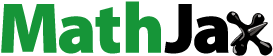
Abstract
One animal-based method to evaluate welfare is the presence of stress. In dairy ruminants, the responses to stressors include the activation of both neuroendocrine and autonomous nervous system that can be evaluated through an endocrine assessment. The present study aimed to validate a radioimmunoassay method for cortisol in buffalo milk. Three formulations (whole and skimmed milk and whey) and three solvents (methanol diethyl ether and dichloromethane) were tested: methanol was characterised by the best extraction efficiency (69.88%), whey cortisol concentrations showed a significant correlation with whole extracted milk and were not affected by fat content variation during the milking session. The RIA used in the present study showed good precision, sensitivity and specificity: the dilutions test indicated the high reproducibility of the results, overlapping of the dilution curve and standard curve highlighted high specificity and the lack of interfering factors by buffalo whey matrix. It is concluded that the present assay suits the cortisol measurement in buffalo milk and the ranges described could be employed in the calibration of a biosensing technologies directly integrated in milking parlour systems.
Buffalo milk whey revealed to be a matrix of great interest because of its high stability in terms of storage, transportation and processing.
RIA method suits the cortisol measurement in buffalo milk
Ranges described can be employed in the calibration of biosensors for non-invasive assessment of cortisol
Highlights
Introduction
Nowadays, ensuring animal welfare is of utmost importance in the production of animal-derived foods and in particular in dairy industry (Hötzel et al. Citation2014). Welfare must be assessed considering the animals and their attempt to cope with the environment (Broom Citation1991). A frequent but accurate welfare assessment requires reliable protocols that can be carried out in a couple of hours (van Eerdenburg et al. Citation2021), although a ‘gold standard’ for welfare assessment is still lacking. Many methods were proposed for assessing animal welfare at farm level called as farm based. Among them, the Animal Needs Index score (ANI 35 L) (Bartussek Citation1999) is focussed on environmental conditions and attributes high and positive scores to pasture. This index has a high repeatability among evaluators and is objective (Amon et al. Citation2001). Other methods described by Capdeville and Veissier (Citation2001) and Whay et al. (Citation2003) involve the direct observations of animals (animal-based). Thus, environmental and animal-based criteria should be included together in an appropriate index for welfare assessment, as proposed by the Welfare Quality® Consortium (Welfare Quality® Citation2009).
One of the main parameters that can be recorded to evaluate welfare in animal-based methods is the presence of stress (Broom Citation2001). Several factors are responsible of stress in dairy ruminants including climatic conditions (De Rensis et al. Citation2015), management techniques (Canaes et al. Citation2009; Marsico et al. Citation2009; Olmos et al. Citation2009) and animal-related factors such as mammary gland health status (Decarvalho et al. Citation2009), lactation stage (Trevisi et al. Citation2009), breed (Negrão Citation2008), parity number (Van Reenen et al. Citation2002) and milk yield (Sevi et al. Citation2001). Responses include behavioural changes, changes to the immune system and activation of both neuroendocrine and autonomous nervous system (Moberg Citation2000); the latter can be evaluated also through endocrine assessment.
Endocrinological analysis can provide a picture of such responses; in particular, cortisol is considered a useful tool to monitor the response of the hypothalamo-pituitary-adrenocortical axis (HPA) to stress (Sevi Citation2009; Kirovski et al. Citation2014). Furthermore, its release over time reflects also the ‘allostatic load’ of the animal, that is the cumulative effect of experiences in daily life, that involve ordinary events (subtle and long-standing life situations) as well as major challenges (McEwen Citation2007). Therefore, the evaluation of the allostatic load of animals could be used as a complement to other welfare assessment methods (McEwen Citation2003).
The radioimmunoassay for steroids used in this study belonged to an in-house method already validated in plasma (Neglia et al. Citation2012), feathers (Frongia et al. Citation2020), hair (Peric et al. Citation2013; Prandi et al. Citation2018), wool (Peric et al. Citation2020) and bovine whey (Comin et al. Citation2005).
