Abstract
The present study aimed to compare the nutrient composition, in vitro ruminal fermentation values and microbiome in the ruminal fermentation of herbage and silage of the Plantago media, P. major and P. lanceolata species. The lactic acid (LA) content of P. lanceolata silage was higher than those of other plantago silages (p < 0.05). The α-linolenic, w-3, polyunsaturated (PUFA), medium chain (MCFA) and long-chain fatty acids (LCFA) of plantago silages were lower than those of plantago herbages (p < 0.05). The neutral detergent fibre (NDF) and acid detergent fibre (ADF) contents, total gas and methane production, metabolic energy (ME) and organic matter digestion (OMD) values and ammonia-nitrogen concentration in the in vitro fermentation fluid of P. major silage were lower than those of other plantago silages (p < 0.05). The in vitro ruminal methane production and community of archaea Methanobrevibacter in the microbiome of P. major herbage were higher than that of P. media and P. lanceolata herbages. The ensiling process significantly increased the in vitro total gas production, acetic acid concentration and Prevotellaceae bacteria of P. media and P. lanceolate compared their herbages. As a result, P. lanceolata and P. media silages provided high-quality silage fermentation; the nutrient composition was not lost to a great extent in the silage environment and the ruminal fibrolytic bacterial composition was positively affected. Plantago major silage could not provide a good silage quality and the in vitro ruminal fermentation and ruminal fibrolytic bacteria community value were negatively affected.
The crude protein (CP) and very long chain fatty acids contents of P. major herbage were higher than those of P. media and P. lanceolata. However, the P. major silage had high silage CP and ammonia-nitrogen concentration, low in vitro ruminal digestion (total gas production, ME and OMD), and plant cell wall substances.
The ensiling of P. lanceolata and P. media positively affected the ruminal fibrolytic bacterial composition (Rikenellaceae_RC9_gut_group, Oscillospiraceae_UCG-002 and Prevotellaceae).
The in vitro ruminal methane production and community of Methanobrevibacter in the ruminal microbiome of P. major herbage were higher than those of P. media and P. lanceolata herbages.
Highlights
Introduction
The quality of forage, which is general expression of the levels of CP, structural carbohydrates, non-structural carbohydrates, inorganic matter and fatty acids and the fermentation capacity in gastro-intestinal cannula, changes according to the preservation form (herbage, hay, silage), climatic conditions (annual rainfall, temperature etc.) and soil characteristics (irrigation, pH, salty etc.) (Kara et al. Citation2018). Nowadays, the driest period of the last 900 years is experienced in the countries of the south of Europe and the Mediterranean region, where the effect of global drought is more pronounced (Ramirez-Restrepo and Barry Citation2005; IPCC Citation2014). Semi-arid areas are considered arid, while arid regions become desert (Altın et al. Citation2012). The green pastures in the arid and semi-arid areas present at a certain level in the spring and early summer months; after early summer, most plants turn yellow and dry out. In addition, global warming now encountered in the producing of high-quality forages, especially in arid and semi-arid areas (IPCC Citation2014). Researchers focus their studies on forage crops resistant to drought and stay green throughout the year (Pommerrenig et al. Citation2007; Rozentsvet et al. Citation2015; Kara et al. Citation2018; Minnée et al. Citation2020). Mudrik et al. (Citation2003) stated that the wide distribution of the plantago species demonstrated that these species effectively adapt to various environmental conditions, especially soil drought stress. These plants are also resistant to cold weather conditions and can continue to grow in winter. Plantago (250–300 species) belongs to the Plantaginaceae family and grows naturally in different parts of the world (Guil‐Guerrero Citation2001). The plant height varies according to the species, up to 60 cm and the leaf length (lancet, oval, elliptical or sliced according to the species) can reach up to approximately 25 cm. They are perennial and cosmopolitan plants that can grow in salty soils used for feeding (Samuelsen Citation2000). In the leaf parts of plantago species, biologically active glycosides (aucubin and catalpol), flavonoids, flavone glucosides, caffeic acid derivatives, some effective mucilage polysaccharides, monoterpenoids, vitamins and tannins have been found (Samuelsen Citation2000; Oprica et al. Citation2015). Plantago species’ resistance to plant diseases shows their superiority over other forage crops (Kara et al. Citation2016). Although it is a perennial plant, it can bloom in the first year. It usually blooms from April to August (Fons et al. Citation1998). Among these plants, the P. lanceolata (lanceolate plantain), P. major (broad-leaved plantain), and P. media species have the widest distribution (Bıçakçı et al. Citation2011). It has been reported that the leaves of plantago species were consumed voraciously by animals grazing in the pasture, as their flavour increases due to sorbitol, which accumulates at a high rate, especially in those grown in salty and arid soil conditions (Pommerrenig et al. Citation2007). Plantago species differs from each other in terms of their nutritional content. The leaf of P. lanceolata included about 15% crude protein (CP), 28%, neutral detergent fibre (NDF) and 50 mg/100g of total carotenoid (Guil‐Guerrero Citation2001). A study reported that P. lanceolata, harvested in spring, summer, and winter, varied between 71 and 80% in vitro DM digestibility, between 37 and 52% NDF value and between 11 and 19% CP values (Sanderson et al. Citation2003). The lanceot and tonic plantago species cultured from plantago species were on average 19–20% of CP values, 28–39% of NDF values and 84–86% of in vitro DM digestion (Labreveux Citation2002). Hay, vital important source of feedstuffs used in the ruminant ration, contains 1–3% fat and its fatty acid profile affects health and animal product quality (NRC Citation2001). It has been determined that the linolenic acid fatty acids content (C18:3), which has important biological activity, differ significantly between plantago species (45% for P. lanceolata, and 40% for P. major in the total fatty acid profile) (Guil‐Guerrero Citation2001). One of the nutritional ingredients of the plantago species that may affect its biological activity may be its fatty acid composition.
It is understood that it is important to determine the plantago species with the best feed quality, silage quality and effect on the rumen microflora when the effect of drought is evident as globally. The present study aims to compare the nutrient composition, in vitro ruminal fermentation values, and rumen microbiome of herbage and silage of P. media, P. major, and P. lanceolata species. The study hypothesises that the effects of plantago herbage and silage on nutrient composition, silage fermentation, in vitro ruminal fermentation, and microbiome will be variable between species, and the superior species will be determined.
Material and methods
Plantago herbages and silages
The herbage samples of P. lanceolata, P. major, and P. media were collected from Kayseri province, Türkiye (38°56 N, 34° 24 E). The samples of plantago herbage were collected from the same field (approximately 3000 m2) in June 2021 (at the beginning of the summer season). The samples of plantago herbage were collected from six different points of the same field using the random sampling method (Kara et al. Citation2018). The samples (total of 30 kg) were collected from over 1 cm on top of the land. The chopped forage was ensiled within one hour of harvesting time. The plantago species were harvested at the early flowering stage, and included all aerial parts (leaf, stem, or bud flower). For each plantago species, the silage process was done in eight replicates. The P. lanceolata, P. major, and P. media, were ensilaged in a polyethylene (25 cm × 35 cm sizes) silage bag. Each silage bag contained approximately 600 g of silage material. The cutting length of plantago herbage was approximately 2–2.5 cm length. These polyethylene bags were vacuumed using a vacuum machine (Caso VC100, Germany). The silage bags were stored in laboratory conditions in a sun-free environment for 60 days, and, then, over time, all bags opened. All silage bags were analysed in triplicate for chemical composition values.
