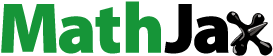
Abstract
Methane plays a major role in greenhouse gas emissions contributing to climate change. Therefore, reducing methane production by ruminants is a primary goal but significant challenge. In this study, five oriental medicine plant extracts (OMPEs), Hypericum ascyron L. (HYA), Rhododendron mucronulatum (RHM), Zizyphus jujube var. inermis (ZIJ), Vitis vinifera L. (VIV), and Rhus succedanea L. (RHS), with different polyphenol and flavonoid contents were evaluated for their effects on in vitro ruminal fermentation parameters, gas profiles, and changes in the microbial population of targeted groups including cellulolytic bacteria and methanogenic archaea (M. archaea). The total polyphenol and flavonoid content of the OMPEs was in the range of 30.53–266.62 mg GAE·g-1 and 4.95–125.43 mg CHE·g-1, respectively. Each OMPE was tested at a fixed dose (50 mg·L-1) after 48 h incubation in a batch culture system. Total gas production in the RHM treatment was significantly higher than that in the control group after 24 h of incubation, whereas methane production decreased by 34.08–41.01% in the RHM, ZIJ, VIV, and RHS treatment groups. Quantification of microbial populations showed that the relative abundance of two Ruminococcus species was decreased by treatment of all OMPEs whereas the relative abundance of Fibrobacter succinogenes increased. The abundance of M. archaea was reduced by VIV and RHS treatment groups. In conclusion, this study suggests that polyphenols and flavonoids extracted from OMPEs have potential to reduce methane production in ruminants, which should be further validated testing in vivo feeding trials for implementation.
Some oriental medicine plants rich in polyphenol and/or flavonoid contents can be used to mitigate ruminant methane production.
Supplementation of Vitis vinifera L. and Rhus succedanea L. extracts could reduce methane production and the abundance of methanogenic archaea.
Highlight
Introduction
Greenhouse gases such as methane and carbon dioxide produced by ruminants are correlated with climate change. Methane has 28 times more global warming potential than carbon dioxide (Stocker Citation2014). In addition, 2–12% of the total feed energy intake is reportedly lost through methane production (Johnson and Johnson Citation1995). Thus, reducing methane production from ruminants can have marked economic and environmental benefits, which is a critical challenge to ruminant nutritionists. The most feasible solution to manipulate the rumen microbial ecosystem is to increase fibre-rich feed digestibility and reduce methane and nitrogen excretion.
Several studies on feed additive technology have focussed on the potential applications of natural products. Plants produce various secondary metabolites to protect against insect attacks and microbes (Wallace Citation2004), including essential oils, tannins, saponins, flavonoids, and other polyphenols, which represent promising anti-methanogenic compounds (Hayat et al. Citation2020; Ku-Vera et al. Citation2020). Notably, adding medicinal plant extracts has beneficial effects on reduce methane production by altering the ruminal microbial population and structure (Petrič et al. Citation2020). However, several studies have shown that although plant extract additives in the diet reduce methane production, they have detrimental effects on the rumen microbial population, digestibility, and fermentation parameters at high levels (Bharanidharan et al. Citation2021; Huang et al. Citation2021). Therefore, discovering plant extracts that effectively reduce methane production with the added effects of improved digestibility and ruminant performance is one of the most important goals in the field of ruminant nutritionists.
The high flavonoid content in plant extracts has several positive effects, such as reducing methane production, stimulating microbial metabolism, increasing the digestibility of crude protein (CP) and neutral detergent fibres (NDF), and improving the yield and efficiency of microbial biomass products (Broudiscou et al. Citation2000; Citation2002). Flavonoids such as quercetin in the extracts of the oriental medicinal plants (OMPEs); have been identified in Hypericum ascyron L. (HYA), Rhododendron mucronulatum (RHM), Zizyphus jujube var. inermis (ZJI), and Vitis vinifera L. (VIV). Additionally, HYA comprises kaempferol and isoquercitrin; RHM comprises myricetin and myricitrin; ZJI comprises rutin, isoquercitrin, nicotiflorin, and phloretin; and VIV comprises myricetin, kaempferol, and isorhamnetin (Kim et al. Citation1996; Kwon et al. Citation2000; Castillo-Muñoz et al. Citation2007; Lee et al. Citation2016). In contrast, the bioflavonoids amentoflavone, agathisflavone, robustaflavone, hinokiflavone, volkensiflavone, rhusflavanone, and succedaneaflavanone have all been isolated from Rhus succedanea L. (RHS) (Lin et al. Citation1997).