So far, cortisol in buffalo milk has never been assayed with immunomethods. The RIA assay is the traditional gold standard method for immunoassays (Reimers et al. Citation1981) as it is sensitive, specific and reproducible.
The present study aimed to validate a reliable radioimmunoassay method to assess cortisol concentration in buffalo milk in order to provide a preliminary data for the calibration of future biosensing technologies for non-invasive assessment of cortisol to be integrated in milking parlour systems.
Materials and methods
Milk sampling represents a non-invasive procedure and within standard farm practices. All experimental procedures and the care of the animals complied to the Italian legislation on animal care (DL n.116, 27/1/1992) and were approved by the Ethical Committee of the University of Naples ‘Federico II’ (Protocol number: 25539-2022).
Animals and milk sampling
The study was carried out at a commercial buffalo dairy farm in southern Italy (Campania region, 41°03′40.6″N − 14°02′16.5″E), where a total of 950 buffaloes were bred.
The trial involved 71 randomly selected Italian Mediterranean dairy buffaloes (Bubalus bubalis) with an average weight of 423.02 ± 3.05 kg and an average age of 4.40 ± 0.29 years, with different parity (33 multiparous and 38 primiparous) and days in milk (DIM = 121.52 ± 8.46). They were maintained in pens with a concrete floor and were milked twice daily in the morning and afternoon; the animals were clinically mastitis-free.
In 63 out of the 71 buffaloes, individual milk samples were collected by the mean of sterile falcon tubes (Falcon® 50 mL, Corning Science, Mexico) from the tank of an automatic sampler (MM15 DeLaval) calibrated by breeders’ association (Campania region Breeders Association, ARAC) and placed in the milking parlour. Thanks to the automated sampler each individual specimen was representative of the animal’s whole milking. After collection, samples were immediately placed into dry ice (−78 °C) and transported to the laboratory where they were stored at −20 °C until lab processing.
An integrative individual milk sampling was performed in the milking parlour on 8 out of the 71 buffaloes. In this case milking was carried out manually by trained professionals and the sampling was performed in two different timeframes: at the beginning and at the end of milking. The samples were collected in sterile falcon tubes (Falcon® 50 mL, Corning Science, Mexico), immediately placed into dry ice (−78 °C) and transported to the laboratory where they were stored at −20 °C until lab processing.
Milk sample preparation for first testing
After thawing, 5 randomly selected milk samples (out of the 63 automatically gathered) were divided into three 5 mL aliquots to be used in analysis as whole milk (first aliquot), skimmed milk (from the second aliquot) and whey (from the third aliquot). The second aliquot of whole milk was skimmed in tubes centrifugated at 2000 × g for 10 min at 4 °C and the fatty supernatant was removed through a pipette obtaining skimmed milk. The third aliquot was used to obtain whey from whole milk that undergone coagulation procedure. Briefly, 400 µL of rennet (60 g of a commercial compound in 250 mL of ultrapure water) were added to 5 mL of whole milk, gently mixed for 3 min at room temperature and incubated at 37 °C for 30 min to complete the clotting process. After incubation, samples were centrifuged at 3500 × g for 10 min at 4 °C and separated from the fat and curd phases supernatant twice.
Whole milk, skimmed milk and whey extraction for first testing
A solvent evaluation was carried out to identify the most efficient one also by the mean of the test on cortisol recovery. Three different solvents were chosen for the extractions: methanol (Sigma-Aldrich, St. Louis, MO, 99.8%), diethyl ether (Sigma-Aldrich, St. Louis, MO, 99.8%) and dichloromethane (Fluka Honeywell, 99.5%). Briefly, to 400 µL of each sample (whole milk, skimmed milk, or whey) obtained as described previously were added 20 µL of antigen tracer (hydrocortisone {cortisol [1,2,6,7-3H (N)]-}, PerkinElmer Life Sciences Inc., Waltham, MA, USA) and kept at 4 °C. After 20 min, 5 mL of one of the three solvents were added to each tube, mixed for 5 min at room temperature and centrifuged for 15 min at 3500 × g and 4 °C. The vials were then kept at −20 °C, and once frozen the solvent was moved to a tube to dry at 37 °C under an airstream suction hood. The remaining residue was dissolved in 0.5 mL of RIA buffer (0.05 M phosphate-buffered saline, pH 7.5, 0.1% BSA).