Chemical compositions in herbage and silages
The silage materials and herbage were dried in a thermostatically controlled cabinet (Lovidond, Switzerland) for 48 hours at 60 °C, and dry matter (DM) content was calculated. Dried samples were mill in a grinder milled (IKA Werke, Germany) to a maximum of 1 mm particle size. The samples were analysed for the determination of DM, ash, crude protein (CP; nitrogen x 6.25), diethyl ether extract (EE) (AOAC Citation1995), neutral detergent fibre (NDF) and acid detergent fibre (ADF) (Van Soest et al. Citation1991). The EE contents of herbage and silage were methylated with the three-stage modified procedure of Wang et al. (Citation2015) in triplicate. A column of the fatty acid methyl esters (FAME’s), used for the separation of fatty acid (cyanopropylphenyl-based phase, length: 60 m, internal diameter: 0.25 mm, film: 0.25 µm and maximum temperature 250–260 °C; Thermo Scientific TRACE™, TR-FAME GC Columns, catalogue number: 260M153P, USA). The FAME’s in n-hexane were taken in a 1.5 ml screw neck ND-9 amber vial with 9 mm screw caps (silicone white/PTFE caps). The percentages of FAME’s were detected in a gas chromatograph with flame-ionisation detection (GC-FID, Thermo Scientific, USA). The peak identification of each FAME was detected in a commercial FAME’s mix standard solution in dichloromethane (Chem-Lab, catalogue number: CL.40.13093.0001, Zedelgem, Belgium). The FAME’s identification was performed by comparing the retention times with the expected retention times of the standard mixture in chromatograms (Kara Citation2020).
Acidity values and ammonia-nitrogen concentration in silages
After the silage bags were opened, the pH values of the materials were immediately determined. Firstly, 25 g of wet silage sample was shredded for 15 seconds with 100 mL of distilled water in a laboratory-type blender (Waring Commercial, Torrington, CT, USA). The pH value of the filtered silage material was measured with a digital pH metre (Mettler Toledo, S220 pH/ion metre, Ohio, USA). The lactic acid (LA) content in silage fluid was determined in a spectrophotometrically (SI Analytics – Xylem Analytics Germany Sales GmbH & Co. KG, Mainz, Germany) method (Tekin and Kara Citation2020), modified from Barnett (1951). The analysis of straight short-chain fatty acids (SCFA) [acetic acid – AA (C 2:0), propionic acid - PA (C 3:0), butyric acid - BA (C 4:0), valeric acid - VA (C 5:0), and caproic acid - CA (C 6:0)] and branched SCFAs (BSCFA) [iso-butyric acid - IBA (C 4:0i), iso-valeric acid - IVA (C 5:0i) and iso-caproic acid – ICA (C 6:0i)] in silage fluid was measured by using a gas chromatograph- flame ionisation detector (GC-FID) (TRACETM1300, Thermo Fisher Scientific, Orlando, USA) with polyethylene glycol column (length: 60 m, i.d: 0.25 mm, film thickness: 0.25 µm; TG-WAXMS, Thermo Scientific, Orlando, FL, USA) device according to the method of Ersahince and Kara (Citation2017). The ammonia-nitrogen concentration in silage fluid was determined using commercial ammonia assay procedure (Megazyme, K-AMIAR 02/20, Wicklow, Ireland).
The in vitro ruminal fermentation of forages
The in vitro ruminal fermentation values of plantago herbages (P. lanceolata herbage, P. major herbage, P. media herbage) and silages (P. lanceolata silage, P. major silage, P. media silage) were analysed using an in vitro gas production technique. Rumen fluid (approximately 1 L), used in the in vitro gas production technique, was taken from two Brown Swiss-Simmental mix breed cattle using a gastric tube. Rumen fluid was taken 3 hours after feeding. The cattle consumed the ration, which included maize silage (5 kg/day on as fed basis), wheat straw (1.7 kg/day on as fed basis), alfalfa herbage (1.5 kg/day on as fed basis), sugar beet pulp (4.5 kg/day on as fed basis) and concentrated mix feed (5 kg/day on as fed basis). Approximately one litter of rumen fluid was collected in a thermos, which included water, at 39 °C using CO2 gas, and filtered with six layers of cheesecloth in the laboratory. The samples were incubated in rumen fluid and buffer mix in 100 ml capacity calibrated anaerobic glass fermenter (Fortuna®, Poulten & Graf Ltd., Wertheim, Germany) following the procedures of Menke et al. (Citation1979). The reducing solution in the in vitro fermentation medium was added for providing the oxidation-reduction potential (ORP) and the anoxic conditions. The pH and ORP of the in vitro digestion fluids were measured using Mettler Toledo InLab® Expert Pro-ISM sensor probes in pH-Ion metre (Seven CompactTM pH/Ion S220, Mettler-Toledo, Schwerzenbach, Switzerland). The 200 ± 10 mg of dried samples (substrates) were incubated with 20 ml of buffer mixture and 10 ml of filtered rumen fluid in an anaerobic glass fermenter at 39.0 ± 0.5 °C in an incubator for 24 h, as five repetitions (Menke et al. Citation1979). Besides, five blank glass fermenters (no samples) were incubated to provide correction values. The total gas volume and produced substrates were read from the volume lines on the glass fermenter at 24 h. The amount of methane gas in total gas produced at 24 h was determined in an infra-red methane measurement device (Sensor, Europe GmbH, Erkrath, Germany) according to Kara et al. (Citation2015), in all anaerobic glass fermenter. The metabolic energy (ME) and organic matter digestion (OMD) values of the herbage and silages were calculated with total gas production and nutrient matter composition using equations by Menke and Steingass (Citation1988).
The SCFA and ammonia-nitrogen in the in vitro fermentation fluid
After in vitro incubation, SCFA and ammonia-nitrogen analyses of the in vitro fermentation fluid were carried out. The 2 mL of fermentation fluid was centrifuged at 15000 rpm x g for 15 min in a micro-centrifuge (Gyrozen 1524, Gyrozen Co. Ltd., Daejeon, Korea). The 1.25 mL of the supernatant and 0.25 mL of meta-phosphoric acid (25%, w/v) were mixed. Analysis of SCFA was determined by using a gas chromatograph (GC) device (Thermo Trace 1300, Thermo Scientific, USA) with an auto sampler (Thermo AI-1310, Thermo Scientific, USA). The GC device was equipped with a Flame Ionisation Detector (FID), with polyethylene glycol columns (length: 60 m, i.d: 0.25 mm × 0.25 µm, film thickness: 0.25 µm) (TG-WAXMS, Thermo Scientific, USA). The operation procedure of the GC-FID was according to the study of Ersahince and Kara (Citation2017). The ammonia-nitrogen content in fermentation fluid was determined using a commercial ammonia assay procedure (Megazyme, K-AMIAR 02/20, Wicklow, Ireland) on a spectrophotometer (Xylem Analytics Germany Sales GmbH & Co. KG, Mainz, Germany).