These OMPEs exhibit various properties, such as antioxidant, antimicrobial, and antiviral functions, which can aid ruminal fermentation. Using an in vitro gas production technique, Chinese medicinal plants were demonstrated to reduce methane production by approximately 23–24% (Wang et al. Citation2019). Furthermore, in our previous study evaluating the effect of different concentrations of RHS extract on in vitro ruminal gas production, a dose of 50 mg·L-1 was found to reduce methane production by up to 31% (Kim et al. Citation2018). Therefore, the objective of the present study was to verify our previous results by evaluating the effects of the other above-mentioned OMPEs on rumen methane production (in terms of in vitro ruminal fermentation, gas profile, and microbial population), using the RHS extract as a positive control. We hypothesised that OMPEs rich in polyphenol and flavonoid contents would have a greater effect in reducing methane production and consequently alter the microbial population.
Material and methods
Ethical statement
All experimental protocols used in this study were reviewed and approved by the Animal Ethics Committee of Gyeongsang National University (GNU-180130-A0007).
Preparation and antioxidants activity evaluation of OMPEs
Five OMPEs were purchased from the Korea Plant Extract Bank (KRIBB, Daejeon, Korea). General information, chemical constituents, and pharmacological activities of the extracts were shown in Table . The plants were obtained cut or crushed and then dried naturally in the shade, and extracted with 99.9% methanol. The OMPEs were dissolved in dimethyl sulfoxide and used.
Table 1. General information, chemical constituents, and pharmacological activities on oriental medicine plant extracts used in the in vitro experiment.
Total polyphenols content was determined according to the Folin-Ciocalteu method with a slight modification reported by Velioglu et al. (Citation1998), using gallic acid as a standard. Briefly, 10 µL of each OMPEs were added 180 µL of 2% Na2CO3 and 10 µL of 50% Folin-Ciocalteu reagent, the mixtures were incubated for 30 min. The each reaction mixtures were calculated from the optical density (OD) at 765 nm using Multimicroplate reader-SpectraMax M5 (Molecular Devices, Sunnyvale, CA, USA). Total polyphenol content was expressed as mg of gallic acid equivalents (GAE)·g-1 of OMPEs.
Total flavonoids content was determined according to the aluminium chloride method with a slight modification reported by Zhishen et al. (Citation1999), using catechin hydrate as a standard. Briefly, 25 µL of each OMPEs were diluted with methanol of 75 µL, then 7.5 µL of 5% NaNO2 and 15 µL of 10% AICI3·6H2O were added sequentially, the mixtures were incubated for 15 min. Each reaction mixtures were calculated from the OD at 430 nm using Multimicroplate reader-SpectraMax M5 (Molecular Devices, Sunnyvale, CA, USA). Total flavonoid content was expressed as mg of catechin hydrate equivalents (CHE)·g-1 of OMPEs.
Animals and in vitro experimental design
The rumen fluid used for the in vitro experiments was collected from two rumen fistulated Hanwoo cows (450 ± 30 kg) fed a 6:4 mixed diet [3% dry matter (DM) of body weight] of Timothy hay and corn-based concentrate twice a day (07:00 and 17:00) and had free access to mineral block and water. The rumen fluid collected was transported to the laboratory within 10 min, and filtered from 4 layers of sterilised cheesecloth. Before incubation rumen fluid and McDougall’s buffer were mixed at a ratio of 1:2 (v/v), and the mixture was flushed with carbon dioxide at 39 °C. Substrate was ground through a 1 mm screen with a Wiley mill (Arthur H. Thomas, Philadelphia, PA), and 300 mg Timothy hay (moisture content, 5.89%; CP, 10.49%; ether extracts, 5.48%; crude fibre, 30.11%; crude ash, 5.93%; NDF, 62.27%, and acid detergent fibre, 38.14%) were placed into nylon bags (pore size 50 ± 10 µm, R510, Ankom Technology, NY, USA). The fluid mixture (20 mL·bottle-1) was anaerobically dispensed into 50 mL serum bottle containing nylon bag and one of five OMPEs (50 mg·L-1), under a stream of O2-free N2. After sealing with butyl rubbers plus crimped aluminium seals, the serum bottles were incubated at 39 °C for 3, 6, 9, 12, 24, and 48 h at a 120 rpm shaking incubator (SI-900R: Jeio Tech, Daejeon, Korea). The in vitro fermentation experiments were replicated in three separate communities. Each in vitro fermentation experiment was a completely randomised block design and performed in three runs, using 108 serum bottles (6 incubation times × 6 treatments × 3 repetitions).
