Abstract
This study was conducted to investigate the effects of dietary inclusion of alfalfa meal (AM) on the productive performance of Zhuanghe Dagu chickens. A total of 288 35-week-age layers were randomly assigned into four dietary treatments in a 35-day trial. Dietary conditions were based on a basal diet and supplemented with 0, 3, 6, 9% AM (CON, AM3, AM6 and AM9). Results showed that dietary inclusion of AM had positive effects on the egg production rate, feed conversion ratio (FCR), egg mass, yolk colour, albumen height and haugh unit. In addition, the small intestinal morphology such as villus height, crypt width and muscular thickness were improved by including AM in the diet. AM supplementation enriched the microbiota diversity in the caecum. The Firmicutes/Bacteroidetes ratio has decreased, and the Synergistetes has increased in AM supplemented groups compared with the CON group. Predictive functional analysis revealed that metabolic pathways of carbohydrate, lipid, terpenoids and polyketides, etc. were significantly enriched in the experimental groups. A total of 397 differential metabolites have been identified between AM6 group and CON group. Among them, a total of 176 metabolites were upregulated and 221 metabolites were downregulated. Therefore, dietary inclusion of AM was beneficial to improve the laying performance, egg quality, small intestinal morphology, caecal microbiota diversity and caecal metabolic function in Zhuanghe Dagu chickens, with the optimum dose being 6%.
Feeding Dagu chickens with AM containing diet had beneficial effects on the productive performance
Dietary inclusion of AM improved intestinal morphology parameters in Dagu chickens
The nutritional metabolic function of Dagu chickens was positively affected by including AM
Highlights
Introduction
Dagu chicken, an excellent Chinese indigenous chicken, is mainly reared in Zhuanghe City, Liaoning Province. They are well-known for their large body, large egg, delicious meat and rich nutrients. Generally, they are brooded indoors and raised in free-range systems (Su et al. Citation2014). The free-range systems did not play any limitation on chickens, contributing to decrease stress conditions and increase comfort for chickens (de Jong et al. Citation2005; Dishman et al. Citation2009; Fu et al. Citation2015). However, additional energy expenditure and the increase in the risk of bacterial, parasitic and viral disease incidences are problems that need to have attention in the scattered feeding systems (Al-Rawi et al. Citation1976; Fossum et al. Citation2009; Wang et al. Citation2009). In order to avoid the above risks, an intensive management system seems to be a good way in raising indigenous chickens. However, raising native chickens in cages may not be suitable for the natural behaviour of the birds. This will result in lower productivity. Therefore, exploring methods to improve the productivity of native chickens in intensive management systems is beneficial to native chickens farming. At present, research on the improvement in productivity of indigenous chickens under intensive management systems is still limited (Sebola et al. Citation2015).
In addition, since the cost and scarcity of conventional ingredients such as soybean and maize are increased, using cheap resources to formulate feed is essential for sustainable production in indigenous chickens (Ochetim Citation1993; Onuh et al. Citation2010). Alfalfa meal (AM) is a commercial feed ingredient that is rich in proteins, minerals, vitamins and large counts of functional active ingredients, such as luteins, β-carotenes, flavonoids, polysaccharides and saponins (Dong et al. Citation2007; Jiang et al. Citation2012; Laudadio et al. Citation2014). AM has been used in chicken breeding for a long time. Its high fibre and low energy contents are the main restrictive factors for its utilisation in poultry (Yıldız et al. Citation2020). However, an appropriate amount of insoluble fibre has been reported to modulate intestinal morphology, digestive organ development, nutrient absorption, growth performance and intestinal microbiota in chickens (Tejeda and Kim Citation2021). The effects of dietary supplementation of AM on the productive performance of poultry are variable. Some studies reported that dietary supplementation of AM had positive effects on the productive performance of chickens (Zheng et al. Citation2019). However, the negative effects also have been reported (Mourão et al. Citation2006; Englmaierova et al. Citation2019). The differences in the above studies might be related to the cellulose contents of alfalfa meal, environment and the breed, age, physiological status of the chickens are different, especially for chicken breeds. The indigenous chickens have been reported to be characterised with higher fibre digestibility than selected chicken breeds (Mabelebele et al. Citation2014).
However, studies on the effects of dietary supplementation of AM on the productive performance of cage-reared Dagu chickens were still limited. We hypothesised that feeding cage-reared Dagu chickens with AM containing diet had positive effects on the intestinal microbiota and intestinal morphology, thus improving laying performance and egg quality. The objective of this study was to evaluate the effects of AM supplementation on laying performance, egg quality, intestinal morphology, intestinal microbiota and functional prediction analysis of intestinal microbiota in Dagu chickens.
Material and methods
AM collection and preparation
AM was collected from alfalfa, planted in the experimental farm of Jinzhou Medical University, dried and crushed. Its components were measured by a commercial company (Qingdao Kechuang Quality Testing Co., Ltd., Qingdao, Shandong, China), which contains 87.90% dry matter, 22.48% crude protein, 19.57% crude fibre, 2.50% crude fat, 10.01% crude ash, 43.27% neutral detergent fibre, 25.01% acid detergent fibre, 1.20% lysine, 7.06 MJ/kg metabolisable energy, 0.36% flavonoids, 0.22% methionine and 0.016% β-carotene.
Birds, dietary treatments, experimental design and sample collection
The Ethical approval for the present study was obtained from the Ethical Committee of Jinzhou Medical University. A total of 288 35-week-age female Dagu chickens were randomly divided into four groups in a 35-day feeding trial. Dietary conditions were based on a basal diet and supplemented with 0, 3, 6, 9% AM (CON, AM3, AM6 and AM9). There were 6 replicates in each group and 12 hens per replicate. Laying hens were caged in two-tier battery cages under a 16 h light/8 h dark cycle. The size of the steel cage was 70.5 × 40 × 42 cm (940 cm2 bird−1). Water and feed were provided ad libitum during the experimental period. All diets were formulated to be isoenergetic and isoproteic. The ingredient composition and nutrient contents of the experimental diets were presented in Table . On day 35, six hens were randomly selected from each group and were euthanized. Duodenum, jejunum and ileum samples were collected and fixed in 10% phosphate-buffered formalin for morphological evaluation (Shang et al. Citation2020). The caecal contents were collected into 5 mL cryotubes and snap-frozen immediately in liquid nitrogen and stored at −80 °C until further microbial, metagenomics and untargeted metabolomics analysis.
Table 1. Ingredient composition and nutrient levels of the diet, (%, as-fed basis).
Layer Performance and egg quality
Egg numbers and weight were recorded daily. Laying rate was calculated from the total number of eggs divided by the total number of days. Average daily feed intake (ADFI) was determined according to the daily residual feed. Egg mass was calculated as egg weight × egg production. The feed conversion ratio (FCR) was calculated as grams of feed intake per gram of egg mass produced (Xiang et al. Citation2019). At the end of the experiment, six eggs were randomly collected from each replicate for determining egg quality, including yolk colour, shape index, albumen height, yolk height, shell thickness and haugh unit. The relative weight of yolk, albumin and eggshell was determined by the method provided by Abdollahi et al. (Citation2021).
Intestinal morphology
Intestinal samples were gradually dehydrated through increasing concentrations of ethanol (70 to 100%) and further cleared with xylene and embedded in paraffin. Paraffin blocks were sliced using a microtome into five-micron slices and stained with haematoxylin and eosin. The software of Image-Pro Plus (Media Cybernetics, USA) was used to determine the morphometric measurements of villus height (VH), crypt depth (CD) and the muscular thickness (MT) of the duodenum, jejunum and ileum by using an Olympus CX41 microscope (Olympus Cooperation, Japan). VH was measured from the tip to the base of villus and diameter, and CD was defined as the depth of the invagination between adjacent villi (Shang et al. Citation2020).