Microbiome analyses of the in vitro fermentation fluid
After in vitro incubation, microbiome analyses of the in vitro fermentation fluid were carried out. Total DNA of in vitro rumen fluid contents was extracted using the commercially kit (GeneMATRIX Tissue & Bacterial DNA Purification Kit, EURx Moleculer Biology Products, Przyrodników, Gdańsk, Poland). The concentration and quality of DNA were evaluated using a fluorometer (The VICTOR3™ Multilabel Plate Reader, Perkin Elmer, Shelton, CT, USA) using fluorescent-based double-chain Picogreen dye. The 16S rRNA (V3-V4) sequences were used to taxonomically identify bacterial and archaeal strains. This primer pair amplifies a region of approximately 460 bases. Before the next-generation sequencing readout, the sample’s 16S V3- V4 regions are amplified by PCR during amplicon library preparation. The V3–V4 region was amplified using the 16S Amplicon PCR Forward Primer = 5′ (TCGTCGGCAGCGTCAGATGTGTATAAGAGACAGCCTACGGGNGGCWGCAG) and the 16S Amplicon PCR Reverse Primer = 5′ (GTCTCGTGGGCTCGGAGATGTGTATAAGAGACAGGACTACHVGGGTATCTAATCC) primers (Klindworth et al. Citation2013).
The primers used for the gene-specific 16S rRNA V3-V4 region were taken from the Klindworth et al. (Citation2013). Metagenomic sequencing was performed on an Illumina Novaseq 6000 platform (Illumina, San Diego, CA, USA). Illumina’s ‘Sequencing by Synthesis’ technology detected each base as it was added to the DNA template during sequencing. Deoxyribonucleotide triphosphate (dNTP) mixes used in sequencing were special reversible, terminator-bound dNTPs. These dNTPs reduced the margin of error and the possibility of incorrect base addition due to their unique chemical structure in each sequencing cycle. The Illumina Novaseq instrument processes were read with the Novaseq Control Software program and bases were determined using the Real Time Analysis v1.18 program. Base reads were converted to FASTQ format with the bcl2fastq (v1.8.4) program. Scythe (v0.994 BETA) and Sickle programs extracted non-specific adapter sequences from the read results. With bioinformatics analysis, the taxonomic classification of the samples, alignment according to reference sequences, operational taxonomic unit determination, and grouping of species according to their similarities were performed.
Statistical analysis
Three different herbages and three silages were studied in five repetitions, and 30 anaerobic glass fermenters were used. For each plantago species, each silage bag was analysed as three replications (8 silage bags × 3 replications = a total of 24 replications). In vitro fermentation and end product analyses (total gas, methane, estimated fermentation values, SCFA, microbiome, etc.) were performed with five replications.
The experimental data were first subjected to Levene’s test to detect the variance homogeneity. Statistical significance among plantago silages for silage acidity and ammonia-nitrogen were determined by one-way variance analysis. The multivariate analyses were implemented for homogeneous variances by General Linear Model procedures to test treatment differences for nutrient, fatty acids, and in vitro ruminal fermentation values of plantago herbage and silages. The two-way variance analysis was conducted on silage chemical compositions and in vitro digestion values tested in different silages. Data (nutrient matter, fatty acids, and in vitro ruminal fermentation values) were analysed using the following statistical model: Yijk = µ + Ei + Dj + EDij + eijk. Where, Yijk – the overall mean for each parameter investigated; μ – over mean; E – effect of i: plantago species on the observed parameters; D – effect of j – forage type on the observed parameters; ED – interaction between the i – plantago species and j – forage type; eijk – the standard error term. Tukey post hoc test was used to determine the significant differences at p < 0.05.
Results
Chemical composition
The chemical compositions of plantago herbage and silages are shown in Table . The DM and ADF contents of plantago species’ herbages were similar (p > 0.05). The DM and ash contents of P. major silage were higher than those of P. media silage and P. lanceolata silage (p < 0.05). The NDF and ADF contents of P. major silage were lower than those of P. media silage and P. lanceolata silage (p < 0.05). The average DM, ash and EE contents of plantago silages were higher than those of plantago herbages (p < 0.05). The CP, NDF and ADF contents of plantago silages were lower than those of plantago herbages (p < 0.05).
Table 1. The chemical composition of plantago herbage and silages.
Silage ammonia-nitrogen and acidity values
The ammonia-nitrogen concentration and acidity values in silages of different plantago species are shown in Table . The ammonia-nitrogen concentration of P. major silage was higher than those of P. media and P. lanceolata silages (p < 0.05). The pH values in silage fluids of P. media and P. major were higher than that of P. lanceolata (p < 0.05). The LA content in silage of P. lanceolata was higher than those of P. major and P. media (p < 0.05). Besides, the BA, PA and IBA contents in silage of P. media were higher than those of P. major and P. lanceolata (p < 0.05).
Table 2. The ammonia-nitrogen concentration and acidity values in silages of different plantago species.
Fatty acid composition
The compositions of fatty acid in total fatty acids of plantago herbage and silages are shown in Table . The percentage of palmitic acid of P. media herbage was lower than those of P. major and P. lanceolata herbage (p < 0.05). The percentages of stearic acid, oleic acid, monounsaturated fatty acids (MUFA) and medium chain fatty acids (MCFA) of P. major herbage were lower than those of P. media and P. lanceolata herbages (p < 0.05). The percentages of linoleic acid and long chain fatty acids (LCFA) of P. lanceolata herbage were higher than those of P. media and P. major herbages (p < 0.05). The percentages of oleic, linoleic and w-9 fatty acids of P. lanceolata silage were lower than those of P. media and P. major silages (p < 0.05). The percentages of very long chain fatty acids (VLCFA) in total fatty acids of P. lanceolata and P. major silages were higher than that of P. media silage (p < 0.05). The percentages of oleic, w-6 and VLCFA fatty acids in total fatty acids of plantago silages (average value) were higher than those of plantago herbages (average value) (p < 0.05). The percentages of α-linolenic, PUFA, w-3, MCFA and LCFA fatty acids in total fatty acids of plantago silages (average value) were lower than those of plantago herbages (average value) (p < 0.05).
Table 3. The compositions of fatty acid in total fatty acids of plantago herbage and silages.
The in vitro ruminal fermentation and end-products
The in vitro ruminal fermentation values of plantago herbage and silages are in Table . The in vitro ruminal fermentation values of different plantago species ’herbages did not same (p < 0.05). The in vitro total gas production, in vitro methane production, ME and OMD values and ammonia-nitrogen concentration in rumen fluid of P. major silage were lower than those of P. media and P. lanceolata silages. The in vitro total gas production, ME and OMD values of plantago silages were higher than those of plantago herbage (p < 0.05).
Table 4. The in vitro ruminal fermentation values of plantago herbage and silages.
Short chain fatty acids (mmol/L) in the in vitro ruminal fermentation fluid of plantago herbage and silages are shown in Table . The concentrations of straight short-chain fatty acids (SCFA) and branched short-chain fatty acids (BSCFA) in the fermentation fluid of plantago herbages and silages did not differ (p > 0.05). Ensiling of plantago species increased the concentrations of AA, PA, VA, CA, iso-acids (IVA and IBA) and total short-chain fatty acids (tSCFA) in the in vitro ruminal fermentation fluid (p < 0.05).
Table 5. Short-chain fatty acids (mmol/L) in the in vitro ruminal fermentation fluid of plantago herbage and silages.