Milk sample preparation and extraction for second testing
For the second testing the rest of the 63 milk samples collected automatically were divided after thawing in two aliquots to obtain whole milk and whey as described previously. Then, 400 µL of the whole milk and 400 µL of the whey has been extracted adding 5 mL of methanol (Sigma-Aldrich, St. Louis, MO, 99.8%), mixed for 5 min at room temperature and centrifuged for 15 min at 3500 × g and 4 °C. Once having frozen the content of the vials (−20 °C), the solvent was moved to a tube to dry it at 37 °C under an airstream suction hood. The remaining residue was dissolved in 0.5 mL of RIA buffer (0.05 M phosphate-buffered saline, pH 7.5, 0.1% BSA).
Milk sample preparation for third testing
The milk samples collected manually from the 8 buffaloes at the beginning and the end of milking were curdled after thawing as described previously to obtain whey.
Cortisol analysis by RIA
The concentration of cortisol was measured using a solid-phase microtiter radioimmunoassay (RIA). In brief, a 96-well microtiter plate (OptiPlate; PerkinElmer Life Science, Boston, MA, USA) was coated with goat anti-rabbit γ-globulin serum diluted 1:1000 in 0.15 mM sodium acetate buffer (pH 9) and incubated overnight at 4 °C. The plate was then washed twice with RIA buffer (pH 7.5) and incubated overnight at 4 °C with 200 µL of the antibody serum diluted at ratios of 1:20,000 for cortisol (Analytical Antibodies, Bologna, Italy). The cross-reactivities of the anti-cortisol antibody with other steroids were as follows: cortisol, 100%; corticosterone, 1.8%; aldosterone, <0.02%. After washing the plate with RIA buffer, the standards (5–200 pg/well), the quality-control extract, the test sample or extract and the tracer (hydrocortisone {cortisol [1,2,6,7-3H (N)]-}, PerkinElmer Life Science, Boston, MA, USA) were added, and the plate was incubated overnight at 4 °C. The bound hormones were separated from the free hormones by decanting and washing the wells in RIA buffer. After the addition of 200 µL of scintillation cocktail (MicroScint-20, PerkinElmer Life Science, Boston, MA, USA), the plate was counted on a β-counter (Top-Count, PerkinElmer Life Science, Boston, MA, USA). Moreover, a 50 µL sample spiked with the tracer and 200 µL of scintillation cocktail were placed in the plate and counted. The extraction recovery value for each unknown well was determined by expressing the count rate for that well as a percentage of the counts added before extraction. The cortisol content of the unknown well was then corrected by the extraction recovery percentage for that well and the cortisol content of the unknown sample expressed in pg/mL.
Cortisol RIA validation tests
Each validation tests involved different pools constituted by five whey samples and were analysed by quintuple. The parallelism test consisted of determining the deviation from the standard curve of a series of whey samples containing known amounts of cortisol, they were prepared by serial dilution of whey samples from animals that showed high concentrations of cortisol. Linear regression was used to determine if whey samples and the standard cortisol curve deviated from parallelism.
The recovery test was conducted to evaluate the system response to an increasing amount of cortisol standard added to a whey sample with low concentration. The percentage of recovery was determined as follows:
The sensitivity of the curve was as the hormone concentration resulting in a displacement of the labelled hormone at least two standard deviations from maximal binding.
Precision was estimated by repeatedly assaying samples in the inter- and intra-assay and was expressed as the coefficients of variation (CV%).
Statistical analysis
Statistical analyses were carried out using SPSS (28.0) for Windows 10 (SPSS Inc., Chicago, IL). To compare cortisol concentrations between different milk formulations and sampling timeframes Student’s t-test was used. Pearson correlation test was performed to assess possible correlations between different milk formulations. Results are expressed as mean ± standard error mean (SEM). A statistically significant difference was accepted at p < .05.
