Abstract
To evaluate the effects of heat stress on lipid metabolism in the liver and abdominal adipose deposition in broilers, totally, 120 female 21-day old broilers were selected and randomly allocated to 2 groups with 6 replication and 10 broilers per each replication. Broilers in the control group (CON) were reared at 24 °C, and broilers in the heat stress group (HS) were reared at 34 °C. Heat stress exposure increased (P < 0.05) rectal temperature, relative weight of abdominal adipose and liver, serum triacylglycerol (TG) and total cholesterol (TC) level, liver TG, TC, and very low-density lipoprotein (VLDL) content. Hepatic mRNA expression level of fatty acid synthase (FAS), acetyl-coenzyme A carboxylase (ACC), and abdominal adipose mRNA expression level of ACC and CCAAT/enhancer-binding protein-α (C/EBPα) were up-regulated (P < 0.05) when compared with the CON group. Heat stress exposure increased serum and hepatic TC and TG content, up-regulated the mRNA expression of FAS and ACC in liver, and promoted preadipocytes differentiation by increasing C/EBPα mRNA expression in abdominal adipose. Therefore, heat stress exposure increased abdominal adipose deposition, which related to increase in hepatic de novo lipogenesis, and promoted preadipocytes differentiation in abdominal adipose.
Heat stress increased abdominal adipose deposition
Heat stress increased hepatic de novo lipogenesis
Heat stress promoted preadipocytes differentiation in abdominal adipose
HIGHLIGHTS
Introduction
Heat stress was one of the main environmental stressors in poultry industry. In modern poultry industry, the genetic selection and commercial production for fast growth rate and feed efficiency, making broilers more sensitive to high temperature (Attia et al. Citation2017; Attia and Hassan Citation2017; Attia et al. Citation2018). Heat stress induced detrimental effects on feed intake and feed utilisation, disturbing intestinal and immune function, inducing endocrine disorder and oxidative damage, leading to poor growth performance (Farag and Alagawany Citation2018; Gonzalez-Rivas et al. Citation2020; Wasti et al. Citation2020; Kikusato et al. Citation2021; Olugbenga et al. Citation2022; Park et al. Citation2022). Meanwhile, heat stress response also involved in lipid metabolism. The lipid content in the intramuscular, subcutaneous, and abdominal adipose tissue of broilers increased under heat stress condition (Lu et al. Citation2007; Zhang et al. Citation2012; De Antonio et al. Citation2017; Lu et al. Citation2019). The excessive fat deposition was a negative phenomenon for economic benefit in poultry industry due to reduce feed efficiency and carcase yield, affected consumers’ acceptance, enhanced the processing fee for adipose discarding, and even polluted the environment (Fouad and El-Senousey Citation2014; Han et al. Citation2016; Moreira et al. Citation2018; Liu et al. Citation2019).
Liver was the main site of de novo lipogenesis (DL) in broilers, and synthesised over 90% fatty acids, while adipose tissue served as a fat storage site (Laliotis et al. Citation2010). The synthesised fatty acids were incorporated into triacylglycerol (TG) and assembled into very-low density lipoprotein (VLDL), then ferry to other tissues for energy supply or storage (Emami et al. Citation2020). Meanwhile, VLDL played a critical role in TG transportation to extra-hepatic tissues. Thus, hepatic lipid metabolism was closely related to TG accumulation in abdominal fat. Therefore, the increased fat deposition of heat stress exposure broilers may result in increasing hepatic DL and TG exportation to extra-hepatic tissues. Former literatures indicated that liver was responsive and susceptible to heat stress and changed the hepatic lipid metabolism (Faylon et al. Citation2015; Tang et al. Citation2015; Flees et al. Citation2017; Jastrebski et al. Citation2017). Meanwhile, literatures also illustrated that heat stress increased the level of TG and VLDL in serum and liver, as well as mRNA expression level of fatty acid synthase related genes (Lu et al. Citation2019). Heat stress increased fat deposition in broilers, but the relationship between heat stress and lipid metabolism in the liver and abdominal adipose tissue were poorly understood. Therefore, we hypothesised that heat stress increased abdominal adipose deposition by up-regulating hepatic DL in broilers. The aim of this study was to evaluate the effects of heat stress on lipid metabolism in the liver and abdominal adipose deposition in broilers.