In vitro fermentation and gas profiles
At each incubation time, total gas production was calculated through detachable pressure transducer and a digital readout voltmeter (Laurel Electronics, inc., Costa Mesa, Ca, USA) at headspace gas pressure in incubation time according to Theodorou et al. (Citation1994), as follows:
Headspace samples of serum bottles were collected to determine for methane and carbon dioxide in the vacuum tube and then that were analysed by gas chromatography (Agilent Technologies HP 5890, Germany) with thermal conductivity detector on Carboxen 1006 PLOT capillary column (30 × 0.53 mm; Supelco, Bellefonte, PA, USA). The methane and carbon dioxide production was converted according to López et al. (Citation2007), as follows:
After opening serum bottle, pH of the rumen fluid was determined using a pH metre (S210 SevenCompact, Mettler-Toledo, Greifensee, Switzerland) and sampled for volatile fatty acid (VFA), microbial growth rate, ammonia nitrogen concentration analysis. Rumen fluid was stored at −80 °C in a freezer until use for analysis. The VFA production was analysed using high-performance liquid chromatography (HPLC; Agilent Technologies 1200 series, USA) according to Tabaru et al. (Citation1988). Microbial growth rate, and ammonia nitrogen concentration were measured using a spectrophotometer (Model 680, Bio-Rad Laboratories, Hercules, CA, USA) to OD at 550 nm and 630 nm respectively according to the method described previously (Lee et al. Citation2019). After incubation, the apparentDM digestibility rate was determined according to the nylon bag digestion method. The nylon bag with substrate was washed in a water bath using Heidolphs Rotamax 120 (Heidolph Instrument, Nuremberg, Germany) for 20 min at 100 rpm, three repetitions, then that was oven-dried at 65 °C for 72 h. Apparent DM digestibility rate was calculated as difference in the weight of the nylon bag before and after incubation.
Relative quantification of specific ruminal microbes
At the end of incubation, 1 mL of the rumen fluid mixture was used to extract total DNA using the QIAamp mini kit (QIAGEN, Valencia, CA, USA) using the modified bead-beating protocol. The concentration and purity of the extracted DNA were measured using a NanoDrop (Thermo Scientific, Wilmington, DE, USA). Real time-polymerase chain reaction (RT-PCR) assays were conducted using a RT-PCR Machine (CFX96 Real-Time system, Bio-Rad, USA). The reaction was done using the SYBR Green Supermix (QPK-201, Toyobo Co., Ltd., Tokyo, Japan) in each final volume of 20 μL containing forward and reverse primers (10 pmol each), the SYBR Green Supermix, and template DNA. The primers used for general bacteria (internal standard of each bacteria) have been described by Denman & McSweeney (Citation2006) and those for Methanogenic archaea (M. archaea) and cellulolytic bacteria (Ruminococcus albus; R. albus, Fibrobacter succinogenes; F. succinogenes, and Ruminococcus flavefaciens; R. flavefaciens) have been described by Tajima et al. (Citation2001) and Koike & Kobayashi (Citation2001), respectively. The negative control was analysed in RT-PCR analysis with the mixture of each primer without template DNA. RT-PCR condition was as follows; one cycle of initiation at 95 °C for 3 min followed by 40 cycles of denaturation at 95 °C for 30 s, annealing at 60 °C for 30 s, and elongation at 72 °C for 30 s. Relative quantification of each microbe was obtained by the difference in between cycle threshold (Ct) of control and Ct of additive follow as = 2−ΔCt(Additive) −ΔCt(Control).
Statistical analysis
All data were statistically analysed with a completely randomised block design procedure of Statistical Analysis used SAS software, version 9.4. The statistical model used was as follows: Yijkl = μ + αi + βj + γk + εijkl, where Yijkl = experimental data, µ = overall mean, αi = random effect of the fermentation trials, βj = fixed effect of dietary treatments, γk = fixed effect of incubation times, and εijkl = unexplained random error. Significant differences between individual means were identified using Tukey’s multiple comparison tests. Significance was defined at a p-value < .05.
Results
Total polyphenols and flavonoids in the five OMPEs
The total polyphenol and flavonoid contents in the five OMPEs are shown in Table , which were in the range of 30.53–266.62 mg GAE·g-1 and 4.95–125.43 mg CHE·g-1, respectively. In particular, the total polyphenol concentration was the highest in the RHS extract, followed by the HYA and RHM extracts. The total flavonoid concentration was the highest in the HYA extract, followed by the RHM extract.
Table 2. Total polyphenols and flavonoids in oriental medicine plant extracts.
In vitro fermentation characteristics
The in vitro pH, microbial growth rate and ammonia nitrogen concentrations at 12 h and 24 h incubation are shown in Table . The pH of the VIV and RHS treatment groups at 12 h was significantly higher (p < .05) than that of the control (CON); the pH of the VIV treatment at 24 h incubation was also significantly higher (p < .05) than that of the CON. The microbial growth rate in the VIV treatment after 24 h of incubation was significantly lower (p < .05) than that in the CON. The ammonia nitrogen concentrations in all OMPE treatment groups at 12 h and 24 h incubation were not significantly different (p > .05) from that in the CON.
Table 3. Effect of oriental medicine plant extracts on ruminal fermentation characteristics after 12 and 24 h in vitro incubation.