Results
First testing – solvent efficiency
The test on cortisol recovery to evaluate the solvents extraction efficiency gave the following rates when used dichloromethane: 52.03 ± 3.42% on whole milk, 60.71 ± 2.17% on skimmed milk, 60.00 ± 1.75% on whey. Diethyl ether showed a recovery of 54.76 ± 5.69%, 63.03 ± 1.87% and 68.67 ± 1.64% respectively for whole, skimmed and whey. Finally, methanol reported recoveries of 58.89 ± 3.47%, 59.09 ± 4.03% and 69.88 ± 1.43% for whole, skimmed and whey, respectively.
The cortisol concentrations recorded in the three types of samples (whole milk, skimmed milk and whey) with the three solvents tested for hormone extraction are described in Figure .
Second testing
Based on the first testing results only methanol was chosen as solvent for the subsequent second testing. The cortisol concentrations (pg/mL) of the 63 milk samples that were assayed as whole extracted milk, extracted whey and whey are displayed in Figure .
Figure 2. Cortisol concentrations (pg/mL) in 63 milk samples assessed as whole extracted milk, extracted whey and whey. When relevant the extraction was made with methanol.
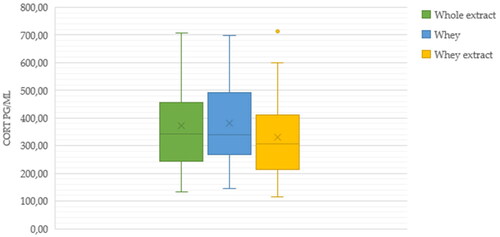
No significant differences were recorded between the three formulations.
The cortisol concentrations (pg/mL) detected in whey and whole extracted milk showed a significant correlation (p < .001, correlation coefficient + 0.51) (Figure ).
Third testing
The whey cortisol concentrations of milk sampled in the two different timeframes of the milking session are displayed in Figure along with the percentage of cortisol’s variation and the difference in whey cortisol concentrations between the beginning and the end of milking. Average values were 328.84 and 250.29 pg/mL, respectively, for beginning and end of milking.
Figure 4. Cortisol concentrations (pg/mL) of whey from milk sampled at the beginning and at the end of milking session. Difference (Δ) between the two sampling times was calculated as Cb − Ce; percentage of cortisol’s variation (variation %) was calculated as follows: [(Cb − Ce)/ Cb] *100. Cb is the whey cortisol concentration at the beginning of the milking and Ce the cortisol at the end of the milking session.
![Figure 4. Cortisol concentrations (pg/mL) of whey from milk sampled at the beginning and at the end of milking session. Difference (Δ) between the two sampling times was calculated as Cb − Ce; percentage of cortisol’s variation (variation %) was calculated as follows: [(Cb − Ce)/ Cb] *100. Cb is the whey cortisol concentration at the beginning of the milking and Ce the cortisol at the end of the milking session.](/cms/asset/3d862dbb-24da-4a3b-9148-e9198d1befff/tjas_a_2147868_f0004_c.jpg)
Among the two timeframes sampled for milk, whey cortisol concentrations ranged between a minimum of 152.10 (pg/mL, end of milking) and a maximum of 499.20 (pg/mL, beginning of milking). No significant differences were highlighted by comparing the two concentrations (p > .05).
RIA validation
The parallelism between the dilution curves and the standard one indicated that milk cortisol and standard cortisol reacted identically to the antibodies because of the high correlation (r = 0.99) observed between the concentrations obtained and those expected (Figure ). The relationship between whey cortisol concentrations and the standard cortisol curve was given by the equation y = 1.02x − 0.97 (Figure ).
Figure 5. Relationship between theoretical whey cortisol concentration and the observed whey cortisol.