Materials and methods
Animals and diets
A total of 120 one-day old female Arbour broilers were provided by a local commercial hatchery and the diet was formulated to meet or exceed the nutritional requirements of broilers during starter (day 1–21) and grower (day 22–42) phases, according to the NRC (1994) recommendations (Table ). The temperature was maintained at 33 ± 1 °C for the first week. From day 8, the temperature was gradually reduced by 0.5 °C per day until maintained at 24 °C. Artificial light was provided 23 h/d by fluorescent lights. On day 21, 120 broilers were randomly selected and allocated to 2 groups with 6 replication and 10 broilers of each replication. Broilers in the control group (CON) reared at 24 °C, and broilers in the heat stress group (HS) reared at 34 °C, all broilers were ad libitum accessed to water and commercial feed diet. On day 1, 7, 14, and 21, two broilers were randomly selected from each pen to measure the rectal temperature at 9:00.
Table 1. Ingredient composition and nutrient content of diets.
Growth performance
On day 21 and 42, body weight and feed intake were recorded as pen-basis to calculate average daily gain (ADG), average daily feed intake (ADFI), and feed conversion ratio (FCR).
Sample collection
On day 42, one broiler was randomly selected from each pen. After body weight check, blood samples were collected from the wing vein, and serum were separated for later analysis of TG, total cholesterol (TC), VLDL, low density lipoprotein (LDL), high density lipoprotein (HDL). Then, broilers were sacrificed, and the abdominal adipose and liver were isolated and weighted to calculated the relative weight of abdominal adipose and liver. Finally, abdominal adipose and liver samples were collected, freeze in lipid nitrogen, and stored at −80 °C for later analysis of hepatic TC, TG, VLDL, LDL, and HDL content, as well as gene expression.
Blood and liver TC, TG, VLDL, LDL, and HDL assay
The content of TC (A111-1-1), TG (A110-1-1), VLDL (H249), LDL (A113-1-1), and HDL (A112-1-1) in serum and liver were measured using commercial kits (Nanjing Jiancheng Bioengineering Institute, Nanjing, China) followed the kits instruction.
Gene expression
Total RNA extraction, cDNA synthesis, and real-time quantitative polymerase chain reaction (RT-PCR) were followed the methods described by Lan et al. (Citation2014), and the relative gene expression was calculated using the 2-ΔΔCt method (Livak and Schmittgen Citation2001). The primer sequences were listed in Table .
Table 2. Primers for real-time PCR.
Table 3. Effects of heat stress exposure on growth performance of broilers.
Statistical analysis
The pen as the experimental unit, all data were analysed using the general linear model (GLM) procedure of the SAS 2003 (SAS Institute Inc., Cary, NC). The statistical differences between the CON and HS group were using Student’s t-test for unpaired data. The results presented as mean ± standard error, and P < 0.05 indicated significant difference.
Results
Rectal temperature
As shown in Figure , after 1-day, 7-day, 14-day and 21-day heat stress exposure, the rectal temperature in the HS group was higher (P < 0.05) than that in the CON group.
Growth performance
As shown in Table , heat stress exposure decreased (P < 0.05) the final body weight, ADG and ADFI when compared to the CON group, while increased (P < 0.05) the FCR.
Relative weight of abdominal adipose and liver
As shown in Figure , heat stress increased (P < 0.05) the relative weight of abdominal adipose and liver when compared to the CON group.