16 s rRNA gene sequencing analysis
Total DNA was extracted and quantified from 0.5 g of each caecal sample using a E.Z.N.A® Soil DNA Kit (Omega Biotek, Norcross, GA, USA) following the manufacturer's instructions. The final DNA concentration and purity were determined by a NanoDrop 2000 UV–vis spectrophotometer (Thermo Scientific, Wilmington, NC, USA), and DNA quality was checked by 1% agarose gel electrophoresis. The V3-V4 hypervariable regions of the bacterial 16S rRNA gene were amplified with 338 F (5′-ACTCCTACGGGAGGCAGCAG-3′) and 806 R (5′-GGACTACHVGGGTWTCTAAT- 3′) primers. PCR reactions were performed in triplicate with each 20 µL reaction mixture containing 4 µL of 5 × FastPfu Buffer, 2 µL of 2.5 mM dNTPs, 0.8 µL of each primer (5 µM), 0.4 µL FastPfu Polymerase and 10 ng template DNA. The PCR programs were 95 °C for 3 min; 95 °C for 30 s, 55 °C for 30 s and 72 °C for 45 s, repeat for 27 cycles; 72 °C for 10 min. The resulting PCR products were extracted from a 2% agarose gel and further purified using an AxyPrep DNA Gel Extraction Kit (Axygen Biosciences, Union City, CA, USA) and quantified using QuantiFluorTM-ST (Promega, USA) according to the manufacturer’s protocol. Sequencing libraries were generated using a NEXTFLEX Rapid DNA-Seq Kit (Bioo Scientific, USA) following the manufacturer's instructions. Finally, the library was sequenced on an Illumina MiSeq PE300 platform (Illumina, San Diego, CA, USA) according to standard protocols, by Majorbio Bio-Pharm Technology Co., Ltd. (Shanghai, China).
Sequence splicing and quality control, operational taxonomic units (OTUs) clustering and bioinformatics were carried out and analysed at http://www.isanger.com/ (Majorbio Bio-Pharm Technology Co., Ltd., China). Paired-end reads were trimmed by cutting off the barcode and primer sequence using Cutadapt software (Martin, Citation2011) to generate the raw reads. Then, chimeric sequences of the raw reads were removed using the USEARCH software based on UCHIME algorithm, and the clean reads were obtained (Edgar et al., Citation2011). The clean reads were clustered into operational taxonomic units (OTUs) based on a 97% similarity threshold using Uparse software. Taxonomy assignment of OTUs was performed by RDP Classifier against the Silva (SSU123) Database using a confidence threshold of 70%. OTU abundance data were normalised using a standard sequence number corresponding to the sample with the fewest sequences. Subsequent statistical analyses of alpha diversity was performed based on these normalised output data. Alpha diversity was used to analyse the complexity of species diversity through observed species Chao1, Ace, Shannon and Simpson diversity indices. The LEfSe analysis was performed with LEfSe software, and the screening value, the linear discriminant analysis (LDA) score, was 2.5. The functional capabilities of microbial communities were determined by using ‘Tax4Fun’ based on the SILVA database. Tax4Fun converted the 16S taxonomies to the prokaryotic taxonomies for the KEGG database (Kyoto Encyclopaedia of Genes and Genomes). Then, the description and functional information of each prokaryote was obtained from the KEGG database (http://www.genome.jp/kegg/) (Ao et al. Citation2021).
Untargeted metabolomics analysis
Caecal contents (100 mg) from 12 chickens (n = 6 per group) were individually grounded with liquid nitrogen and the homogenate was resuspended with prechilled 80% methanol and 0.1% formic acid by well vortex. The samples were incubated on ice for 5 min and then were centrifuged at 15,000 × g, 4 °C for 5 min. Some of supernatant was diluted to final concentration containing 53% methanol by LC-MS grade water. The samples were subsequently transferred to a fresh Eppendorf tube and then were centrifuged at 15,000 × g, 4 °C for 10 min. Finally, the supernatant was injected into the LC-MS/MS system analysis (Want et al. Citation2006). UHPLC-MS/MS analyses were performed using a Vanquish UHPLC system (Thermo Fisher, Germany) coupled with an Orbitrap Q ExactiveTM HF-X mass spectrometer (Thermo Fisher, Germany) in Novogene Co., Ltd. (Beijing, China). Samples were injected onto a Hypesil Gold column (100 × 2.1 mm, 1.9 μm) using a 17-min linear gradient at a flow rate of 0.2 mL/min. The eluents for the positive polarity mode were eluent A (0.1% FA in Water) and eluent B (Methanol). The eluents for the negative polarity mode were eluent A (5 mM ammonium acetate, pH 9.0) and eluent B (Methanol). The solvent gradient was set as follows: 2% B, 1.5 min; 2-100% B, 12.0 min; 100% B, 14.0 min; 100-2% B, 14.1 min; 2% B, 17 min. Q ExactiveTM HF-X mass spectrometer was operated in positive/negative polarity mode with spray voltage of 3.2 kV, capillary temperature of 320 °C, sheath gas flow rate of 40 arb and aux gas flow rate of 10 arb.
The raw data files generated by UHPLC-MS/MS were processed using the Compound Discoverer 3.1 (CD3.1, Thermo Fisher) to perform peak alignment, peak picking and quantitation for each metabolite. Peak intensities were normalised to the total spectral intensity. The normalised data was used to predict the molecular formula based on additive ions, molecular ion peaks and fragment ions. And then peaks were matched with the mzCloud (https://www.mzcloud.org/), mzVault and MassList database to obtain the accurate qualitative and relative quantitative results.
Data analysis
Data were analysed using a one-way analysis of variance (ANOVA) procedure in SPSS 20.0 (SPSS Inc., Chicago, IL, USA). The replicate pen was used as the experimental unit. Variability in the data was expressed as the standard error of means. A probability value p < .05 was considered statistically significant and p < .10 was considered as a trend. The Duncan’s Multiple Range Test was employed to gauge differences between the treatments. Partial least squares discriminant analysis (PLS-DA) were performed at metaX. We applied univariate analysis (t-test) to calculate the statistical significance (p value). The metabolites with the Variable Importance in the Projection (VIP) values > 1, p value <.05 and Fold Change (FC) ≥ 1.5 or ≤ 0.667 were considered to be differential metabolites (Sreekumar et al. Citation2009; Haspel et al. Citation2014; Heischmann et al. Citation2016).
Results
Feeding layers with 6% AM containing diet had higher egg production ratio (p < .10) and egg mass (p < .05) than those fed with the CON group diet. In addition, FCR in AM3 and AM6 groups were lower than in other groups (p < .05). However, the ADFI and egg weight did not differ among all groups (Table ).
Table 2. Effect of dietary supplementation of alfalfa meal (AM) on laying performance of Dagu chicken.
Dietary supplementation of AM had no significant effects on the egg shape index, yolk height, eggshell thickness, yolk weight and eggshell weight. However, AM supplementation positively affected the yolk colour, in which AM9 group had the highest value (p < .05). In addition, feeding layers with 9% AM containing diet had higher albumin height (p < .10) and haugh unit (p < .10) than those in the CON group (Table ).
Table 3. Effect of dietary supplementation of alfalfa meal (AM) on egg quality of Dagu chicken.
