids profile in Santa Inês sheep. BMC Genom. 19:1–14.
- Salehi R, Ambrose DJ. 2017. Prepartum maternal diets supplemented with oilseeds alter the fatty acid profile in bovine neonatal plasma possibly through reduced placental expression of fatty acid transporter protein 4 and fatty acid translocase. Reprod Fertil Dev. 29(9):1846–1855.
- Shand JH, Noble RC. 1979. Delta 9- and delta 6-desaturase activities of the ovine placenta and their role in the supply of fatty acids to the fetus. Biol Neonate. 36(5–6):298–304.
- Shand JH, Noble RC. 1981. The metabolism of 18:0 and 18:2(n-6) by the ovine placenta at 120 and 150 days of gestation. Lipids. 16(1):68–71.
- Shao T, Ireland FA, McCann JC, Shike DW. 2020. Effects of late gestation calcium salts of fatty acids supplementation to beef cows on offspring pre-weaning growth performance and gene expression. J Anim Sci. 98(Supplement_3):136–136.
- Starčević K, Filipović N, Šperanda M, Đidara M, and Mašek T. 2017. The influence of sex and gonadectomy on hepatic and brain fatty acid composition, lipogenesis and β-oxidation. J Anim Physiol Anim Nutr. 101(4):649–657.
- Tarry-Adkins JL, Ozanne SE. 2011. Mechanisms of early life programming: current knowledge and future directions. Am J Clin Nutr. 94(6 Suppl):1765S–1771S.
- Tedeschi LO, Cannas A, Fox DG. 2010. A nutrition mathematical model to account for dietary supply and requirements of energy and other nutrients for domesticated small ruminants: the development and evaluation of the small ruminant nutrition system. Small Rumin Res. 89(2–3):174–184.
- Tejeda JF, Peña RE, and Andrés AI. 2008. Effect of live weight and sex on physico-chemical and sensorial characteristics of Merino lamb meat. Meat Sci. 80(4):1061–1067.
- Ulbricht TLV, Southgate DAT. 1991. Coronary heart disease: seven dietary factors. Lancet. 338(8773):985–992.
- Valvo MA, Lanza M, Bella M, Fasone V, Scerra M, Biondi L, Priolo A. 2005. Effect of ewe feeding system (grass v. concentrate) on intramuscular fatty acids of lambs raised exclusively on maternal milk. Anim Sci. 81(3):431–436.
- Vieau D. 2011. Perinatal nutritional programming of health and metabolic adult disease. World J Diabetes. 2(9):133–136.
- Vlaeminck B, Fievez V, Tamminga S, Dewhurst RJ, Van Vuuren A, De Brabander D, Demeyer D. 2006. Milk odd-and branched-chain fatty acids in relation to the rumen fermentation pattern. J Dairy Sci. 89(10):3954–3964.
- Wachira AM, Sinclair LA, Wilkinson RG, Enser M, Wood JD, Fisher AV. 2002. Effects of dietary fat source and breed on the carcass composition, n-3 polyunsaturated fatty acid and conjugated linoleic acid content of sheep meat and adipose tissue. Br J Nutr. 88(6):697–709.
- Ye Y, Eyres GT, Reis MG, Schreurs NM, Silcock P, Agnew MP, Johnson PL, Maclean P, Realini CE. 2020. Fatty acid composition and volatile profile of M. longissimus thoracis from commercial lambs reared in different forage systems. Foods. 9(12):1885.
- Zhou Y, Nijland M, Miller M, Ford S, Nathanielsz PW, Brenna JT. 2008. The influence of maternal early to mid-gestation nutrient restriction on long chain polyunsaturated fatty acids in fetal sheep. Lipids. 43(6):525–531.
- Zhu MJ, Ford SP, Means WJ, Hess BW, Nathanielsz PW, Du M. 2006. Maternal nutrient restriction affects properties of skeletal muscle in offspring. J Physiol. 575(1):241–250.
- Zhu MJ, Ford SP, Nathanielsz PW, Du M. 2004. Effect of maternal nutrient restriction in sheep on the development of fetal skeletal muscle. Biol Reprod. 71(6):1968–1973.