Lipid metabolism parameters in serum and liver
As shown in Figure , heat stress increased (P < 0.05) the content of TC and TG in the serum, but had no significant effects on VLDL, LDL, or HDL content. In addition, heat stress increased (P < 0.05) the content of TC, TG, and VLDL in the liver, but had no significant effects on LDL or HDL content (Figure ).
Figure 3. Effects of heat stress exposure on the lipid metabolism parameters in serum of broilers. CON, control group; HS, heat stress exposure; TC, total cholesterol; TG, triglyceride; VLDL, very low-density lipoprotein; LDL, low-density lipoprotein; HDL, high-density lipoprotein. The pound key (#) indicated significant difference (P < 0.05).
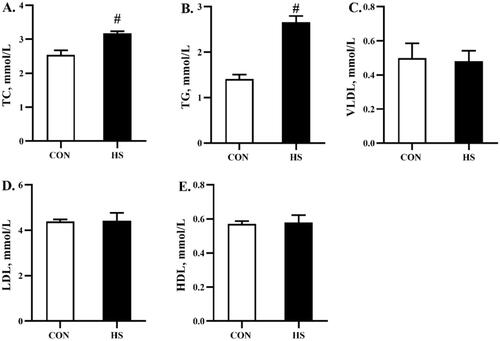
Figure 4. Effects of heat stress exposure on the lipid metabolism parameters in liver of broilers. CON, control group; HS, heat stress exposure; TC, total cholesterol; TG, triglyceride; VLDL, very low-density lipoprotein; LDL, low-density lipoprotein; HDL, high-density lipoprotein. The pound key (#) indicated significant difference (P < 0.05).
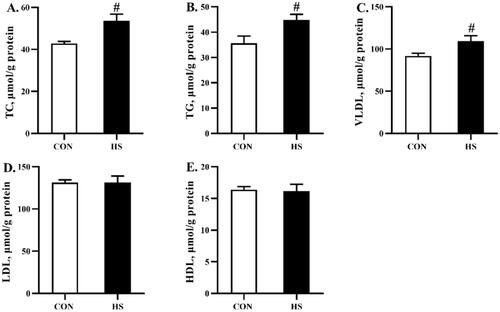
Gene expression related to lipid metabolism
In the liver, heat stress increased (P < 0.05) the mRNA expression level of FAS and ACC which were related to lipogenesis (Figure ), while the mRNA expression level of PPARα, MTTP, LPL, and CPT1 which were related to lipolysis were not significantly affected (Figure ).
Figure 5. Effects of heat stress exposure on gene expression related to lipid metabolism in the liver and abdominal adipose of broilers. CON, control group; HS, heat stress exposure; ChREBP, carbohydrate response element-binding protein; SREBP-1c, sterol regulatory element–binding transcription factor-1c; FAS, fatty acid synthase; ACC, acetyl-coenzyme A carboxylase; PPAR, peroxisome proliferator–activated receptor; MTTP, microsomal triglyceride transfer protein; LPL, lipoprotein lipase; CPT1, carnitine palmitoyl transferase 1; C/EBP, CCAAT/enhancer-bindingprotein. The pound key (#) indicated significant difference (P < 0.05).
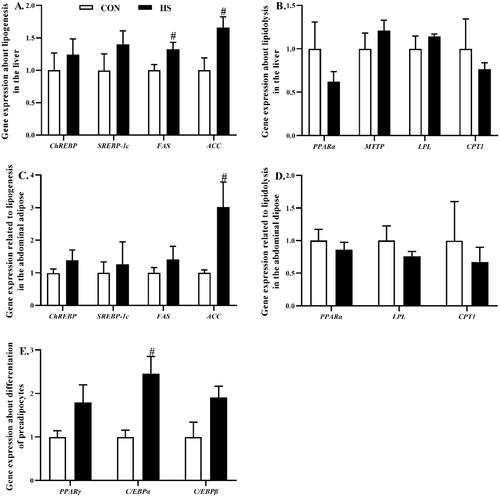
In the abdominal adipose, heat stress only increased (P < 0.05) the mRNA expression level of ACC (Figure ), while the lipolysis related genes PPARα, LPL, and CPT1 were not significantly affected (Figure ). Moreover, we also detected the mRNA expression level of PPARγ, C/EBPα, and C/EBPβ in the abdominal adipose, which could affect preadipocytes differentiation. Heat stress increased (P < 0.05) the mRNA expression level of C/EBPα (Figure ).