In the duodenum, the VH in the AM3, AM6 and AM9 groups (p < .05), the VH:CD ratio in the AM3 and AM6 groups (p < .05) and the MT in the AM9 group (p < .10) were significantly higher than that in the CON group. In the jejunum, the VH and VH:CD ratio in AM3 and AM6 groups (p < .05) and the MT in AM3, AM6 and AM9 groups (p < .05) were significantly higher than that in the CON group. Additionally, CD in AM9 group (p < .05) was higher than that in AM6 group. In the ileum, the VH, VH:CD ratio and MT in AM3, AM6 and AM9 groups (p < .05) were significantly higher than that in the CON group, whereas the CD in AM3, AM6 and AM9 groups (p < .05) was significantly lower than that in the CON group (Table ).
Table 4. Effect of dietary supplementation of alfalfa meal (AM) on intestinal morphology of Dagu chicken.
The Chao1 index and Ace index are two indicators used to estimate species richness. The species richness of three AM containing groups was significantly higher than CON group (p < .05). Shannon and Simpson diversity value are indices used to estimate the microbial diversity in the samples. The Shannon indices in AM6 and AM9 groups were significantly higher than those in CON group (p < .05). The Simpson indices in three AM containing groups were significantly lower than that in the CON group (p < .05) (Table ).
Table 5. Effect of dietary supplementation of alfalfa meal (AM) on diversity indices of caecal microbiota of Dagu chicken.
Principal Component Analysis (PCA) reflects differences between samples in a two-dimensional coordinate map; the more similar the composition of the microbiota between the samples, the closer the distance representing their coordinate points in the PCA plot. At the genus level, the 3%, 6% and 9% AM containing groups were close to each other in their projection plane, so it was difficult to distinguish them. However, the CON group was most away from the other experimental groups, scattered in different quadrants and can be distinguished (Figure ).
Figure 1. Principal component analysis (PCA) of the dissimilarity among the microbial samples at the genus levels. The values of axes 1 and 2 are the percentages that can be explained by the corresponding axis.
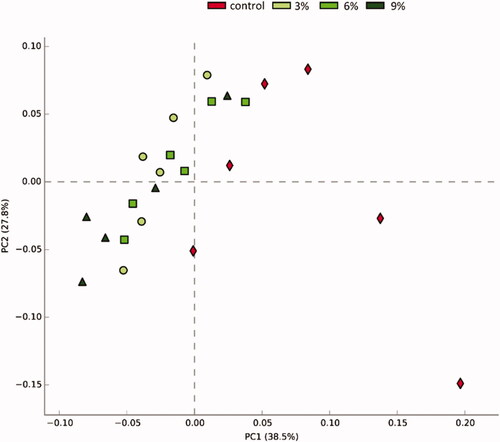
A relative abundance histogram was constructed using the species information and abundance of all samples at the phylum level. Bacteroidetes, Firmicutes, Proteobacteria, Spirochaetes and Synergistetes were the dominant phyla (Figure ). The Firmicutes/Bacteroidetes ratio in the CON group was higher than in other experimental groups (Figure ). The relative abundance of Synergistetes in caecum in the CON group was lower than that in the other experimental groups (Figure ). A relative abundance histogram was drawn using the top 20 species information and abundance of all samples at the genus level. Bacteroides, Rikenellaceae_RC9_gut_group and unclassified_o_Bacteroidales were the top three dominant genera (Figure ).
Figure 2. Effects of various levels of alfalfa meal supplementation on the relative abundance of intestinal microbiota at the phylum level.
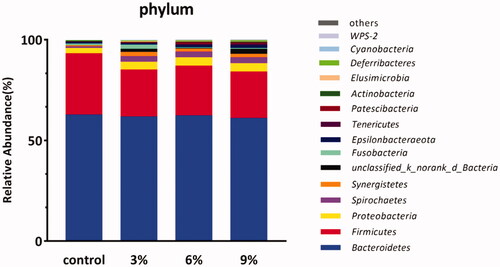
Figure 3. Effect of various levels of alfalfa meal supplementation on the Firmicutes/Bacteroidetes ratio in caecal.
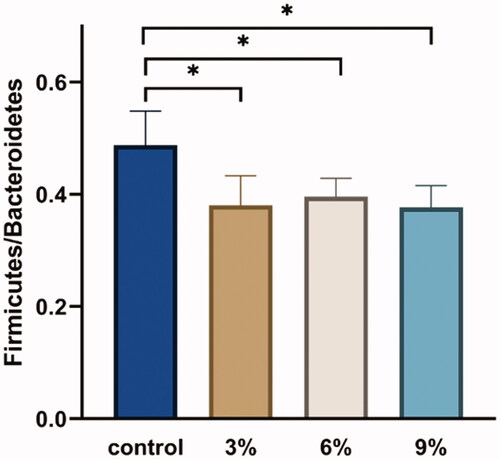
Figure 4. Effect of various levels of alfalfa meal supplementation on the relative abundance of Synergistetes in caecal.
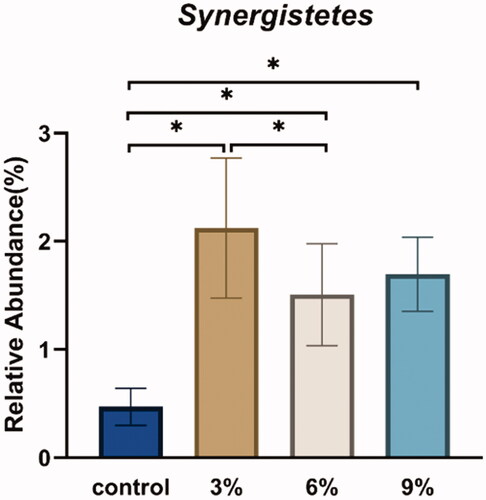
Figure 5. Effect of various levels of alfalfa meal supplementation on the relative abundance of intestinal microbiota at the genus level.
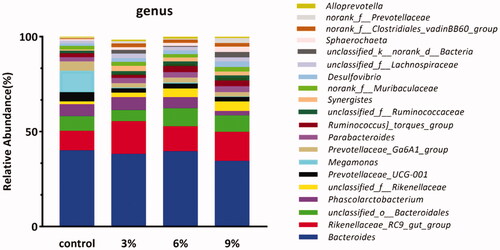
Lefse was used to compare the differences at the genus level among groups. Lefse analysis showed that there were 24 biomarkers with LDA scores > 2.5. There were four taxa in the CON group, six taxa in the AM3 group, five taxa in the AM6 group and nine taxa in the AM9 group (Figure ).
Figure 6. The LDA value (influence value of linear discriminant analysis) distribution histogram shows bacterial community or species (Biomarker) with LDA score greater than 2.5. All the bacterial communities or species displayed have statistical differences among all groups. The length of histogram represented the influence of Biomarkers.
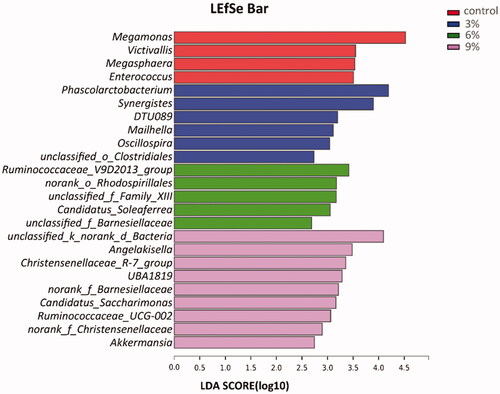
Correlation analysis was manifested by a heatmap and conducted between the 24 biomarkers at genus level and laying rate, ADFI and FCR. The threshold |R| > 0.5 was used to establish the existence of a correlation. The results indicated that Enterococcus was positively correlated with FCR but negatively correlated with laying rate. Phascolarctobacterium and Candidatus_Soleaferrea were negatively correlated with FCR (Figure ).