Microbiome analyses
The relative abundance of the bacteria kingdom of the in vitro fermentation fluid microbiome differed for different plantago forages and ranged from 89.40 to 93.25% (p < 0.05). The archaea community of P. major herbage (9.71%) and P. major silage (7.09%) in the microbiome were higher than those of other forages (p < 0.05).
The most relative abundant bacteria phylums in the in vitro rumen fermentation fluid’ microbiome of plantago forages were Bacteriodota (38.39–32.21%), Firmicutes (41.69–28.11%), Planctomycetota (5.62–2.61%), Fusobacteriota (9.22–0.13%), Spirochaetota (4.36–1.92%), Proteobacteriota (4.13–1.71%), Verrucomicrobiota (4.77–1.20%), Patescibacteria (2.00–1.22%), Desulfobacterota (1.33–0.95%) and Actinobacteriota (2.41–0.30%), respectively (Figure ).
Relative abundance of the dominant genus with an average relative abundance ≥0.5%
At genus level of bacteria, the Rikenellaceae_RC9_gut_group (18.50–15.51%) Prevotella (9.82–4.06%), Solibacillus (8.36–0.5%), Fusobacterium (9.18–0.44%), the Bacteroideales F082 group (3.82–2.90%), Oscillospiraceae_NK4A214_group (3.89–1.98%), Christensenellaceae R-7 group (2.27–1.98%), Pirellula (2.53–1.21%), Treponema (2.66–0.70%), Muribaculaceae (2.64–1.03%), Lachnospiraceae (1.80–0.77%), the Eubacterium_coprostanoligenes group (2.08–1.01%), Lysinibacillus (4.22–0.15%), the Lachnospiraceae NK3A20 group (2.07–0.80%), Oscillospiraceae UCG-002 (1.81–0.87%), Butyrivibrio (2.07–0.59%), Prevotellaceae UCG-001 (1.54–0.99%), the Sphaerochaeta (1.48–0.78%), Desulfovibrio (1.18–0.87%) were the most abundant species in microbiome of the in vitro fermentation fluid of plantago forages (Figure ).
Figure 2. Bacteria and archaea genus in microbiome of the in vitro ruminal fermentation fluid of plantago forages.
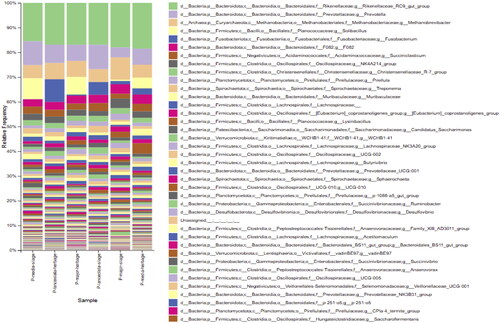
The relative abundance of genus Sphaerochaeta and Treponema in the Spirochaetaceae family in the rumen fluid of plantago silages were higher than those of plantago herbages (p < 0.05). Relative abundance of Lachnospiraceae_NK3A20_group (cellulolytic bacteria) in rumen fluid of P. major silage and P. major herbage were higher than those of P. media and P. lanceolata forage (p < 0.05). The relative abundance of Oscillospiraceae_UCG-002 in rumen fluid of plantago silages was higher than those of plantago herbages (p < 0.05). Prevotellaceae UCG-001 were relatively more abundant in P. media silage than in other plantago forages (p < 0.05).
Discussion
Nutrient content
The DM content of the plantago species (P. media, P. lanceolata and P. major) used in the present study at the time of harvesting was higher than the stated results (14.2, 13.4, and 12.3%, respectively) for these species in the previous research (Guil‐Guerrero Citation2001). Besides, the DM (29%) and ash (15%) values reported by another researcher (Alghamdi Citation2018) for P. lanceolata herbage, the arid zones with a short scattered rainy season and prolonged dry period, were higher than that of the present study. Labreveux (Citation2002) stated that the cultured lanceot and tonic plantago species included an average of 19–20% of CP and 28–39% of NDF from spring to late summer. The ash, CP, NDF and ADF contents of P. lanceolata herbage in the present study were similar to the result of the previous studies (Sanderson et al. Citation2003; Kara et al. Citation2018; Alghamdi Citation2018; Bariroh et al. Citation2021). Although it was determined that the CP content of P. major herbage was higher than those of P. media and P. lanceolata herbages in the present study, in the previous survey (Alghamdi Citation2018), it was reported that CP content of P. media was higher than those of P. lanceolata and P major herbage. In the present study, decreased CP and increased DM contents of P. media and P. lanceolata herbages with the ensilaging process were similar to the previous survey of Bariroh et al. (Citation2021). Significant differences in nutrient compositions (particularly fatty acid profile) of different plantago species to the present study are similar in the previous studies (Sanderson et al. Citation2003; Kara et al. Citation2018; Alghamdi Citation2018; Bariroh et al. Citation2021).
In the present study, there are differences between the nutrient composition results of plantago species. The results of previous studies may depend on the cultivation status or collection from natural pasture, climatic conditions, vegetation period, and soil type. Anaerobic fermentation in the silage medium causes the bacterial breakdown of easily digestible carbohydrates, causing silage gas formation increased silage acidity. In the present study, the decrease in CP content with the ensilaging of P. major showed that protein compounds were broken down in the silage enviroment. Decreasing CP content in forage material was undesirable for ruminant feeding and forage quality. However, the fact that the CP value did not change in P. lanceolata and P. media silages stands out as an important advantage compared to P. major. The significant decrease in the NDF value of P. major herbage, which was already lower than the other herbage, with silage production indicates that this material had a high fibre digestibility in the silage medium. The structural carbohydrate components of P. major suffered a significant loss after ensilaging. Considering these results, silage of P. major was a disadvantage for P. media and P. lanceolata silages in terms of loss of CP and structural carbohydrates.
In the present study, the major fatty acids in total fatty acids of plantago herbages can be depicted in a series based on their content in the following order: С18:3>С16:0>С18:2>С18:1>С18:0 > C16:0. The palmitic, stearic, oleic and linoleic acids were fatty acids that differed between P. media, P. major and P. lanceolata herbages. Rozentsvet et al. (Citation2015) demonstrated that the C16 plus C18 fatty acids content of P. media leaves comprised more than 90% of total fatty acids. The main fatty acid component of P. media herbage was fatty acids with chains containing from 14 to 20 carbon atoms. It included about 2% with hydrocarbon chains longer than 20 carbon atoms in total fatty acids (Rozentsvet et al. Citation2015). The main component of unsaturated fatty of plantago species in the present study was linolenic acid (С18:3; 47.8–50.4% for herbage and 37.4–40.6% for silages), and their predominant saturated fatty acid was palmitic acid (C16:0; 16.8–18.4% for herbage and 23.1–24.5% for silages). Generally, the percentages of unsaturated fatty acids in plantago forages were similar to those of P. media leaves (major unsaturated fatty acids; C18:3 was 33–58% of total fatty acids) by previous researchers (Rozentsvet et al. Citation2015). Although lower than the results of the present study, Guil‐Guerrero (Citation2001) determined that the main fatty acids in leaves for all plantago species was the α-linolenic acid, which was 38.1% for P. media leaves, 40.0% for P. major leaves and 45.1% P. lanceolata leaves. Presently, the differences in fatty acid composition for plantago species may be related to the leaf: stem ratio, leaf width and environmental conditions. Plantago’s fermentation in the silage environment caused a change in the fatty acid concentrations (especially C16:0, C18:0, C18:1, C18:2, and C18:3); the effect also differed between species. The effect of oxidation and differentiation on fatty acids composition in a silage environment has been explored recently (Han and Zhou Citation2013; Kara Citation2021). Increased oxidation in the silage environment changes the fatty acid profile of forage materials (Kara Citation2021). In the present study, the α-linolenic acid % in total fatty acids of plantago species diminished (about 49 vs. 39% in total fatty acids) with the ensiling process was similar to results, for Melilotus officinalis silages (Kara Citation2021). The palmitic acid percentages in total fatty acid of plantago silages were higher than those of plantago herbages demonstrating that palmitic acid level in silage fermentation did not oxidise or decompose. In the overall assessment, ensilaging of plantago species caused a decreasing of approximately 20% ratio in α-linolenic acid and an increasing of approximately 30% ratio in palmitic acid in total fatty acids according to those of their herbages.