Discussion
The rectal temperature was one of the indicators of heat stress, which reflected the body temperature. In broilers, the rectal temperature reflected the balance between heat gain and loss (Farag and Alagawany Citation2018). In this study, the rectal temperature of heat stress exposure broilers was higher on day 1, 7, 14, and 21, which suggested that physiological response and heat stress model established (Zhang et al. Citation2018; He et al. Citation2019).
Heat stress induced a series of deleterious impacts on physiology and immunology of poultry, including decreased feed intake and feed utilisation, disturbing intestinal and immune function, inducing endocrine disorder and oxidative damage (Farag and Alagawany Citation2018; Gonzalez-Rivas et al. Citation2020; Wasti et al. Citation2020; Kikusato et al. Citation2021), which resulted in poor productivity. As expected, heat stress exposure decreased ADG and ADFI, while increased FCR. These results were in consistent with the reports by Cheng et al. (Citation2019), who reported that heat stress exposure decreased ADG and ADFI, as well as increased FCR. Heat stress exposure decreased ADG may related to the lower feed intake and feed utilisation (Habashy et al. Citation2017a, Citation2017b; Chang et al. Citation2020). Moreover, to adapt the high temperature, heat-stressed broilers might consume more energy to maintain homeostasis, thereby reducing energy supply for growth and resulting in poor growth performance (Nawab et al. Citation2018).
Previous studies indicated that heat stress exposure increased liver fat synthesis and abdominal adipose deposition in broilers (Lu et al. Citation2007; Flees et al. Citation2017; Lu et al. Citation2019; Yin et al. Citation2021). In this study, heat exposure increased the relative weight of liver and abdominal adipose. Abdominal adipose deposition mainly depended on the hepatic DL and plasma TG absorption (Hermier Citation1997). TG was synthesised in the liver and VLDL played a vital role in exportation TG to extra-hepatic tissue, which suggested that abdominal adipose deposition was related to the levels of TG and VLDL in plasma (Cryer Citation1981; Griffin et al. Citation1991). In this study, heat exposure increased serum TC and TG, suggested that heat stress exposure increased abdominal adipose deposition might be due to the increased TG exportation from the liver, as well as enhanced fatty acid uptake in abdominal adipose. These results were consistent with the results of He et al. (Citation2015) and Lu et al. (Citation2019). Moreover, abdominal adipose development included the preadipocytes hyperplasia (enhanced in number) and hypertrophy (enhanced in volume) (Guo et al. Citation2011). PPARγ, C/EBPα, and C/EBPβ were reported as the key regulators in preadipocytes differentiation, and played vital role in affecting abdominal adipose deposition (Farmer Citation2006; Xu et al. Citation2021). Higher C/EBPα mRNA expression level in the HS group suggested that abdominal adipose deposition was promoted in some degree. ACC mRNA expression was up-regulated in abdominal adipose by heat stress exposure among all detected genes (ChREBP, SREBP-1c, FAS, and ACC) associated with lipogenesis, while the genes (PPARα, LPL, and CPT1) associated with lipolysis was not significant affected. Broilers DL mainly occurred in the liver, but less in abdominal adipose (O'hea and Leveille Citation1968), thus we speculated that heat stress exposure promoted adipocytes proliferation might comprehensively demonstrated the possible reason for increasing abdominal adipose deposition.