In vitro apparent DM digestibility, and VFA profiles
As shown in Figure , the apparent DM digestibility was affected by the addition of OMPEs at different incubation times. After 3 h of incubation, the apparent DM digestibility was significantly different (p < .05) among the treatment groups. Nonetheless, the overall apparent DM digestibility at other incubation times did not differ significantly (p > .05) among any of the OMPE treatment groups compared with that of the CON.
Figure 1. Effects of oriental medicine plant extracts on apparent dry matter digestibility in in vitro incubation. Error bars are standard error of the mean (n = 3). a,bMeans with different superscripts in the same row indicate significant differences (p < 0.05).
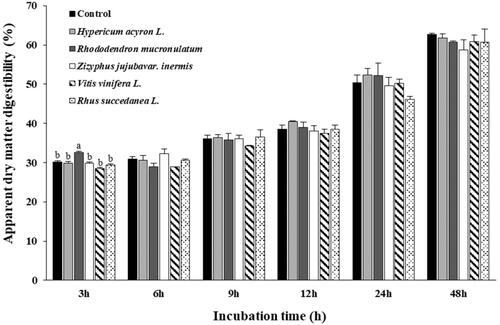
The in vitro VFA profiles and acetic acid to propionic acid ratio at 12 h and 24 h incubation are shown in Table . The total VFA, acetic acid, and propionic acid concentrations after 12 h and 24 h of incubation did not differ significantly (p > .05) between any of the OMPE treatment groups and those of the CON, whereas the acetic acid to propionic acid ratio of the ZJI extract after 12 h of incubation was significantly higher (p < .05) than that of the CON.
Table 4. Effect of oriental medicine plant extracts on volatile fatty acid profiles after 12 and 24 h in vitro incubation.
In vitro gas profiles
The in vitro gas profiles at 12 h and 24 h of incubation are shown in Table . Total gas production at 12 h was slightly affected by the addition of all OMPE treatment groups, but the effect was not statistically significant (p > .05). The total gas production in the RHM treatment at 24 h incubation was significantly higher (p < .05) than that in the CON group. Methane production and proportion of total gas in all OMPE treatment groups at 12 h incubation were not significantly altered (p > .05), whereas those in the RHM, ZJI, VIV, and RHS treatment groups at 24 h incubation were significantly lower (p < .05) than those in the CON, with the lowest values observed in the RHS treatment. Carbon dioxide production and the proportion of total gas did not change significantly (p > .05) in any of the OMPE treatment groups at 12 h and 24 h incubation, although they were reduced in the RHM, ZJI, VIV, and RHS treatment groups at 24 h incubation compared with those in the CON group.
Table 5. Effects of oriental medicine plant extracts on gas profiles after 12 and 24 h in vitro incubation.
Relative quantification of specific ruminal microbes
The effects of the five OMPEs on the population of M. archaea and the major fibrolytic microorganisms R. albus, F. succinogenes, and R. flavefaciens are shown in Figure . The relative abundance of F. succinogenes in the HYA, RHM, and RHS treatment groups at 12 h incubation was significantly higher (p < .05) than that in the CON, and was significantly higher (p < .05) in all OMPE treatment groups at 24 h incubation than that in the CON. The abundance of R. albus and R. flavefaciens in all OMPE treatment groups after 24 h incubation was significantly lower (p < .05) than that in the CON group. The abundance of M. archaea in the VIV and RHS treatment groups at 24 h incubation was significantly lower (p < .05) than that in the CON.
Figure 2. Relative quantification of in vitro rumen microbial population at 12 h (A), 24 h (B) incubation by different oriental medicine plant extracts. Error bars are standard error of the mean (n = 3) R. albus, Ruminococcus albus; F. succinogenes, Fibrobacter succinogenes; R. flavefaciens, Ruminococcus flavefaciens; M. archaea, Methanogenic archaea. a,b,cMeans with different superscripts in the same row indicate significant differences (p < .05).