Making silage of green-fresh forage plants provides forage for ruminant nutrition throughout the year. Acidity is important for ideal anaerobic silage fermentation (Tekin and Kara Citation2020). In the silage environment, lactic acid (pKa of 3.86), produced by lactic acid bacteria, is the organic acid, found in the highest concentration in the silage environment. It contributes the most to the decline in pH during anaerobic fermentation of ensiled material because it is about 10 to 12 times stronger than any of the other primary organic acids (acetic acid; pKa of 4.75 and propionic acid; pKa of 4.87) found in silage environment (Kung et al. Citation2018). The lowest pH value and the highest lactic acid level in the silage medium were determined in P. lanceolata silage. These acidity values were the targeted values for quality silage. The higher ammonia-nitrogen level in the silage fluid of P. major silage compared to other silages may have buffered the silage acidity with its alkaline feature. In parallel with the finding that ensiling of P. major herbage decreased the CP content in the forage, and increased the concentration of ammonia-nitrogen in the silage fluid. In the present study, concentrations of AA, the second major acid that inhibits yeasts in silage environments, in plantago silages were <0.2% in DM. Concentrations of BA, which should not be detectable in well-fermented silages, for plantago silages in the present study were at very low levels, especially for P. major and P. lanceolata.
Ruminal fermentation
In vitro gas production technique is a method that can be interpreted for the in vitro fermentation of feed material in the rumen and the energy and digestion level according to the amount of gas produced. In the study, in vitro total gas production and estimated digestion values (ME and OMD) of plantago species were within the expected values for quality forages (Kamalak et al. Citation2011; Kara et al. Citation2016) were found to be at a reasonable level. In the present study, it was observed that there was no difference between the in vitro gas productions values (35–38 ml/0.2 g DM) for herbages of plantago species. Previous researchers (Kara et al. Citation2016; Kara et al. Citation2018) have found that P. lanceolata herbages have higher in vitro gas production and estimated digestion values at 24 hours than those in the present study. Ensiling of plantago species increased in vitro gas production, ME and OMD values, and in vitro digestion values. The in vitro digestion values of P. media silage and P. lanceolata silage were higher than P. major silage. Low values of P. major silage compared to other plantago silages may be due to the failure to reach ideal silage acidity, the significant loss of CP value, and the fermentation of structural carbohydrates. The production amount for studied plantago forages of methane, an important vital gas produced by carbohydrate fermentation in the rumen, was within the values specified for forages (Kara et al. Citation2016; Kara Citation2021). The ensilaging increases the in vitro methane production in parallel with the increase in the in vitro total gas production for P. media and P. lanceolata. In the present study, ensilaging of plantago species increased the concentrations of SCFA (AA, PA, VA, and CA), BCFA (IVA and IBA) and tSCFA in the in vitro fermentation fluid was compatible with the increase in the in vitro gas production and estimated digestion values. Isoacids is the collective term for the branched-chain fatty acids: IBA and IVA and the straight-chain valeric acid, naturally produced in ruminant’ digestive tracts (Andries et al. Citation1987). They are mainly built up from the degradation products of the amino acids valine, isoleucine, leucine and proline and should in turn be used for the biosynthesis of those amino acids and higher branched chain volatile fatty acids. Apajalahti et al. (Citation2019) demonstrated that the 60% of valine, leucine, and isoleucine, untaken with ruminant feedstuffs, was recovered as IBA, 2-methylbutyric, and IVA (products of decarboxylation and deamination), respectively. The concentrations of iso-acids in the in vitro fermentation of P. major herbage were higher than those of P. media and P. lanceolata herbages may be related to the high CP content of P. major herbage. According to previously mentioned results, the low concentrations of iso-acids in P. major silage may be associated with the low CP content in P. major silage. In the present study, the differences in nutrient content with ensiling of plantago herbages changed the SCFA concentration in the in vitro fermentation fluid.
Ruminal microbiome
Ruminal microbiota plays a vital role in the feedstuffs fermentation, gas production (carbon dioxide, methane, etc), SCFA, BCFA and ammonia-nitrogen in the rumen (Patra and Yu Citation2014). Ruminants have various micro-organisms in their rumen, including bacteria, protozoa, fungi and archaea (Kim et al. Citation2011). An 83.40–93.34% of the species belonged to the Bacteria kingdom, 5.44–9.71% to archaea, 0.73–1.30% to unclassified and 0.07–0.2% were Eukaryota. The bacteria community was mainly composed of Bacteriodota and Firmicutes. This was in agreement with previous studies, which presented the rumen microbiota composition in cattle, that reported that these phyla comprise around 90% of the 16S rRNA gene abundance (Delgado et al. Citation2019). The archaea community, rumen methanogens, in the in vitro fermentation fluid of plantago forages in the present study is composed of Euryarchaeota (mainly; Methanobrevibacter and Methanosphaera genus in Methanobacteriales order) and Thermoplasmatota (Methanomassiliicoccales order). These orders have all been characterised as hydrogenotrophic, utilising H2/CO2 or H2/methanol produced by various fermentative bacteria in the anaerobic degradation of plant biomass (Poulsen et al. Citation2013).
The hydrogenotrophic pathway catalysing the conversion of CO2 to methane is dominant in the rumen, and occurs in Methanobrevibacter (Danielsson et al. Citation2017). In the present study, it was observed that the in vitro methane production of P. major herbage (9.57 ml) was higher than that of P. media and P. lanceolata (8.15 and 7.49 ml) herbages, which can be in relation to the high community of Methanobrevibacter (9.68% in the total microbiome) in the in vitro fermentation fluid and AA concentration (an increasing trend) of P. major herbage. The NDF content of P. major herbage was lower than that of other plantago species and the NFC content of it was similar to other herbage. However, the in vitro total gas and methane production of P. major herbage was high, could be attributed to the fact that lignin plus cellulose complexes were not very prominent in this species and cellulose fermentation was higher than those in other herbages. On the other hand, the in vitro methane production of P. major silage (7.03 ml) was lower than those of P. media and P. lanceolata silages (10.66 and 9.94 ml), and the Methanobrevibacter in fermentation fluid of P. major silage was higher than those of P. media and P. lanceolata silages. The complex microbial ecosystem in the rumen produces the SCFA through the fermentation of dietary OM. In the present study, the relative abundance of Prevotellaceae bacteria in the in vitro fermentation fluid of P. major silage was found to be low in those of other plantago silages demonstrating parallel to the low fermentation of OM. Decreased methane production of P. major silage may be related to the decrease in the NDF content with good fermentation of P. major silage in the silage environment and the increase in the NFC content. The methane precursor is SCFA, and methanogen archaea are responsible for converting SCFA to methane. The AA and BA promote methane production, while PA production can be considered a competitive pathway for hydrogen use in the rumen (Moss et al. Citation2000). The BA and VA of ruminal acidifications in P. major silage being lower than those of other silages may be related to low methane production.