Figure 7. Heatmap of the correlation analysis between laying rate, average daily feed intake (ADFI) and feed conversion ratio (FCR) at the genus level. ∗.01 < p ≤ .05; ∗∗.001 < p ≤ .01.
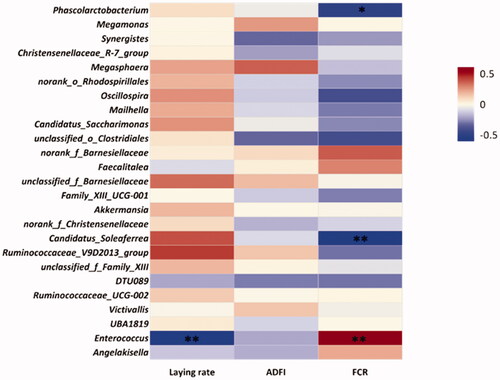
The KEGG function of each sample flora was predicted by Tax4Fun. A total of 40 prediction functions were obtained at level 2. Using PCA, it was difficult to distinguish the functional abundance at pathway level 2 among three experimental groups, but there was a substantive difference between the CON group and other experimental groups, because they were located in different quadrants (Figure ). Therefore, the three experimental groups were combined into one group to analyse the functional differences at pathway level 2 compared to the CON group. Metabolic pathways of cofactors and vitamins, nucleotide, translation, replication and repair, infectious disease: bacterial, cancer: overview, cancer: specific types, transcription and infectious disease: viral were lower than CON group. However, metabolic pathways of carbohydrate metabolism, cellular community-eukaryotes, cellular community-prokaryotes, environmental adaptation, infectious disease: parasitic, lipid metabolism, metabolism of terpenoids and polyketides, neuro-degenerative disease and signal transduction were higher than CON group (Figure ).
Figure 8. Principal component analysis (PCA) evaluated the predicted microbial gene functions changes of the caecal microbiota at KEGG level 2 among groups.
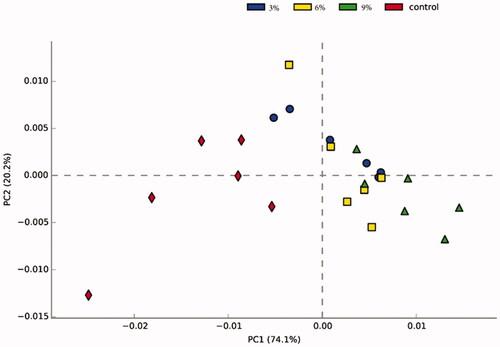
Figure 9. Predicted microbial gene functions of the caecal microbiota with significant differences at KEGG level 2 between control group and alfalfa meal supplementation.
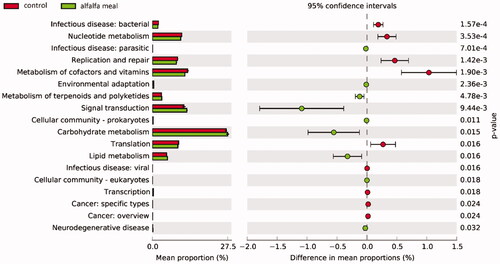
The positive and negative ion data were subjected to further statistical analysis using PLS-DA. The results showed that the parameters were clearly separated between CON and AM6 groups (Figure ).
Figure 10. PLS-DA analysis score of quality control under the positive ion mode (a) and negative ion model (b).
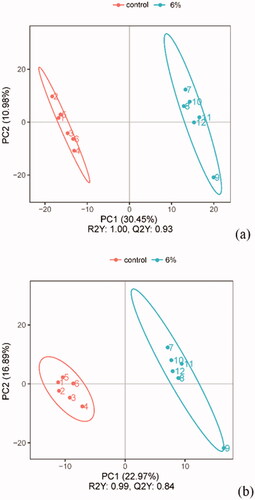
A total of 397 differential metabolites have been identified between AM6 group and CON group (VIP > 1, p < .05 and FC ≥ 1.5 or FC ≤ 0.667). Among them, a total of 176 metabolites from AM6 group were upregulated, whereas 221 metabolites were downregulated (Table ). The Volcano plot of the distribution of differential metabolites between AM6 and CON groups was shown in Figure .
Figure 11. Volcano plot by metabolomics analysis under the positive ion mode (a) and negative ion mode (b).
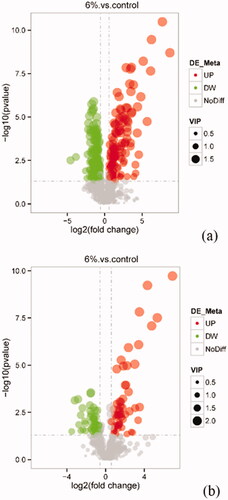
Table 6. Differential metabolites in the caecal of chicks between alfalfa meal 6 (AM6) group and CON group.
In compared with the CON group, the FAHFA (11:0/19:2), 18-β-Glycyrrhetinic acid, Isoliquiritigenin, etc. were upregulated, whereas the Prostaglandin E1, THJ2201 N-pentanoic acid metabolite, etc. were downregulated in the AM6 group (Table ).
Table 7. Top 30 differential metabolites in the caecal of chicks between alfalfa meal 6 (AM6) group and CON group.
Discussion
Feeding layers with AM containing diet has been reported to impair productive performance (Mourão et al. Citation2006; Englmaierova et al. Citation2019). Mourão et al. (Citation2006) reported that the egg mass was decreased in layers consuming 15.1% dehydrated AM containing diet. Englmaierova et al. (Citation2019) noted that layers fed with 4% dehydrated AM containing diet decreased egg production rate and egg mass. However, in the present study, dietary inclusion of AM had no negative effects on the productive performance, but improved egg production rate and egg mass and reduced FCR. The mean egg production rate and egg weight were 62.14% and 62.50 g, respectively, in this study, which was in line with the standards of Dagu chicken production, in which the egg production rate and egg weight during ages of 35 to 39 weeks were 62.60 ± 2.00% and 62.2 ± 0.7g, respectively (Su et al. Citation2014). Yıldız et al. (Citation2020) reported that the high cellulose contents in the AM were one of the main limiting factors of its utilisation in poultry diets. Dagu chickens, also known as grass chicken, have the characteristic of herbivorous habits, presenting in stronger tolerance to crude fibre compared with the commercial chicken. Similarly, Zheng et al. (Citation2019) noted that feeding Beijing-you chickens with 5% AM containing diet decreased FCR and without inducing any negative effects on productive performance. Therefore, we considered that the differences with the above studies were related to the different breeds of chickens. On the other hand, the growth and development of ovarian follicles were accompanied by a series of complicated biochemical and physiological changes in the reproductive system of layers. Hormones play a key role in inducing follicular development (Yang et al. Citation2018). Alfalfa is the most widespread perennial legumes and has been reported to be rich in a series of phytoestrogens ingredients such as apigenins (135–833 μg/g dry matter), luteolins (51–151 μg/g of dry matter), coumestrols (86–257 μg/g of dry matter) and quercetin (30–75 μg/g of dry matter) (Seguin et al. Citation2004; Seguin and Zheng Citation2006; Tucak et al. Citation2018). Due to the structure of phytoestrogens similar to the endogenous oestrogen 17-β-estradiol, it can interact with oestrogen receptors and further lead to oestrogenic and antiestrogenic effects (Landete et al. Citation2016; Peirotén et al. Citation2020; Stojanov and Kreft Citation2020). Liu and Zhang (Citation2008) reported that dietary supplementation of daidzein improved follicle development and laying performance in layers after the peak laying period by upregulating mRNA expression of gonadotropin receptors. Yang et al. (Citation2018) proved that feeding laying hens with phytoestrogen quercetin containing diet had positive effects on the egg production rate and FCR. Saleh et al. (Citation2019) noted that inclusion of mixed sources of phytoestrogens in diets improved laying performance, egg quality, antioxidative status, hormonal profiles and steroidogenesis in aged laying hens. Therefore, adding appropriate amounts of phytoestrogens to poultry diet had positive effects on the laying performance. In this study, the egg production rate and egg mass were significantly increased in the AM6 group in comparison with the CON group, while not AM3 and AM9 groups. It can be predicted that the higher containing of AM in the diet, higher contents of phytoestrogens and fibre will be. Therefore, we speculated that dietary inclusion of 3% AM had no significant effects on the productive performance of layers probably related to the contents of phytoestrogens was low, while in AM9 group probably related to the high contents of fibre, which indicated that 9% AM is too much for Dagu chickens. We considered that the 6% AM was a suitable dose for optimising the productive performance in Dagu chickens.