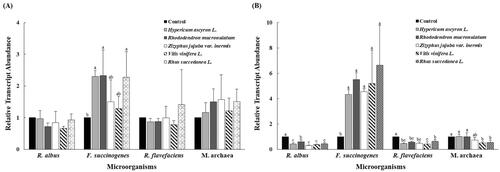
Discussion
Ruminal microorganisms are essential to the host animal for the proper fermentation and digestion of feed. Fibrolytic bacteria are significantly affected by pH; therefore, the ruminal pH is a crucial factor for maintaining microbial function in the rumen (Hiltner and Dehority Citation1983). Furthermore, pH changes can occur via the accumulation of VFA, which should therefore be correlated with the digestion and fermentation of the diet; however, no such effects were noted in this study. Polyphenols and flavonoids can affect the pH value by changing the microbial quantity and quality. Thus, the higher pH of the VIV and RHS treatment groups can be partly explained by activity of bioactive substances, which can slow down the rate of fibre degradation in the ruminal fluid (Cone Citation1991). After the addition of the five OMPEs tested in the study, the pH values of the rumen fluid ranged from 6.44 to 7.04. Reportedly, ruminal microbial activity is negatively affected when the pH is lower than 5.0 − 5.5 (Hoover Citation1986). Our study results suggest that the pH of the tested OMPEs did not have a detrimental effect on fibre degradation. Hence, their apparent DM digestibility was not negatively affected over time. However, there was a significant difference in the RHM treatment at 3 h incubation, which likely occurred because rumen microorganisms in the CON and the all OMPE treatment groups became acclimated to changing environmental conditions during the first 3 h of incubation and may have exponentially increased in number when they adapted at approximately 6 h incubation. Indeed, in a previous study incorporating additives similar to the extracts used in the present study, rumen microorganisms were found to acclimate to changing environmental conditions during the early phase and then increased exponentially after adaptation (Lee et al. Citation2018). Furthermore, these results are supported by the lack of an effect on the microbial growth rate and ammonia nitrogen concentration in the middle stage of fermentation (i.e., at 12 h incubation).
The main end products of ruminal fermentation of organic matter are VFAs and gases. In general, the production of VFAs and total gas was not affected by any of the OMPEs tested in this study. According to Van Soest (Citation1994), VFAs produced in the rumen by microbial fermentation are the main source of energy supply for ruminants. However, high doses of different plant extracts have been shown to reduce the total VFA concentration of mixed ruminal microorganisms, activity of rumen microbes, and total gas production (Busquet et al. Citation2006). The lack of effect of OMPEs on total VFA concentration and gas production in this study suggests that the dose used did not negatively affect ruminant nutrition. The five OMPEs contain plant secondary metabolites, such as flavonoids (kaempferol and isoquercitrin) and/or bioflavonoids, which have strong antibacterial activities (Kim et al. Citation1996; Lin et al. Citation1997; Kwon et al. Citation2000; Castillo-Muñoz et al. Citation2007; Lee et al. Citation2016). Generally, their antimicrobial activity has been found to reduce methane production by altering rumen microorganisms in in vitro cultures. This study identified provides the first demonstration for the ability of RHM, ZJI, VIV, and RHS treatment groups to substantially inhibit methane production without decreasing the total VFA concentration even after 24 h incubation. Additionally, these extracts had a similar effect on the proportion of methane among the total gases, although the RHS extract had the least effect. RHM shells have very high levels of hydrolysed tannins, and Bhatta et al. (Citation2009) confirmed that tannins reduce methanogenesis directly through their anti-methanogenic activity and indirectly through their anti-protozoal activity. Additionally, approximately 39% reduction in methane production was observed at 24 h incubation with addition of the RHS extract, which is higher than the 31% reduction reported by Kim et al. (Citation2018), who identified RHS extract dose-response effects in in vitro batch culture experiment. These differences of results can be explained by differences in microbial populations and activities in rumen fluid over the incubation period. Approximately 80% of the ruminant intestinal methanogens are used as major substrates for hydrogen and carbon dioxide or formate (Whitman et al. Citation1992); although carbon dioxide production in the all OMPE treatment groups did not change significantly.
F. succinogenes, R. albus, and R. flavefaciens are recognised as the main cellulolytic bacterial species in the rumen, and their relative proportions can potentially affect the ratios and concentrations of VFAs available to ruminants (Dehority Citation1993; Forsberg et al. Citation1997). Cushnie and Lamb (Citation2005) reported that flavonoids have antibacterial effects against gram-positive bacteria. Indeed, the abundance of gram-positive bacteria (the two Ruminococcus species) was significantly lower in all OMPE treatment groups after 24 h of incubation than that in the CON. However, the abundance of F. succinogenes was significantly higher in all OMPE treatment groups after 24 h of incubation than that in the CON. Kim et al. (Citation2015) reported that flavonoid-rich plant extract additives increased the abundance of F. succinogenes, but decreased the abundance of R. albus and R. flavefaciens. This suggests that the OMPEs probably likely did not negatively affect cellulose digestion, since Fibrobacter species digest crystalline cellulose more actively than ruminococcal species (Cheng et al. Citation1984; Miron and Ben-Ghedalia Citation1992). Latham and Wolin (Citation1977) and Ntaikou et al. (Citation2008) also reported that R. albus and R. flavefaciens are hydrogen-producing bacteria, whereas F. succinogenes does not produce hydrogen. Therefore, all of the tested OMPEs could reduce methane, as variations in the microbial populations may have reduced the production of precursors to methane, such as hydrogen.