Hepatic TG accumulation was the balance among plasma non-esterified fatty acid absorption, DL, fatty acid oxidation and the secretion of VLDL-TG (Hodson and Gunn Citation2019). Fatty acid synthesised in the liver was incorporated into TG and packed into VLDL, which ferry to hepatic-extra tissues for storage or energy supply (Emami et al. Citation2020). In this study, heat stress exposure enhanced liver TC, TG, and VLDL level, indicating enhanced fat synthesis and TG exportation, which was in line with higher serum TC and TG level. Liver VLDL assembly and subsequent secretion from hepatocytes was depended on MTTP (Liu et al. Citation2016, Citation2019). In this study, the MTTP mRNA expression was not influenced by heat stress exposure, suggesting that heat stress exposure may limit TG export from liver. Thus, the threshold of MTTP might be the main factor for lipid accumulation in the liver of broilers exposed to heat stress, the results consistent with the higher weight of liver in broilers.
Conclusions
In conclusion, heat stress (34 °C) exposure increased abdominal adipose deposition, serum TC and TG level, the mRNA expression of FAS and ACC, promoted preadipocytes differentiation by increasing C/EBPα mRNA expression in abdominal adipose of broilers. Therefore, heat stress exposure increased abdominal adipose deposition, which related to the increasing in hepatic DL, and promoted preadipocytes differentiation in abdominal adipose.
Ethical approval
The experimental protocol used in this study was approved by the Animal Care and Use Committee of Guangdong Ocean University (SYXK-2018-0147).
Disclosure statement
No conflict of interest was reported by the authors.
Data availability statement
The original data of the paper are available upon request from the corresponding author.
Additional information
Funding
References
- Attia YA, Al-Harthi MA, El-Shafey AS, Rehab YA, Kim WK. 2017. Enhancing tolerance of broiler chickens to heat stress by supplementation with vitamin E, vitamin C and/or probiotics. Ann Anim Sci. 17(4):1155–1169.
- Attia YA, Al-Harthi MA, Sh. Elnaggar A. 2018. Productive, physiological and immunological responses of two broiler strains fed different dietary regimens and exposed to heat stress. Ital J Anim Sci. 17(3):686–697.
- Attia YA, Hassan SS. 2017. Broiler tolerance to heat stress at various dietary protein/energy levels. Europ Poult Sci. 81:1–15.
- Chang Q, Lu Y, Lan R. 2020. Chitosan oligosaccharide as an effective feed additive to maintain growth performance, meat quality, muscle glycolytic metabolism, and oxidative status in yellow-feather broilers under heat stress. Poult Sci. 99(10):4824–4831.
- Cheng Y, Chen Y, Chen R, Su Y, Zhang R, He Q, Wang K, Wen C, Zhou Y. 2019. Dietary mannan oligosaccharide ameliorates cyclic heat stress-induced damages on intestinal oxidative status and barrier integrity of broilers. Poult. Sci. 98(10):4767–4776.
- Cryer A. 1981. Tissue lipoprotein lipase activity and its action in lipoprotein metabolism. Int J Biochem. 13(5):525–541.
- De Antonio J, Fernandez-Alarcon M, Lunedo R, Squassoni G, Ferraz A, Macari M, Furlan R, Furlan L. 2017. Chronic heat stress and feed restriction affects carcass composition and the expression of genes involved in the control of fat deposition in broilers. J Agric Sci. 155(9):1487–1496.
- Emami NK, Jung U, Voy B, Dridi S. 2020. Radical response: effects of heat stress-induced oxidative stress on lipid metabolism in the avian liver. Antioxidants. 10(1):35.
- Farag MR, Alagawany M. 2018. Physiological alterations of poultry to the high environmental temperature. J Therm Biol. 76:101–106.
- Farmer SR. 2006. Transcriptional control of adipocyte formation. Cell Metab. 4(4):263–273.
- Faylon M, Baumgard L, Rhoads R, Spurlock D. 2015. Effects of acute heat stress on lipid metabolism of bovine primary adipocytes. J Dairy Sci. 98(12):8732–8740.