Plenty of studies has reported that dietary supplementation of AM had positive effects on the yolk colour. Mourão et al. (Citation2006) reported that feeding layer hens with 15.1% dehydrated AM containing diet increased yolk colour, presenting an increase in yellowness. Zheng et al. (Citation2019) noted that Beijing-you chickens fed the diet supplemented with 5, 8 or 10% AM increased yolk colour. In this study, feeding Dagu chickens with AM containing diet had positive effects on the yolk colour. The colour of egg yolk is due to the accumulation of carotenoids in the egg. However, hens cannot synthesise carotenoids (Nys et al. Citation2011). Therefore, the exogenous carotenoids supplementation was beneficial to improve the yolk colour. It has been reported that the AM was rich in carotene (Nys Citation2000; Alay and Karadas Citation2016; Yıldız et al. Citation2020). Therefore, the inclusion of AM improved yolk colour was related to the contents of carotene riched in the AM. Haugh unit is a unit that expresses the degree of thinning of thick protein, which is related to the protein content in the thicker whites (Eisen et al. Citation1962). In the present study, feeding Dagu chickens with 9% AM containing diet improved the haugh unit. Similarly, Kocaoğlu Güçlü et al. (Citation2004) reported that dietary supplementation of 9% AM improved haugh unit in 10-week-age quails. Additionally, the increase of albumen height was observed in AM9 group, which indicated that dietary inclusion of 9% AM had positive effects on the protein anabolic, attributing to the flavonoid components presented in the AM (Yoshioka et al., Citation2018; Otsuka et al., Citation2019). Therefore, we considered that the Dagu chickens fed the AM containing diet would increase the acceptance of eggs by consumers through improving haugh unit, albumen height and yolk colour.
The AM is rich in fibre. Poultry requires a certain amount of dietary fibre for maintaining normal intestinal physiology functions (Adams et al. Citation2019; Dang et al. Citation2022). In previous studies, supplementing fibre to the diet of poultry increased VH, villus thickness and villi to crypt proportions (Chiou et al. Citation1996; Sklan et al. Citation2003; Rezaei et al. Citation2018), which was consistent with the current study. The increase in VH might be due to the production of short-chain fatty acids by dietary fibre fermentation, thus promoting mucosal epithelium proliferation (Knudsen et al. Citation2012). It also might be due to the physical wear and tear on the intestinal mucosa caused by high level fibres, resulting in the renewal of intestinal mucosal epithelial cells. In addition, it has been reported that high fibre levels in poultry diets inevitably increased the endogenous loss of crude proteins and amino acids (Kluth and Rodehutscord Citation2009). The increase in VH leads to more cell turnover, which may increase the demand for amino acids that are important to intestinal function (Tejeda and Kim Citation2021). Therefore, as the results observed in this study, dietary supplementation of AM was considered as a suitable method to play a positive role in promoting intestinal development and reducing endogenous damage in intestinal epithelium.
Bacteria that existed in the intestine play an important role in human and animal health (Adams et al. Citation2019). In-depth studies of chicken intestinal microbiota were essential to understand their role in host function (Zheng et al. Citation2019). Therefore, high-throughput 16S rRNA sequencing technology was used to evaluate the abundance and diversity of caecal microbiota in Dagu chickens. The results showed that the abundance and diversity of caecal microbiota were significantly increased in AM supplemented groups compared to the CON group, but no significant difference has been observed between AM supplemented groups, which was confirmed by the studies of Escarcha et al. (Citation2012) and Jiang et al. (Citation2014). However, Zheng et al. (Citation2019) reported that dietary supplemented with 5, 8 or 10% AM had no significant effects on the caecal microbiota abundance and diversity. Different from the above study might be due to the difference in genetic background between experimental animals.
The caecum is the location with the most bacteria richness in the gastrointestinal tract of chicks (Salanitro et al. Citation1974; Videnska et al. Citation2013). The absolute counts of bacteria in the caecum are approximately 1010 CFU per gram of digestion, which is composed of about 1000 different species (Rychlik Citation2020). The two main phyla in the caecum of poultry are Firmicutes and Bacteroidetes (Nordentoft et al. Citation2011; Oakley et al. Citation2014; Waite and Taylor Citation2014; Wang et al. Citation2016). In this study, Bacteroidetes and Firmicutes were also the dominant phyla in the caecal microbiota of Dagu chickens. The abundance of Bacteroides (around 60%) did not significantly differ among groups. However, the abundance of Firmicutes in the AM3, AM6 and AM9 groups was decreased by 22.5%, 18% and 25.4%, respectively, in comparison with the CON group. In recent years, the ratio of Firmicutes/Bacteroidetes was considered a biomarker of obesity. It has been reported that the intestinal microbiota in obese animals and humans were characterised by a higher Firmicutes/Bacteroidetes ratio than normal-weight individuals (Ley et al. Citation2005). In this study, diet supplemented with AM significantly decreased the Firmicutes/Bacteroidetes ratio, which might be indicated that the supplementation of AM reduced fat contents in Dagu chickens. Similarly, Jiang et al. (Citation2012) reported that supplementing AM to the diet of ducks decreased the production of abdominal fat. Zheng et al. (Citation2019) noted that the abdominal fat production and yolk cholesterol contents were significantly decreased in Beijing-you chickens fed the diet supplemented with AM. However, more studies were needed to be conducted to evaluate the effects of dietary supplementation of AM on the fat contents in Dagu chickens. In addition, in this study, Synergistetes at the phylum level exhibited significant differences between dietary groups. Synergistites have been reported to degrade amino acids, peptides and proteins (Jumas-Bilak and Marchandin Citation2014). In this study, the supplementation of AM derived from the alfalfa leaves existed high protein contents, which indicated that the protein contents in AM supplemented groups were corresponding increased. Despite the composition of diet in all groups being based on the principle of equal nitrogen and energy, however, the adjustment of protein contents in CON group was used by corn flour. Compared to the protein from corn flour, alfalfa protein was more likely to induce the proliferation of Synergistetes in the caecum, which might be related to the different composition and proportion of amino acids between alfalfa protein and corn flour protein.
The results of LEfSe at the genus level indicated that 24 items were different among dietary groups, the lowest value was shown in the CON group. With the levels of AM increased, nine different items have been found in AM9 group, while the differences in AM3 and AM6 groups were similar. This indicated that the bacteria in the caecum have proliferated by different degrees with the increase of dietary AM levels, which was probably related to the preference for specific substrates of bacteria being different (Castillo et al. Citation2007). However, whether the reason for this change was related to the variety of fibre composition and bioactive substances in AM, the age of birds or the environmental conditions were needed to be explored further.