Methane-producing archaea are known as methanogens, which are a distinct group of organisms that are a normal component of the rumen microbial ecosystem (Tavendale et al. Citation2005). Ku-Vera et al. (Citation2020) reported that some plant extracts contain secondary metabolites such as tannins, saponins, and flavonoids, which act as direct suppressors of M. archaea in rumen. These results are consistent with the results of this study showing that the abundance of M. archaea was significantly lower in all OMPE treatment groups after 24 h of incubation than that in the CON. This difference could be explained by the inhibitory effects of phenolic acids and polyphenols on the population of methanogens, their activity, or both (Bodas et al. Citation2012). Additionally, it is important to consider that place of plantation affects polyphenols compounds in plants, resulting in a variety of flavonoids and phenolic acid contents, which subsequently exert ruminal fermentation and methanogenesis differently (Puchalska et al., Citation2021).
Conclusions
The objective of this study was to screen five OMPEs (50 mg·L-1) with antioxidant activity to determine their potential in reducing ruminal methane production. The type of polyphenols in the plant extracts influenced the degree of methane production. The RHM, ZJI, VIV, and RHS treatment groups reduced methane by approximately 30–40%, without negative effects on apparent DM digestibility, VFA production, and ruminal fermentation. This result is supported that the reduction of R. albus and R. flavefaciens by the antibacterial activities of flavonoids in some OMPEs. Moreover, reduction in methane production with the addition of the VIV and RHS treatment groups was largely due to a decrease in the relative abundance of M. archaea in the microbial population. Therefore, the present study verifies our previous results of the methane-reducing effect of the RHS extract extends these properties to several other OMPEs. Consequently, the polyphenols and/or flavonoids of OMPEs can be used as effective methane reduction additives. Future research with animal hosts are required to evaluate the sustainability of RHM, ZJI, VIV, and RHS supplementation in mitigating methane production and rumen fermentation without detrimental effects on the ruminants.
Disclosure statement
No potential conflict of interest was reported by the authors.
Data availability statement
The data presented in this study are available on request from the corresponding author.
Additional information
Funding
References
- Akaberi M, Hosseinzadeh H. 2016. Grapes (Vitis vinifera) as a potential candidate for the therapy of the metabolic syndrome. Phytother Res. 30(4):540–556.
- Bharanidharan R, Arokiyaraj S, Baik M, Ibidhi R, Lee SJ, Lee Y, Nam IS, Kim KH. 2021. In vitro screening of east asian plant extracts for potential use in reducing ruminal methane production. Animals. 11(4):1020.
- Bhatta R, Uyeno Y, Tajima K, Takenaka A, Yabumoto Y, Nonaka I, Enishi O, Kurihara M. 2009. Difference in the nature of tannins on in vitro ruminal methane and volatile fatty acid production and on methanogenic archaea and protozoal populations. J Dairy Sci. 92(11):5512–5522.
- Bodas R, Prieto N, García-González R, Andrés S, Giráldez FJ, López S. 2012. Manipulation of rumen fermentation and methane production with plant secondary metabolites. Anim Feed Sci Technol. 176(1-4):78–93.
- Broudiscou LP, Papon Y, Broudiscou AF. 2000. Effects of dry plant extracts on fermentation and methanogenesis in continuous culture of rumen microbes. Anim Feed Sci Technol. 87(3-4):263–277.
- Broudiscou LP, Papon Y, Broudiscou AF. 2002. Effects of dry plant extracts on feed degradation and the production of rumen microbial biomass in a dual outflow fermenter. Anim Feed Sci Technol. 101(1-4):183–189.
- Busquet M, Calsamiglia S, Ferret A, Kamel C. 2006. Plant extracts affect in vitro rumen microbial fermentation. J Dairy Sci. 89(2):761–771.
- Castillo-Muñoz N, Gómez-Alonso S, García-Romero E, Hermosín-Gutiérrez I. 2007. Flavonol profiles of Vitis vinifera red grapes and their single-cultivar wines. J Agric Food Chem. 55(3):992–1002.
- Cheng KJ, Stewart CS, Dinsdale D, Costerton JW. 1984. Electron microscopy of bacteria involved in the digestion of plant cell walls. Anim Feed Sci Technol. 10(2-3):93–120.
- Cone JW. 1991. Degradation of starch in feed concentrates by enzymes, rumen fluid and rumen enzymes. J Sci Food Agric. 54(1):23–34.
- Cushnie TPT, Lamb AJ. 2005. Antimicrobial activity of flavonoids. Int J Antimicrob Agents. 26(5):343–356.
- Dehority BA. 1993. Microbial ecology of cell wall fermentation. In: Jung HG, Buxton DR, Hatfield RD, Ralph J editors. Forage cell wall structure and digestibility. Madison, WI, USA: American Society of Agronomy/Crop Science Society of America/Soil Science Society of America. p. 425–453.
- Denman SE, McSweeney CS. 2006. Development of a real-time PCR assay for monitoring anaerobic fungal and cellulolytic bacterial populations within the rumen. FEMS Microbiol Ecol. 58(3):572–582.