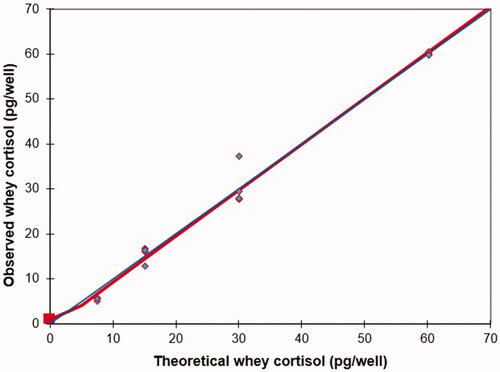
The recovery test aimed to evaluate the reaction of the system to an increasing amount of cortisol standards and revealed a recovery rate of 104.4 ± 6.1% (mean ± SD).
The assay sensitivity was 16.8 pg/mL. Whey sample, in repeated determinations, showed intra- and inter-assay coefficients of variation of 7.6% and 12.7%, respectively.
Discussion
The assessment of cortisol concentration in biological samples is one of the main tools to evaluate the stress in animals. E.g. cortisol has been used to study automatic milking systems (Gygax et al. Citation2006) versus conventional milking parlours, or to investigate the association with animal behaviour (Fukasawa and Tsukada Citation2010). It is known that steroid hormones can permeate cell membranes and may cross the epithelial blood-milk barrier (Rushen et al. Citation2008). A high correlation between milk and plasma cortisol concentrations after ACTH administration has been found (Thinh et al. Citation2011). Considering that milking for dairy cows is a routinary process, milk might represent a non-invasive alternative to blood in HPA axis evaluation (Diaz et al. Citation2013): it can be sampled without any extra animal manipulation and offers the advantage to monitor hormones concentration directly in milking parlour in line or at the official milk recording.
The purposes of the present study were to validate a radioimmunoassay to assess for the first-time cortisol concentration in buffalo milk. The RIA assay is the traditional gold standard method for immunoassays (Reimers et al. Citation1981) as it is sensitive, specific and reproducible. It would provide essential evidence, as the physiological cortisol concentrations range of buffalo species is, for the practical purpose of calibrating a sensor able of automatically measuring cortisol in buffalo milk. Moreover, in this study we considered two variables that could interfere with sensor’s activity on a farm basis: the milk formulation and the sampling timeframe. Differently from Claycomb and Delwiche (Citation1998) who integrated a rapid enzyme immunoassay (EIA) for progesterone in an on-line sensor, the RIA technology won’t be part of the device developed.
According to previous studies performed on bovine milk, three formulations were chosen: whole milk (Butler and Des Bordes Citation1980), skimmed milk (Gellrich et al. Citation2015) and whey (Comin et al. Citation2005).
Two out of three milk formulations showed some criticalities concerning their versatility in sample preparation/using. Great attention had to be paid when pipetting the whole milk samples because of the lipidic film that adhered on the surface of the pipette tips. In the literature it has been reported that milk lipids can be detrimental to give an accurate measurement of cortisol (Butler and Des Bordes Citation1980). Therefore, it has been hypothesised that the removal of fat would make the pipetting easier without affecting the concentrations of cortisol in milk. Nevertheless, milk from Italian Mediterranean water buffaloes is characterised by high fat content (approximately 8.00%) and favourable coagulation characteristics (Costa et al. Citation2020). These features conditioned the skimming procedure, that turn out to be troublesome and time-consuming. Conversely, the whey proved to be a matrix of great interest: coagulation process was effective and timesaving in buffalo milk too and allowed to remove both peptide and lipidic interference. In addition, whey has already been described as a trustworthy milk formulation to measure a steroid such as progesterone in cow milk (Comin et al. Citation2005). As usual for steroid hormones, to exclude any possible interference due to the matrix components, the analytical measurement can be preceded by an extraction step. The extraction solvent must disrupt the binding of the steroid to protein and must extract the steroid of interest quantitatively and leave behind in the aqueous medium non-specific interfering substances (Makin et al. Citation2010).