Intestinal bacteria are closely associated with the production performance of birds. In this study, we found that the levels of Phascolarctobacterium were negatively correlated with FCR. Phascolarctobacterium is a substantial acetate/propionate producer and belongs to the phylum Firmicutes. It has been reported that the levels of Phascolarctobacterium were not only positively correlated with good moods in humans, but also positively correlated to the body weight gain, fat mass, plasma leptin levels in high-fat diet treated rats (Lecomte et al. Citation2015; Wu et al. Citation2017; Gan et al. Citation2020). In this study, the abundance of Phascolarctobacterium in AM9 group was significantly lower than in other groups, and the highest value of FCR has been found in AM9 group. This means that the positive effects of AM supplementation on FCR were diminished when the proportion of AM reached 9%, which might be related to the significant reduction in Phascolarctobacterium levels. In addition, Enterococcus has been reported to induce positive correlation effect on FCR, but induce negative correlation effect on laying rate. Enterococcus can infect birds in the process of hatching, rearing or processing under modern poultry production environment through various routes, such as respiratory system, gastrointestinal tract and integument. After infection, bacteria circulate in the bloodstream and form abscesses, further causing arthritis, osteomyelitis, spondylitis and femoral head necrosis in broilers (Stalker et al. Citation2010; Kolbjørnsen et al. Citation2011; Wideman Citation2016; Song et al. Citation2019). In this study, we found that the abundance of Enterococcus in AM3 and AM6 groups were lower than in other groups, which probably indicated that the AM in 3% or 6% levels had inhibition effects on the proliferation of Enterococcus in the caecum.
The functional annotation was performed to understand the effects of AM supplementation on caecal microbiota community functions in Dagu chickens. The microbial abundant functions were evaluated by level 2 annotations. The results observed in this study indicated that the potential functions of caecal microbiota in the AM-treated groups were different from those in CON group. Microbiota communities in caecum had positive effects on the carbohydrate metabolism, lipid metabolism and metabolism of terpenoids and polyketides, which might be due to the increase of fibre contents and various bioactive components such as terpenoids, polyketides and saponin in the intestine by AM supplementation, thus inducing the proliferation of bacteria that metabolise carbohydrates, terpenoids and polyketides. Saponin and cellulose have been reported to reduce the absorption of cholesterol by binding to the cholesterol in the intestine (Francis et al. Citation2002), thus increasing the contents of lipids in the intestinal tract and subsequently induced the proliferation of bacteria that metabolise lipids.
The AM6 group and CON group were selected based on the criteria of growth performance and egg quality of Dagu chickens for conducting the metabolomics analysis in caecal contents. A total of 397 differential metabolites have been identified among the groups. The top three differential metabolites with the highest VIP value in AM6 group were FAHFA (11:0/19:2), 18-β-Glycyrrhetinic acid, Isoliquiritigenin. FAHFAs are a class of bioactive lipids, show great promise for treating diabetes and inflammatory diseases (Yore et al. Citation2014; Tan et al. Citation2019). 18-β-glycyrrhetinic acid is an active constituent of glycyrrhizic acid, showing an anti-viral against effects on a number of DNA and RNA viruses, which is possibly due to the activation of nuclear factor (NF-κB and induction of IL-8 secretion) (Shaneyfelt et al. Citation2006; Omer et al. Citation2014). Isoliquiritigenin is a natural flavonoid extracted from the liquorice, exists anti-diabetic, anti-cancer, anti-oxidant and anti-inflammatory properties (Sekine‐Osajima et al. Citation2009; Chen et al. Citation2012; Gaur et al. Citation2014). Isoliquiritigenin has been identified from the flavonoids of alfalfa (Chen et al. Citation2020). Therefore, we considered that the addition of AM would improve the concentrations of metabolites of antioxidant, anti-viral and anti-inflammation in the intestine, thus beneficial to the health of the host.
Conclusion
In this study, feeding Dagu chickens with AM containing diet had positive effects on the laying performance, egg quality, small intestinal morphology, caecal microbiota diversity and caecal metabolic function. Therefore, feeding Dagu chickens with AM containing diet was a suitable strategy for improving productive performance under cage-reared system. The optimum dose of AM was 6%.
Ethical approval
The Ethical approval for the present study was obtained from the Ethical Committee of Jinzhou Medical University.
Disclosure statement
No potential conflict of interest was reported by the author(s).
Data availability statement
The data that support the findings of this study are available from the corresponding author upon reasonable request.
Additional information
Funding
References
- Abdollahi A, Karimi A, Sadeghi AA, Bedford MR, Ashengroph M. 2021. The effects of the fiber source and xylanase supplementation on production, egg quality, digestibility, and intestinal morphology in the aged laying hen. Poultr Sci. 100(3):100936.
- Adams S, Xiangjie K, Hailong J, Guixin Q, Sossah FL, Dongsheng C. 2019. Prebiotic effects of alfalfa (Medicago sativa) fiber on cecal bacterial composition, short-chain fatty acids, and diarrhea incidence in weaning piglets. RSC Adv. 9(24):13586–13599.
- Alay T, Karadas F. 2016. The effects of carotenoids in quail breeder diets on egg yolk pigmentation and breeder performance. Acta Agric Scand Sect A Anim Sci. 66(4):206–214.
- Al-Rawi B, Craig JV, Adams AW. 1976. Agonistic behavior and egg production of caged layers: genetic strain and group-size effects. Poultr Sci. 55(2):796–807.
- Ao Y, Yang C, Wang S, Hu Q, Yi L, Zhang J, Yu Z, Cai M, Yu C. 2021. Characteristics and nutrient function of intestinal bacterial communities in black soldier fly (Hermetia illucens L.) larvae in livestock manure conversion. Microb Biotechnol. 14(3):886–896.
- Castillo M, Martín-Orúe SM, Nofrarías M, Manzanilla EG, Gasa J. 2007. Changes in caecal microbiota and mucosal morphology of weaned pigs. Vet Microbiol. 124(3-4):239–247.
- Chen S, Li X, Liu X, Wang N, An Q, Ye XM, Zhao ZT, Zhao M, Han Y, Ouyang KH, et al. 2020. Investigation of chemical composition, antioxidant activity, and the effects of alfalfa flavonoids on growth performance. Oxid Med Cell Longev. 2020:8569237.
- Chen X, Wu Y, Jiang Y, Zhou Y, Wang Y, Yao Y, Yi C, Gou L, Yang J. 2012. Isoliquiritigenin inhibits the growth of multiple myeloma via blocking IL-6 signaling. J Mol Med. 90(11):1311–1319.
- Chiou PW, Lu TW, Hsu JC, Yu B. 1996. Effect of different sources of fiber on the intestinal morphology of domestic geese. Asian Australas J Anim Sci. 9(5):539–550.
- Dang DX, Cho S, Wang H, Seok WJ, Ha JH, Kim IH. 2022. Quercetin extracted from Sophora japonica flower improves growth performance, nutrient digestibility, cecal microbiota, organ indexes, and breast quality in broiler chicks. Anim Biosci. 35(4):577–586.
- de Jong IC, Fillerup M, Blokhuis HJ. 2005. Effect of scattered feeding and feeding twice a day during rearing on indicators of hunger and frustration in broiler breeders. Appl Anim Behav Sci. 92(1-2):61–76.
- Dishman DL, Thomson DM, Karnovsky NJ. 2009. Does simple feeding enrichment raise activity levels of captive ring-tailed lemurs (Lemur catta)? Appl Anim Behav Sci. 116(1):88–95.
- Dong XF, Gao WW, Tong JM, Jia HQ, Sa RN, Zhang Q. 2007. Effect of polysavone (alfalfa extract) on abdominal fat deposition and immunity in broiler chickens. Poultr Sci. 86(9):1955–1959.