- Forsberg CW, Cheng K-J, White BA. 1997. Polysaccharide degradation in the rumen and large intestine. In: Mackie RI, White BA editors. Gastrointestinal Microbiology. New York, NY, USA: Chapman and Hall. p. 319–379.
- Hayat J, Akodad M, Moumen A, Baghour M, Skalli A, Ezrari S, Belmalha S. 2020. Phytochemical screening, polyphenols, flavonoids and tannin content, antioxidant activities and FTIR characterization of Marrubium vulgare L. from 2 different localities of Northeast of Morocco. Heliyon. 6(11):e05609.
- Hiltner P, Dehority BA. 1983. Effect of soluble carbohydrates on digestion of cellulose by pure cultures of rumen bacteria. Appl Environ Microbiol. 46(3):642–648.
- Hoover WH. 1986. Chemical factors involved in ruminal fiber digestion. J Dairy Sci. 69(10):2755–2766.
- Huang H, Szumacher-Strabel M, Patra AK, Ślusarczyk S, Lechniak D, Vazirigohar M, Varadyova Z, Kozłowska M, Cieślak A. 2021. Chemical and phytochemical composition, in vitro ruminal fermentation, methane production, and nutrient degradability of fresh and ensiled Paulownia hybrid leaves. Anim. Feed Sci. Technol. 279(115038):115038.
- Johnson KA, Johnson DE. 1995. Methane emissions from cattle. J Anim Sci. 73(8):2483–2492.
- Kambhoja S, Nagraj MS. 2011. In-vitro antiprotozoal activity of Zizyphus jujube Mill and Lamk. Res J Pharmacol Pharmacodynam. 3(1):34–36.
- Kim DH, Lee SL, Oh DS, Lee ID, Eom JS, Park HY, Choi SH, Lee SS. 2018. In vitro evaluation of Rhus succedanea extracts for ruminants. Asian-Australas J Anim Sci. 31(10):1635–1642.
- Kim ET, Guan LL, Lee SJ, Lee SM, Lee SS, Lee ID, Lee SK, Lee SS. 2015. Effects of flavonoid-rich plant extracts on in vitro ruminal methanogenesis, microbial populations and fermentation characteristics. Asian Australas J Anim Sci. 28(4):530–537.
- Kim M, Jones AD, Chung TY. 1996. Antioxidative activity of flavonoids isolated from Jindalrae flowers (Rhododendron mucronulatum Turcz.). Appl Biol Chem. 39(4):320–326.
- Koike S, Kobayashi Y. 2001. Development and use of competitive PCR assays for the rumen cellulolytic bacteria: Fibrobacter succinogenes, Ruminococcus albus and Ruminococcus flavefaciens. FEMS Microbiol Lett. 204(2):361–366.
- Ku-Vera JC, Jiménez-Ocampo R, Valencia-Salazar SS, Montoya-Flores MD, Molina-Botero IC, Arango J, Gómez-Bravo CA, Aguilar-Pérez CF, Solorio-Sánchez FJ. 2020. Role of secondary plant metabolites on enteric methane mitigation in ruminants. Front Vet Sci. 7:584.
- Kwon SH, Yun SY, Lee KT, Park HJ. 2000. Isolation of steroids and flavonoids from the herbs of Hypericum ascyron L. Korean J Pharmacogn. 31(1):39–44.
- Latham MJ, Wolin M. 1977. Fermentation of cellulose by Ruminococcus flavefaciens in the presence and absence of Methanobacterium ruminantium. Appl Environ Microbiol. 34(3):297–301.
- Lee KM, Kwon KR, Choi SM, Kim TH. 2002. Aliterature study on lacquer poison. J Pharmacopuncture. 5(1):159–169.
- Lee MK, Kim HW, Kim YJ, Lee SH, Jang HH, Jung HA, Kim SB, Lee SH, Choe JS, Kim JB. 2016. Profiling of flavonoid glycosides in fruits and leaves of jujube (Zizyphus jujuba var. inermis (Bunge) Rehder) using UPLC-DAD-QTOF/MS. Korean J Food Preserv. 23(7):1004–1011.
- Lee SJ, Jeong JS, Shin NH, Lee SK, Kim HS, Eom JS, Lee SS. 2019. Impact of Ecklonia stolonifera extract on in vitro ruminal fermentation characteristics, methanogenesis, and microbial populations. Asian-Australas J Anim Sci. 32(12):1864–1872.
- Lee SJ, Shin NH, Jeong JS, Kim ET, Lee SK, Lee SS. 2018. Effect of Rhodophyta extracts on in vitro ruminal fermentation characteristics, methanogenesis and microbial populations. Asian-Australas J Anim Sci. 31(1):54–62.