In the present study, the high in vitro methane production of P. major herbage was higher than those of other herbages. The low the in vitro methane production of P. major silage was higher those of other silages related to the percentages of palmitic acid, oleic acid and MUFA fatty acid of forages samples (O’Brien et al. Citation2014). A previous study stated that oleic acid supplementations at different percentages in the in vitro fermentation of forage dose-dependent manner decreased in vitro methane production in the rumen (Wu et al. Citation2016). The relative abundance of Prevotellaceae bacteria, which can ferment the structural carbohydrate components (xylan/xyloglucan and pectin) and proteins in the rumen (Seshadri et al. Citation2018), in the in vitro fermentation fluid of P. major silage, high MUFA contents, found to be low in those of other plantago silages can be connected to the decreasing methane production. The P. major silage with high C18:0 and C18:1 (according to other plantago silages) decreased the methane production and relative abundances of archaea and fibrolytic bacteria in the present study in the rumen, parallel to the results of Ding et al. (Citation2012). However, Judy et al. (Citation2019) indicated that increasing C18:3 in the ruminant diet might not affect methane production or the digestibility of the diet.
In the present study, at the genus level of bacteria, Rikenellaceae_RC9_gut_group (18.50–15.51%) and Prevotella (9.82–4.06%) were in relative abundance in the in vitro ruminal fermentation fluid. Huang et al. (Citation2021) stated that at the genus level, the ruminal microbiome of the grazing group showed a higher abundance of Rikenellaceae RC9 gut group and Prevotellaceae UCG-003. Rabee et al. (Citation2022) demonstrated that the dominant genera were RC9_gut_group, Ruminococcus, Saccharofermentans, Butyrivibrio, Succiniclasticum, Selenomonas, and Streptococcus, indicating the critical role of these genera in lignocellulose fermentation in the rumen. In the present study, the high relative abundance of Rikenellaceae_RC9_gut_group in the in vitro ruminal fermentation fluid liquid of plantago herbage and silages with high structural carbohydrate (NDF, ADF) were consistent with the results of the studies (Zened et al. Citation2013; Zhang Citation2017) that feedstuffs materials containing high fibre or high structural carbohydrates increased the relative abundance of ruminal Rikenellaceae_RC9_gut_group bacteria. Prevotellaceae is involved in plant cell wall polysaccharides degradation, and can utilise branched-SCFA and participate in glucose metabolism (Liu et al. Citation2019). The relative abundance of Prevotellaceae UCG-001 in rumen fluid in the present study was similar to the results of Qiu et al. (Citation2022). In the present study, the relative abundance of Prevotellaceae bacteria in the in vitro fermentation fluid of P. major silage was lower than those of other plantago silages. It is thought that the decrease in BCFA (iso-acids; IVA and IBA) in the in vitro fermentation fluid of P. major silage may be related to the decreased relative abundance of Provetella bacteria in the in vitro fermentation fluid. The Prevotellaceae bacteria can also produce acetate and propionic acid by fermentation of structural carbohydrate components (xylan/xyloglucan and pectin) in the rumen (Seshadri et al. Citation2018). The increase in the in vitro total gas production and the AA, PA, VA, and CA concentrations with the ensilaging of plantago species can be associated with the relative abundance of Prevotellaceae bacteria in the rumen fluid. The ensiling process increased the in vitro total gas, acetic acid concentration and relative abundance of Prevotellaceae bacteria in the rumen fluid of P. media and P. lanceolata. The Treponema, positively correlated with dietary fibre (Billenkamp et al. Citation2021), in the in vitro fermentation fluid increased by about 40–50% with the ensilaging of P. media and P. lanceolata in the present study. Liu et al. (Citation2014) showed that Treponema saccharophilum was a pectinolytic bacterium isolated from the bovine rumen. Ensilaging of herbages in the present study increased the PA concentration in the in vitro rumen fluid. Muribaculaceae are predicted to produce PA as a fermentation end product and are abundant and diverse in the guts of mice, although few isolates are available (Smith et al. Citation2021). Besides, the relative abundance of cellulolytic bacteria, such as Ruminococcus, Lachnospiraceae_NK3A20_group, Ruminiclostridium and Lachnospiraceae_UCG-008 was compatible with the results of Wang et al. (Citation2021). In the present study, the relative abundance of genus Sphaerochaeta and Treponema in the Spirochaetaceae family in rumen fluid of plantago silages were higher than those of plantago herbages. Some species within Treponema and Sphaerochaeta in the rumen show pectinolytic activities instead of being pathogens (Xie et al. Citation2018) and also produce AA, which is a lower energy source for the animal in comparison with BA (Abt et al. Citation2012). The production of H2S in the rumen depends on the availability of sulphate reduction by ruminal sulphate-reducing bacteria (Howard and Hungate Citation1976). In the rumen, so many bacteria, fungi and protozoa are present, some bacteria are sulphate-reducing bacteria and these bacteria are anaerobic (Howard and Hungate Citation1976). Desulfovibrio is important sulphate-reducing strain found in the rumen by Howard and Hungate (Citation1976). In the present study, Desulfovibrio ranged from 0.87% to 1.18% in the microbiome of rumen fluid for plantago forages. These bacteria can reduce sulphate into hydrogen sulphide in the animals’ rumen, as Desulfovibrio reduces sulphate to sulphide and methanogens reduce CO2 to produce CH4. Desulfovibrio can also competitively attach to hydrogen ions. In the rumen, Desulfovibrio’s compatibility with methanogens depends mainly on the sulphate levels (Jiang et al. Citation2010).
Conclusion
The P. major herbage was more advantageous than P. media and P. lanceolata in terms of the CP and VLCFA contents of P. major herbage. Compared with other plantago silages, the ensiled P. major silage could not provide a good silage quality (nutrient composition - such as CP and NDF, and silage acidity). The ensiling of plantago herbage positively affected the ruminal fibrolytic bacterial composition (Rikenellaceae_RC9_gut_group, Oscillospiraceae_UCG-002, and Prevotellaceae) of in vitro fermentation fluid. Besides, the in vitro ruminal methane production and community of Methanobrevibacter in the microbiome of P. major herbage were higher than those of P. media and P. lanceolata herbages. In general, the increase in the in vitro total gas production and the acetic, propionic, valeric, and caproic acid concentrations with the ensilaging of plantago species were associated with the increase in the relative abundance of Prevotellaceae bacteria in the rumen fluid.
Ethical approval
This study was approved (number: 22/198) by the Local Ethics Committee for Animal Experiments of Erciyes University, Kayseri, Türkiye.