- Edgar RC, Haas BJ, Clemente JC, Quince C, Knight R. 2011. UCHIME improves sensitivity and speed of chimera detection. Bioinformatics. 27(16):2194–2200.
- Eisen EJ, Bohren BB, McKean HE. 1962. The Haugh unit as a measure of egg albumen quality. Poultr Sci. 41(5):1461–1468.
- Englmaierova M, Skřivan M, Vit T. 2019. Alfalfa meal as a source of carotenoids in combination with ascorbic acid in the diet of laying hens. Czech J Anim Sci. 64( 1):17–25.
- Escarcha JF, Callaway TR, Byrd JA, Miller DN, Edrington TS, Anderson RC, Nisbet DJ. 2012. Effects of dietary alfalfa inclusion on Salmonella typhimurium populations in growing layer chicks. Foodborne Pathog Dis. 9(10):945–951.
- Fossum O, Jansson DS, Etterlin PE, Vagsholm I. 2009. Causes of mortality in laying hens in different housing systems in 2001 to 2004. Acta Vet Scand. 51(1):9.
- Francis G, Kerem Z, Makkar HP, Becker K. 2002. The biological action of saponins in animal systems: a review. Br J Nutr. 88(6):587–605.
- Fu D, Zhang D, Xu G, Li K, Wang Q, Zhang Z, Li J, Chen Y, Jia Y, Qu L. 2015. Effects of different rearing systems on meat production traits and meat fiber microstructure of Beijing-you chicken. Anim Sci J. 86(7):729–735.
- Gan L, Zhao Y, Mahmood T, Guo Y. 2020. Effects of dietary vitamins supplementation level on the production performance and intestinal microbiota of aged laying hens. Poultr Sci. 99(7):3594–3605.
- Gaur R, Yadav KS, Verma RK, Yadav NP, Bhakuni RS. 2014. In vivo anti-diabetic activity of derivatives of isoliquiritigenin and liquiritigenin. Phytomedicine. 21(4):415–422.
- Haspel JA, Chettimada S, Shaik RS, Chu J-H, Raby BA, Cernadas M, Carey V, Process V, Hunninghake GM, Ifedigbo E, et al. 2014. Circadian rhythm reprogramming during lung inflammation. Nat Commun. 5(1):1–15.
- Heischmann S, Quinn K, Cruickshank-Quinn C, Liang LP, Reisdorph R, Reisdorph N, Patel M. 2016. Exploratory metabolomics profiling in the kainic acid rat model reveals depletion of 25-hydroxyvitamin D3 during epileptogenesis. Sci Rep. 6:1–14.
- Jiang JF, Song XM, Huang X, Wu JL, Zhou WD, Zheng HC, Jiang YQ. 2012. Effects of alfalfa meal on carcase quality and fat metabolism of Muscovy ducks. Br Poult Sci. 53(5):681–688.
- Jiang JF, Song XM, Wu JL, Jiang YQ. 2014. Effects of alfalfa meal on the intestinal microbial diversity and immunity of growing ducks. J Anim Physiol Anim Nutr. 98(6):1039–1046.
- Jumas-Bilak E, Marchandin H. 2014. The phylum synergistetes. In: Rosenberg E, DeLong EF, Lory S, Stackebrandt E, Thompson F, editors. The prokaryotes. Berlin: Springer. p. 931-954.
- Kluth H, Rodehutscord M. 2009. Effect of inclusion of cellulose in the diet on the inevitable endogenous amino acid losses in the ileum of broiler chicken. Poult Sci. 88(6):1199–1205.
- Knudsen KEB, Hedemann MS, Laerke HN. 2012. The role of carbohydrates in intestinal health of pigs. Anim Feed Sci Technol. 173(1-2):41–53.
- Kocaoğlu Güçlü B, Işcan KM, Uyanik F, Eren ME, Can Ağca A. 2004. Effect of alfalfa meal in diets of laying quails on performance, egg quality and some serum parameters. Arch Anim Nutr. 58(3):255–263.
- Kolbjørnsen Ø, David B, Gilhuus M. 2011. Bacterial osteomyelitis in a 3-week-old broiler chicken associated with Enterococcus hirae. Vet Pathol. 48(6):1134–1137.
- Landete JM, Arqués J, Medina M, Gaya P, de Las Rivas B, Muñoz R. 2016. Bioactivation of phytoestrogens: intestinal bacteria and health. Crit Rev Food Sci Nutr. 56(11):1826–1843.
- Laudadio V, Ceci E, Lastella NMB, Introna M, Tufarelli V. 2014. Low-fiber alfalfa (Medicago sativa L.) meal in the laying hen diet: effects on productive traits and egg quality. Poultr Sci. 93(7):1868–1874.
- Lecomte V, Kaakoush NO, Maloney CA, Raipuria M, Huinao KD, Mitchell HM, Morris MJ. 2015. Changes in gut microbiota in rats fed a high fat diet correlate with obesity-associated metabolic parameters. PLoS One. 10(5):e0126931.
- Ley RE, Bäckhed F, Turnbaugh P, Lozupone CA, Knight RD, Gordon JI. 2005. Obesity alters gut microbial ecology. Proc Natl Acad Sci U S A. 102(31):11070–11075.
- Liu HY, Zhang CQ. 2008. Effects of daidzein on messenger ribonucleic acid expression of gonadotropin receptors in chicken ovarian follicles. Poultr Sci. 87(3):541–545.
- Mabelebele M, Ng'ambi J, Norris D, Ginindza M. 2014. Comparison of gastrointestinal tract and pH values of digestive organs of Ross 308 broiler and indigenous Venda chickens fed the same diet. Asian J Anim Vet Adv. 9(1):71–76.
- Martin M. 2011. Cutadapt removes adapter sequences from high-throughput sequencing reads. EMBnet. journal. 17(1):10–2.
- Mourão JL, Ponte PIP, Prates JAM, Centeno MSJ, Ferreira LMA, Soares MAC, Fontes CMGA. 2006. Use of β-glucanases and β-1, 4-xylanases to supplement diets containing alfalfa and rye for laying hens: effects on bird performance and egg quality. J Appl Poult Res. 15(2):256–265.
- Nordentoft S, Mølbak L, Bjerrum L, De Vylder J, Van Immerseel F, Pedersen K. 2011. The influence of the cage system and colonisation of Salmonella Enteritidis on the microbial gut flora of laying hens studied by T-RFLP and 454 pyrosequencing. BMC Microbiol. 11:187.
- Nys Y. 2000. Dietary carotenoids and egg yolk coloration. Archiv Für Geflugelkunde. 64:45–54.
- Nys Y, Bain M, Van Immerseel F. 2011. Improving the safety and quality of eggs and egg products: volume 1: egg chemistry, production and consumption. Cambridge: Elsevier.
- Oakley BB, Lillehoj HS, Kogut MH, Kim WK, Maurer JJ, Pedroso A, Lee MD, Collett SR, Johnson TJ, Cox NA. 2014. The chicken gastrointestinal microbiome. FEMS Microbiol Lett. 360(2):100–112.
- Ochetim S. 1993. The feeding and economic value of maize cob meal for broiler chickens. Asian Australas J Anim Sci. 6(3):367–371.
- Omer MO, AlMalki WH, Shahid I, Khuram S, Altaf I, Imran S. 2014. Comparative study to evaluate the anti-viral efficacy of Glycyrrhiza glabra extract and ribavirin against the Newcastle disease virus. Pharmacognosy Res. 6(1):6–11.
- Onuh SO, Ortserga DD, Okoh JJ. 2010. Comparative evaluation of maize and soyabeans as energy sources for broiler chickens. Pak J Nutr. 9(6):520–523.