- Li XM, Luo XG, Si CL, Wang N, Zhou H, He JF, Zhang TC. 2015. Antibacterial active compounds from Hypericum ascyron L. induce bacterial cell death through apoptosis pathway. Eur J Med Chem. 96:436–444.
- Lin YM, Anderson H, Flavin MT, Pai YHS, Mata-Greenwood E, Pengsuparp T, Pezzuto JM, Schinazi RF, Hughes SH, Chen FC. 1997. In vitro anti-HIV activity of biflavonoids isolated from Rhus succedanea and Garcinia multiflora. J Nat Prod. 60(9):884–888.
- López S, Dhanoa MS, Dijkstra J. a, Bannink A, Kebreab E, France J. 2007. Some methodological and analytical considerations regarding application of the gas production technique. Anim Feed Sci Technol. 135(1-2):139–156.
- Miron J, Ben-Ghedalia D. 1992. The degradation and utilization of wheat-straw cell-wall monosaccharide components by defined ruminal cellulolytic bacteria. Appl Microbiol Biotechnol. 38(3):432–437.
- Ntaikou I, Gavala HN, Kornaros M, Lyberatos G. 2008. Hydrogen production from sugars and sweet sorghum biomass using Ruminococcus albus. Int J Hydrogen Energy. 33(4):1153–1163.
- Petrič D, Mravčáková D, Kucková K, Čobanová K, Kišidayová S, Cieslak A, Ślusarczyk S, Váradyová Z. 2020. Effect of dry medicinal plants (wormwood, chamomile, fumitory and mallow) on in vitro ruminal antioxidant capacity and fermentation patterns of sheep. J Anim Physiol Anim Nutr. 104(5):1219–1232.
- Popescu R, Kopp B. 2013. The genus Rhododendron: an ethnopharmacological and toxicological review. J Ethnopharmacol. 147(1):42–62.
- Puchalska J, Szumacher-Strabel M, Patra AK, Ślusarczyk S, Gao M, Petrič D, Nabzdyk M, Cieślak A. 2021. The effect of different concentrations of total polyphenols from Paulownia hybrid leaves on ruminal fermentation, methane production and microorganisms. Animals. 11(10):2843.
- Stocker T. 2014. Climate change 2013: the physical science basis: Working Group I contribution to the Fifth assessment report of the Intergovernmental Panel on Climate Change. New York, USA: Cambridge University Press.
- Tabaru H, Kadota E, Yamada H, Sasaki N, Takeuchi A. 1988. Determination of Volatile Fatty Acids and Lactic Acid in Bovine Plasma and Ruminal Fluid by High Performance Liquid Chromatography. Nihon Juigaku Zasshi. 50(5):1124–1126.
- Tajima K, Nagamine T, Matsui H, Nakamura M, Aminov RI. 2001. Phylogenetic analysis of archaeal 16S rRNA libraries from the rumen suggests the existence of a novel group of archaea not associated with known methanogens. FEMS Microbiol Lett. 200(1):67–72.
- Tavendale MH, Meagher LP, Pacheco D, Walker N, Attwood GT, Sivakumaran S. 2005. Methane production from in vitro rumen incubations with Lotus pedunculatus and Medicago sativa, and effects of extractable condensed tannin fractions on methanogenesis. Anim Feed Sci Technol. 123-124:403–419.
- Theodorou MK, Williams BA, Dhanoa MS, McAllan AB, France J. 1994. A simple gas production method using a pressure transducer to determine the fermentation kinetics of ruminant feeds. Anim Feed Sci Technol. 48(3-4):185–197.
- Van Soest PJ. 1994. Nutritional ecology of the ruminant. Ithaca, NY, USA: Cornstock Publishing Associates, Cornell University Press.
- Velioglu Y, Mazza G, Gao L, Oomah BD. 1998. Antioxidant activity and total phenolics in selected fruits, vegetables, and grain products. J Agric Food Chem. 46(10):4113–4117.
- Wallace RJ. 2004. Antimicrobial properties of plant secondary metabolites. Proc Nutr Soc. 63(4):621–629.
- Wang WJ, Wang SP, Luo DM, Zhao XL, Yin MJ, Zhou CF, Liu GW. 2019. Effect of Chinese herbal medicines on rumen fermentation, methanogenesis and microbial flora in vitro. SA J an Sci. 49(1):63–70.
- Whitman WB, Bowen TL, Boone DR. 1992. The methanogenic bacteria. In: A Ballows, HG Truper, M Dworkin, W Harder, and K-H Schleifer, editors. The prokaryotes: a handbook on the biology of bacteria: ecophysiology, isolation, identification, application. Vol I. New York: Springer-Verlag. p. 719–767.
- Zhishen J, Mengcheng T, Jianming W. 1999. The determination of flavonoid contents in mulberry and their scavenging effects on superoxide radicals. Food Chem. 64(4):555–559.