Acknowledgments
The authors thank the Scientific Research Project Fund of Erciyes University for financial support and The Editing Office of Erciyes University for the manuscript editing.
Disclosure statement
No potential conflict of interest was reported by the author(s).
Data availability statement
The data supporting this study’s findings are available on request from the corresponding author, [initials]. The data are not publicly available due to restrictions [e.g. information that could compromise the privacy of research participants].
Correction Statement
This article has been republished with minor changes. These changes do not impact the academic content of the article.
Additional information
Funding
References
- AOAC. 1995. Official methods of analysis. Arlington (VA): Association of Official Analytical Chemists.
- Abt B, Han C, Scheuner C, Lu M, Lapidus A, Nolan M, Lucas S, Hammon N, Deshpande S, Cheng J-F, et al. 2012. Complete genome sequence of the termite hindgut bacterium Spirochaeta coccoides type strain (SPN1T), reclassification in the genus Sphaerochaeta as Sphaerochaeta coccoides comb. nov. and emendations of the family Spirochaetaceae and the genus Sphaerochaeta. Stand Genomic Sci. 6(2):194–209.
- Alghamdi AA. 2018. Nutritional value and phytochemical constituents of some plantago spp. of ha‟il region, Saudi Arabia. Alghamdi Univ Hail J Sci. 1(1):8–18.
- Altın TB, Barak B, Altın BN. 2012. Change in precipitation and temperature amounts over three decades in central Anatolia, Turkey. ACS. 02(01):107–125.
- Andries JI, Buysse FX, De Brabander DL, Cottyn BG. 1987. Isoacids in ruminant nutrition: Their role in ruminal and intermediary metabolism and possible influences on performances. Animal Feed Sci Technol. 18(3):169–180.
- Apajalahti J, Vienola K, Raatikainen K, Holder V, Moran CA. 2019. Conversion of branched-chain amino acids to corresponding isoacids - an in vitro tool for estimating ruminal protein degradability. Front Vet Sci. 6:311.
- Bariroh NR, Bryant R, Black A. 2021. Effect of maturity and storage duration on plantain (Plantago lanceolata) silage quality. 1st ICADAI 2021, E3S Web of Conferences 306, 01039
- Bıçakçı A, Altunoğlu MK, Tosunoğlu A, Akkaya A, Malyer H, Sapan N. 2011. Allergenic Plantago (plantain) pollen concentrations in Turkey. Asthma Allergy Immun. 9:44–153.
- Billenkamp F, Schnabel K, Hüther L, Frahm J, von Soosten D, Meyer U, Höper D, Beer M, Seyboldt C, Neubauer H, et al. 2021. No hints at glyphosate-induced ruminal dysbiosis in cows. NPJ Biofilms Microbiomes. 7(1):30.
- Danielsson R, Dicksved J, Sun L, Gonda H, Müller B, Schnürer A, Bertilsson J. 2017. Methane production in dairy cows correlates with rumen methanogenic and bacterial community structure. Front Microbiol. 8:226.
- Delgado B, Bach A, Guasch I, González C, Elcoso G, Pryce JE, Gonzalez-Recio O. 2019. Whole rumen metagenome sequencing allows classifying and predicting feed efficiency and intake levels in cattle. Sci Rep. 9(1):11.
- Ding X, Long R, Zhang Q, Huang X, Guo X, Mi J. 2012. Reducing methane emissions and the methanogen population in the rumen of Tibetan sheep by dietary supplementation with coconut oil. Trop Anim Health Prod. 44(7):1541–1545.
- Ersahince AC, Kara K. 2017. Nutrient composition and in vitro digestion parameters of Jerusalem artichoke (Helianthus tuberosus L.) herbage at different maturity stages in horse and ruminant. J Anim Feed Sci. 26(3):213–225.
- Fons F, Rapior S, Gargadennec A, Andary C, Bessiere JM. 1998. Volatile components of Plantago lanceolata (Piantaginaceae). Acta Bot Gallica. 145(4):265–269.
- Guil‐Guerrero JL. 2001. Nutritional composition of Plantago species (P. Major L., P. Lanceolata L., and P. Media L. Ecol Food Nutr. 40(5):481–495.
- Han L, Zhou H. 2013. Effects of ensiling processes and antioxidants on fatty acid concentrations and compositions in corn silages. J Anim Sci Biotechnol. 4(1):48.
- Howard B, Hungate RE. 1976. Desulfovibrio of the sheep rumen. Appl Environ Microbiol. 32(4):598–602.
- Huang C, Ge F, Yao X, Guo X, Bao P, Ma X, Wu X, Chu M, Yan P, Liang C. 2021. Microbiome and metabolomics reveal the effects of different feeding systems on the growth and ruminal development of yaks. Front Microbiol. 12:682989.
- IPCC. 2014. Summary for policymakers. In: Climate Change 2014: Mitigation of Climate Change. Contribution of Working Group III to the Fifth Assessment Report of the Intergovernmental Panel on Climate Change. USA: Cambridge University Press.
- Jiang G, Gutierrez O, Sharma KR, Yuan Z. 2010. Effects of nitrite concentration and exposure time on sulfide and methane production in sewer systems. Water Res. 44(14):4241–4251.
- Judy JV, Bachman GC, Brown-Brandl TM, Fernando SC, Hales KE, Harvatine KJ, Miller PS, Kononoff PJ. 2019. Increasing the concentration of linolenic acid in diets fed to Jersey cows in late lactation does not affect methane production. J Dairy Sci. 102(3):2085–2093.
- Kamalak A, Canbolat O, Ozkan CO, Atalay AI. 2011. Effect of thymol on in vitro gas production, digestibility and metabolizable energy content of alfalfa hay. Kafkas Univ Vet Fak Derg. 17(2):211–216.
- Kara K. 2020. Milk urea nitrogen and milk fatty acid compositions in dairy cows with subacute ruminal acidosis. Veterinarni Medicina. 65( 8):336–345.
- Kara K. 2021. Nutrient matter, fatty acids, in vitro gas production and digestion of herbage and silage quality of yellow sweet clover (Melilotus officinalis L.) at different phenological stages. J Anim Feed Sci. 30(2):128–140.
- Kara K, Aktuğ E, Özkaya S. 2016. Ruminal digestibility, microbial count, volatile fatty acids and gas kinetics of alternative forage sources for arid and semi-arid areas as in vitro. Ital J Anim Sci. 15(4):673–680.
- Kara K, Güçlü BK, Baytok E. 2015. Comparison of nutrient composition and anti-methanogenic properties of different Rosaceae species. J Anim Feed Sci. 24(4):308–314.
- Kara K, Ozkaya S, Baytok E, Guclu BK, Aktug E, Erbas S. 2018. Effect of phenological stage on nutrient composition, in vitro fermentation and gas production kinetics of Plantago lanceolata herbage. Veterinarni Medicina. 63( 6):251–260.
- Kim M, Morrison M, Yu Z. 2011. Status of the phylogenetic diversity census of ruminal microbiomes. FEMS Microbiol Ecol. 76(1):49–63.
- Klindworth A, Pruesse E, Schweer T, Peplies J, Quast C, Horn M, Glöckner FO. 2013. Evaluation of general 16S ribosomal RNA gene PCR primers for classical and next-generation sequencing-based diversity studies. Nucleic Acids Res. 41(1):e1.