- Flees J, Rajaei-Sharifabadi H, Greene E, Beer L, Hargis BM, Ellestad L, Porter T, Donoghue A, Bottje WG, Dridi S. 2017. Effect of Morinda citrifolia (noni)-enriched diet on hepatic heat shock protein and lipid metabolism-related genes in heat stressed broiler chickens. Front Physiol. 8:919.
- Fouad A, El-Senousey H. 2014. Nutritional factors affecting abdominal fat deposition in poultry: a review. Asian-Australas J Anim Sci. 27(7):1057–1068.
- Gonzalez-Rivas PA, Chauhan SS, Ha M, Fegan N, Dunshea FR, Warner RD. 2020. Effects of heat stress on animal physiology, metabolism, and meat quality: a review. Meat Sci. 162:108025.
- Griffin H, Windsor D, Whitehead C. 1991. Changes in lipoprotein metabolism and body composition in chickens in response to divergent selection for plasma very low density lipoprotein concentration. Br Poult Sci. 32(1):195–201.
- Guo L, Sun B, Shang Z, Leng L, Wang Y, Wang N, Li H. 2011. Comparison of adipose tissue cellularity in chicken lines divergently selected for fatness. Poult Sci. 90(9):2024–2034.
- Habashy W, Milfort M, Adomako K, Attia Y, Rekaya R, Aggrey S. 2017a. Effect of heat stress on amino acid digestibility and transporters in meat-type chickens. Poult Sci. 96(7):2312–2319.
- Habashy WS, Milfort MC, Fuller AL, Attia YA, Rekaya R, Aggrey SE. 2017b. Effect of heat stress on protein utilization and nutrient transporters in meat-type chickens. Int J Biometeorol. 61(12):2111–2118.
- Han J, Li L, Wang D, Ma H. 2016. Hydroxycitric acid reduced fat deposition via regulating lipid metabolism-related gene expression in broiler chickens. Lipids Health Dis. 15:1–13.
- He S, Li S, Arowolo MA, Yu Q, Chen F, Hu R, He J. 2019. Effect of resveratrol on growth performance, rectal temperature and serum parameters of yellow‐feather broilers under heat stress. Anim Sci J. 90(3):401–411.
- He S, Zhao S, Dai S, Liu D, Bokhari SG. 2015. Effects of dietary betaine on growth performance, fat deposition and serum lipids in broilers subjected to chronic heat stress. Anim Sci J. 86(10):897–903.
- Hermier D. 1997. Lipoprotein metabolism and fattening in poultry. J Nutr. 127(5 Suppl):805S–808S.
- Hodson L, Gunn PJ. 2019. The regulation of hepatic fatty acid synthesis and partitioning: the effect of nutritional state. Nat Rev Endocrinol. 15(12):689–700.
- Jastrebski SF, Lamont SJ, Schmidt CJ. 2017. Chicken hepatic response to chronic heat stress using integrated transcriptome and metabolome analysis. PLoS One. 12(7):e0181900.
- Kikusato M, Xue G, Pastor A, Niewold TA, Toyomizu M. 2021. Effects of plant-derived isoquinoline alkaloids on growth performance and intestinal function of broiler chickens under heat stress. Poult Sci. 100(2):957–963.
- Laliotis G, Bizelis I, Rogdakis E. 2010. Comparative approach of the de novo fatty acid synthesis (lipogenesis) between ruminant and non ruminant mammalian species: from biochemical level to the main regulatory lipogenic genes. Curr Genomics. 11(3):168–183.
- Lan R, Liu F, He Z, Chen C, Liu S, Shi Y, Liu Y, Yoshimura Y, Zhang M. 2014. Immunolocalization of GnRHRI, gonadotropin receptors, PGR, and PGRMCI during follicular development in the rabbit ovary. Theriogenology. 81(8):1139–1147.