- Otsuka Y, Egawa K, Kanzaki N, Izumo T, Rogi T, Shibata H. 2019. Quercetin glycosides prevent dexamethasone-induced muscle atrophy in mice. Biochem Biophys Rep. 18:100618.
- Peirotén Á, Bravo D, Landete JM. 2020. Bacterial metabolism as responsible of beneficial effects of phytoestrogens on human health. Crit Rev Food Sci Nutr. 60(11):1922–1937.
- Rezaei M, Karimi Torshizi MA, Wall H, Ivarsson E. 2018. Body growth, intestinal morphology and microflora of quail on diets supplemented with micronised wheat fibre. Br Poult Sci. 59(4):422–429.
- Rychlik I. 2020. Composition and function of chicken gut microbiota. Animals. 10(1):103.
- Salanitro JP, Blake IG, Muirhead PA. 1974. Studies on the cecal microflora of commercial broiler chickens. Appl Microbiol. 28(3):439–447.
- Saleh AA, Ahmed EA, Ebeid TA. 2019. The impact of phytoestrogen source supplementation on reproductive performance, plasma profile, yolk fatty acids and antioxidative status in aged laying hens. Reprod Dom Anim. 54(6):846–854.
- Sebola NA, Mlambo V, Mokoboki HK, Muchenje V. 2015. Growth performance and carcass characteristics of three chicken strains in response to incremental levels of dietary Moringa oleifera leaf meal. Livestock Sci. 178:202–208.
- Seguin P, Zheng W. 2006. Phytoestrogen content of alfalfa cultivars grown in eastern Canada. J Sci Food Agric. 86(5):765–771.
- Seguin P, Zheng W, Souleimanov A. 2004. Alfalfa phytoestrogen content: impact of plant maturity and herbage components. J Agron Crop Sci. 190(3):211–217.
- Sekine-Osajima Y, Sakamoto N, Nakagawa M, Itsui Y, Tasaka M, Nishimura-Sakurai Y, Chen C-H, Suda G, Mishima K, Onuki Y, et al. 2009. Two flavonoids extracts from Glycyrrhizae radix inhibit in vitro hepatitis C virus replication. Hepatol Res. 39(1):60–69.
- Shaneyfelt ME, Burke AD, Graff JW, Jutila MA, Hardy ME. 2006. Natural products that reduce rotavirus infectivity identified by a cell-based moderate-throughput screening assay. Virol J. 3:68.
- Shang Q, Wu D, Liu H, Mahfuz S, Piao X. 2020. The impact of wheat bran on the morphology and physiology of the gastrointestinal tract in broiler chickens. Animals. 10(10):1831.
- Sklan D, Smirnov A, Plavnik I. 2003. The effect of dietary fibre on the small intestines and apparent digestion in the turkey. Br Poult Sci. 44(5):735–740.
- Song H, Bae Y, Jeon E, Kwon Y, Joh S. 2019. Multiplex PCR analysis of virulence genes and their influence on antibiotic resistance in Enterococcus spp. isolated from broiler chicken. J Vet Sci. 20(3):e26.
- Sreekumar A, Poisson LM, Rajendiran TM, Khan AP, Cao Q, Yu J, Laxman B, Mehra R, Lonigro RJ, Li Y, et al. 2009. Metabolomic profiles delineate potential role for sarcosine in prostate cancer progression. Nature. 457(7231):910–914.
- Stalker MJ, Brash ML, Weisz A, Ouckama RM, Slavic D. 2010. Arthritis and osteomyelitis associated with Enterococcus cecorum infection in broiler and broiler breeder chickens in Ontario, Canada. J Vet Diagn Invest. 22(4):643–645.
- Stojanov S, Kreft S. 2020. Gut Microbiota and the metabolism of phytoestrogens. Rev Bras Farmacogn. 30(2):145–154.
- Su Y, Tian Y, Wang T. 2014. Zhuanghe Dagu chicken breeding technology. Beijing: China Agriculture Press.
- Tan D, Ertunc ME, Konduri S, Zhang J, Pinto AM, Chu Q, Kahn BB, Siegel D, Saghatelian A. 2019. Discovery of FAHFA-containing triacylglycerols and their metabolic regulation. J Am Chem Soc. 141(22):8798–8806.
- Tejeda OJ, Kim KW. 2021. Role of dietary fiber in poultry nutrition. Animals. 11:461.
- Tucak M, Horvat D, Cupic T, Krizmanic G, Tomas V, Ravlic M, Popovic S. 2018. Forage legumes as sources of bioactive phytoestrogens for use in pharmaceutics: a review. Curr Pharm Biotechnol. 19(7):537–544.
- Videnska P, Faldynova M, Juricova H, Babak V, Sisak F, Havlickova H, Rychlik I. 2013. Chicken faecal microbiota and disturbances induced by single or repeated therapy with tetracycline and streptomycin. BMC Vet Res. 9:30–39.
- Waite DW, Taylor MW. 2014. Characterizing the avian gut microbiota: membership, driving influences, and potential function. Front Microbiol. 5:223.
- Wang W, Cao J, Yang F, Wang X, Zheng S, Sharshov K, Li L. 2016. High-throughput sequencing reveals the core gut microbiome of bar-headed goose (Anser indicus) in different wintering areas in Tibet. Microbiologyopen. 5(2):287–295.
- Wang XL, Zheng JX, Ning ZH, Qu LJ, Xu GY, Yang N. 2009. Laying performance and egg quality of blue-shelled layers as affected by different housing systems. Poultr Sci. 88(7):1485–1492.
- Want EJ, O'Maille G, Smith CA, Brandon TR, Uritboonthai W, Qin C, Trauger SA, Siuzdak G. 2006. Solvent-dependent metabolite distribution, clustering, and protein extraction for serum profiling with mass spectrometry. Anal Chem. 78(3):743–752.
- Wideman Jr RF. 2016. Bacterial chondronecrosis with osteomyelitis and lameness in broilers: a review. Poult Sci. 95(2):325–344.
- Wu F, Guo X, Zhang J, Zhang M, Ou Z, Peng Y. 2017. Phascolarctobacteriumáfaecium abundant colonization in human gastrointestinal tract. Experimental and Therapeutic Medicine. 14(4):3122–3126.
- Xiang Q, Wang C, Zhang H, Lai W, Wei H, Peng J. 2019. Effects of different probiotics on laying performance, egg quality, oxidative status, and gut health in laying hens. Animals. 9(12):1110.
- Yang JX, Chaudhry MT, Yao JY, Wang SN, Zhou B, Wang M, Han CY, You Y, Li Y. 2018. Effects of phyto‐oestrogen quercetin on productive performance, hormones, reproductive organs and apoptotic genes in laying hens. J Anim Physiol Anim Nutr. 102(2):505–513.
- Yıldız AÖ, Şentürk ET, Olgun O. 2020. Use of alfalfa meal in layer diets–a review. World Poultr Sci J. 76(1):134–143.
- Yore MM, Syed I, Moraes-Vieira PM, Zhang T, Herman MA, Homan EA, Patel RT, Lee J, Chen S, Peroni OD, et al. 2014. Discovery of a class of endogenous mammalian lipids with anti-diabetic and anti-inflammatory effects. Cell. 159(2):318–332.
- Yoshioka Y, Yamashita Y, Kishida H, Nakagawa K, Ashida H. 2018. Licorice flavonoid oil enhances muscle mass in KK-Ay mice. Life Sci. 205:91–96.
- Zheng M, Mao P, Tian X, Guo Q, Meng L. 2019. Effects of dietary supplementation of alfalfa meal on growth performance, carcass characteristics, meat and egg quality, and intestinal microbiota in Beijing-you chicken. Poultr Sci. 98(5):2250–2259.