- Kung L, Shaver RD, Grant RJ, Schmidt RJ. 2018. Silage review: Interpretation of chemical, microbial, and organoleptic components of silages. J Dairy Sci. 101(5):4020–4033.
- Labreveux ME. 2002. Productivity of forage cultivars of chicory and plantain in the northeast region of the United States. The Pennsylvania State University The Graduate School Depart of Crop and Soil Sciences. A. [Thesis]. in Agronomy. A Thesis in Agronomy, p. 1–112.
- Liu J, Wang JK, Zhu W, Pu YY, Guan L, Liu JX. 2014. Monitoring the rumen pectinolytic bacteria Treponema saccharophilum using real-time PCR. FEMS Microbiol Ecol. 87(3):576–585.
- Liu C, Wu H, Liu S, Chai S, Meng Q, Zhou Z. 2019. Dynamic alterations in yak rumen bacteria community and metabolome characteristics in response to feed type. Front Microbiol. 10:1116.
- Menke KH, Raab L, Salewski A, Steingass H, Fritz D, Schneider W. 1979. The estimation of the digestibility and metabolizable energy content of ruminant feedstuffs from the gas production when they are incubated with rumen liquor. J Agric Sci. 93(1):217–222.
- Menke HH, Steingass H. 1988. Estimation of the energetic feed value obtained from chemical analysis and in vitro gas production using rumen fluid. Anim Res Develop. 28(1):7–55.
- Minnée EMK, Leach CMT, Dalley DE. 2020. Substituting a pasture-based diet with plantain (Plantago lanceolata) reduces nitrogen excreted in urine from dairy cows in late lactation. Livest Sci. 239:104093.
- Moss AR, Jouany JP, Newbold J. 2000. Methane production by ruminants: its contribution to global warming. Ann Zootech. 49(3):231–253.
- Mudrik V, Kosobrukhov A, Knyazeva I, Pigulevskaya T. 2003. Changes in the photosynthetic characteristics of Plantago major plants caused by soil drought stress. Plant Growth Regul. 40(1):1–6.
- NRC. 2001. Nutrient requirements of dairy cattle. 7th ed. Washington (DC): National Academy Press.
- O’Brien AM, Navarro-Villa A, Purcell PJ, Boland TM, O’Kiely AP. 2014. Reducing in vitro rumen methanogenesis for two contrasting diets using a series of inclusion rates of different additives. Anim Prod Sci. 54:41–157.
- Oprica L, Ivan M, Grigore MN, Zamfirache MM. 2015. Antioxidant activity of plantago species in vegetative and flowering stages. Iranian J Public Health. 44(1):142–144.
- Patra AK, Yu Z. 2014. Effects of vanillin, quillaja saponin, and essential oils on in vitro fermentation and protein-degrading microorganisms of the rumen. Appl Microbiol Biotechnol. 98(2):897–905.
- Pommerrenig B, Papini-Terzi FS, Sauer N. 2007. Differential regulation of sorbitol and sucrose loading into the phloem of plantago major in response to salt stress. Plant Physiol. 144(2):1029–1038.
- Poulsen M, Schwab C, Jensen BB, Engberg RM, Spang A, Canibe N, Højberg O, Milinovich G, Fragner L, Schleper C, et al. 2013. Methylotrophic methanogenic Thermoplasmata implicated in reduced methane emissions from bovine rumen. Nat Commun. 4:1428.
- Qiu X, Qin X, Chen L, Chen Z, Hao R, Zhang S, Yang S, Wang L, Cui Y, Li Y, et al. 2022. Serum biochemical parameters, rumen fermentation, and rumen bacterial communities are partly driven by the breed and sex of cattle when fed high-grain diet. Microorganisms. 10(2):323.
- Rabee AE, Sayed Alahl AA, Lamara M, Ishaq SL. 2022. Fibrolytic rumen bacteria of camel and sheep and their applications in the bioconversion of barley straw to soluble sugars for biofuel production. PLoS One. 17(1):e0262304.
- Ramirez-Restrepo CA, Barry TN. 2005. Alternative temperate forages containing secondary compounds for improving sustainable productivity in grazing ruminants. Anim Feed Sci Technol. 120(3-4):179–201.
- Rozentsvet O, Golovko T, Bogdanova E, Tabalenkova G, Kokovkina E. 2015. Lipid content variation in plantago media leaves in response to light conditions. Cuba J Ecosys Ecograph. 5:163.
- Samuelsen AB. 2000. The traditional uses, chemical constituents and biological activities of Plantago major L. J Ethnopharmacol. 71(1-2):1–21.
- Sanderson MA, Labreveux M, Hall MH, Elwinger GF. 2003. Nutritive value of chicory and english plantain forage. Crop Sci. 43:1797–1804.
- Seshadri R, Leahy SC, Attwood GT, Teh KH, Lambie SC, Cookson AL, Eloe-Fadrosh EA, Pavlopoulos GA, Hadjithomas M, Varghese NJ, Hungate1000 project orators, et al. 2018. Cultivation and sequencing of rumen microbiome members from the Hungate1000 Collection. Nat Biotechnol. 36(4):359–367.
- Smith BJ, Miller RA, Schmidt T M. 2021. Muribaculaceae genomes assembled from metagenomes suggest genetic drivers of differential response to acarbose treatment in mice. mSphere. 22;6(6):e0085121.
- Tekin M, Kara K. 2020. The forage quality and the in vitro ruminal digestibility, gas production, organic acids, and some estimated digestion parameters of tomato herbage silage with molasses and barley. Turk J Vet Anim Sci. 44(2):201–213.
- Van Soest PJ, Robertson JB, Lewis BA. 1991. Methods for dietary fiber, neutral detergent fiber and non starch polysaccharides in relation to animal nutrition. J Dairy Sci. 74(10):3583–3597.
- Wang Y, Nan X, Zhao Y, Wang Y, Jiang L, Xiong B. 2021. Ruminal degradation of rumen-protected glucose influences the ruminal microbiota and metabolites in early-lactation dairy cows. Appl Environ Microbiol. 87(2):e01908–20.
- Wang J, Wu W, Wang X, Wang M, Wu F. 2015. An affective GC method for the determination of the fatty acid composition in silkworm pupae oil using a two-step methylation process. J Serb Chem Soc. 80(1):9–20.
- Wu D, Xu L, Tang S, Guan L, He Z, Guan Y, Tan Z, Han X, Zhou C, Kang J, et al. 2016. Influence of oleic acid on rumen fermentation and fatty acid formation in vitro. PLoS One. 11(6):e0156835.
- Xie X, Yang C, Guan LL, Wang J, Xue M, Liu JX. 2018. Persistence of cellulolytic bacteria Fibrobacter and Treponema after short-term corn stover-based dietary intervention reveals the potential to improve rumen fibrolytic function. Front Microbiol. 9:1363.
- Zened A, Combes S, Cauquil L, Mariette J, Klopp C, Bouchez O, Troegeler-Meynadier A, Enjalbert F. 2013. Microbial ecology of the rumen evaluated by 454 GS FLX pyrosequencing is affected by starch and oil supplementation of diets. FEMS Microbiol Ecol. 83(2):504–514.
- Zhang 2017. Research Gi development and rumen microbiome from 0 to 56-day-ole at cashmere goat. China: Northwest A and F University.