- Liu Z, Li Q, Liu R, Zhao G, Zhang Y, Zheng M, Cui H, Li P, Cui X, Liu J, et al. 2016. Expression and methylation of microsomal triglyceride transfer protein and acetyl-CoA carboxylase are associated with fatty liver syndrome in chicken. Poult Sci. 95(6):1387–1395.
- Liu Y, Liu X, Zhou J, Ren Z, Yang X, Cao Y, Yang X. 2019. Folic acid perfusion administration reduced abdominal fat deposition in starter Arbor Acres broilers. Poult Sci. 98(12):6816–6825.
- Livak KJ, Schmittgen TD. 2001. Analysis of relative gene expression data using real-time quantitative PCR and the 2− ΔΔCT method. Methods. 25(4):402–408.
- Lu Z, He X, Ma B, Zhang L, Li J, Jiang Y, Zhou G, Gao F. 2019. Increased fat synthesis and limited apolipoprotein B cause lipid accumulation in the liver of broiler chickens exposed to chronic heat stress. Poult Sci. 98(9):3695–3704.
- Lu Q, Wen J, Zhang H. 2007. Effect of chronic heat exposure on fat deposition and meat quality in two genetic types of chicken. Poult Sci. 86(6):1059–1064.
- Moreira GCM, Boschiero C, Cesar ASM, Reecy JM, Godoy TF, Pértille F, Ledur MC, Moura A, Garrick DJ, Coutinho LL. 2018. Integration of genome wide association studies and whole genome sequencing provides novel insights into fat deposition in chicken. Sci Rep. 8:1–14.
- Nawab A, Ibtisham F, Li G, Kieser B, Wu J, Liu W, Zhao Y, Nawab Y, Li K, Xiao M, et al. 2018. Heat stress in poultry production: Mitigation strategies to overcome the future challenges facing the global poultry industry. J Therm Biol. 78:131–139.
- O'hea E, Leveille G. 1968. Lipogenesis in isolated adipose tissue of the domestic chick (Gallus domesticus). Comp Biochem Phys. 26(1):111–120.
- Olugbenga OO, Adebola SS, Friday AD, Mercy AT, Keniokpo OS. 2022. Effect of dietary tomato powder on growth performance and blood characteristics of heat-stressed broiler chickens. Trop Anim Health Prod. 54:1–7.
- Park JS, Kang DR, Shim KS. 2022. Proteomic changes in broiler liver by body weight differences under chronic heat stress. Poult Sci. 101(5):101794.
- Tang X, Meng Q, Gao J, Zhang S, Zhang H, Zhang M. 2015. Label-free quantitative analysis of changes in broiler liver proteins under heat stress using SWATH-MS technology. Sci Rep. 5:1–15.
- Wasti S, Sah N, Mishra B. 2020. Impact of heat stress on poultry health and performances, and potential mitigation strategies. Animals. 10(8):1266.
- Xu J, Strasburg GM, Reed KM, Velleman SG. 2021. Effect of temperature and selection for growth on intracellular lipid accumulation and adipogenic gene expression in turkey pectoralis major muscle satellite cells. Front Physiol. 12:667814.
- Yin C, Tang S, Liu L, Cao A, Xie J, Zhang H. 2021. Effects of bile acids on growth performance and lipid metabolism during chronic heat stress in broiler chickens. Animals. 11(3):630.
- Zhang J, Bai K, Su W, Wang A, Zhang L, Huang K, Wang T. 2018. Curcumin attenuates heat-stress-induced oxidant damage by simultaneous activation of GSH-related antioxidant enzymes and Nrf2-mediated phase II detoxifying enzyme systems in broiler chickens. Poult Sci. 97(4):1209–1219.
- Zhang Z, Jia G, Zuo J, Zhang Y, Lei J, Ren L, Feng D. 2012. Effects of constant and cyclic heat stress on muscle metabolism and meat quality of broiler breast fillet and thigh meat. Poult Sci. 91(11):2931–2937.