Several extraction protocols have been described in ruminants reporting different procedures. We choose to refer to Hagen et al. (Citation2004) who performed extraction of bovine milk with diethyl ether and Castro-Gómez et al. (Citation2014) who extracted whole milk with a dichloromethane-methanol solution. According to our data, methanol was characterised by the best results based on cortisol recovery to evaluate the solvents extraction efficiency compared to diethyl ether and dichloromethane. Also, visually the samples extracted with methanol formed a homogenous solution after the solvent addition and a clear separation between the two phases after centrifugation. Furthermore, methanol has been already used for steroid extraction in several studies on different matrices (Gleixner and Meyer Citation1997; Ashley et al. Citation2011; Palme et al. Citation2013) providing convenient and efficient extraction. This interesting finding leads to point at methanol as the most reliable solvent for steroid analysis in buffalo milk.
Subsequently, the evaluation of the biological matrix to be used in milk cortisol evaluation has been extended to a larger sample size. We reported the absence of significant differences (p > .05) in cortisol concentrations among the formulations, suggesting that all of them can be used in cortisol measurement, but a significant correlation was found between whey and whole extracted milk. In any case, considering that the whole milk was hard to store before analysis and to handle throughout sample preparation, milk whey was chosen as the best matrix to be used because of its high stability in terms of storage, transportation and processing.
Some modifications have been reported in bovine milk composition during milking session (Nielsen et al. Citation2005), with a marked increment in milk fat concentration at the beginning of milking and a small decrement in non-fat milk solids at the end of the session. The exact reason for such variation remains unclear, but several theories have been proposed: the progressive filtration of milk fat globule clusters through the mammary ducts, the mechanical shearing of alveolar contractions and decreasing adsorption of fat globule to the alveolar wall during milking (Rico et al. Citation2014). Moreover, a circadian rhythm of milk fatty acids concentration has been reported and it may be linked to a stratification of the fat contentand fatty acids profile of the cistern and alveolar milk (Daly et al. Citation1993; Rottman et al. Citation2014). Given that buffalo milk has higher fat concentration than bovine milk and the present study aimed to provide preliminary data for sensor-based technologies, an integrative analysis was performed to study if the high fat percentage of buffalo milk and sampling timeframe could affect measurements (Pope et al. Citation1976) and to properly describe what should be the best timeframe to be sampled throughout milk ejection.
Our findings revealed that whey cortisol concentrations were not affected by fat content variation during the milking session, showing no significant difference between the concentrations of the two timeframes sampled (p > .05). Moreover, endpoint cortisol concentrations were always lower than the beginning with a variation always less than 0.1 ng/mL. Such evidence seems to suggest that cortisol concentration in whey doesn’t significantly change with the increasing amount of fat in buffalo milk.
The RIA analysis method used in the present study showed good precision, sensitivity and specificity also for determining cortisol in buffalo whey. The analysis of cortisol concentrations in buffalo whey resulted to be feasible and reproducible; the dilutions tested indicated the high reproducibility of the results. Overlapping of the dilution curve and standard curve indicates the method’s high specificity and the lack of interfering factors by buffalo whey matrix.
Conclusions
To our knowledge, this is the first study aimed to measure cortisol in buffalo milk matrix.
The measurement of cortisol in buffalo milk has been evaluated step-by-step, approaching the potential interferences and variables given by the peculiarity of buffalo milk itself. This aimed to provide reliable and solid data for the calibration of future biosensing technologies for non-invasive assessment of cortisol to be integrated in milking parlour systems. In case of extraction, a more time-consuming procedure, it has been demonstrated the necessity to work with a suitable solvent for buffalo milk, but the results have also shown that it is possible to opt for a more versatile matrix such as whey without losing in quality of the data obtained in assay. The RIA method revealed good sensitivity and specificity for measuring cortisol in buffalo milk whey, the present assay suits the cortisol measurement in buffalo milk and the ranges described can be employed in the calibration of a biosensing method for non-invasive assessment of cortisol directly integrated in milking parlour systems.
Ethical approval
All experimental procedures and the care of the animals complied to the Italian legislation on animal care (DL n.116, 27/1/1992) and were approved by the Ethical Committee of the University of Naples ‘Federico II’ (Protocol number: 25539-2022).
Acknowledgements
The authors gratefully acknowledge Dr. Antonella Comin whose guidance has allowed the development of this research.
Disclosure statement
No potential conflict of interest was reported by the author(s).
Data availability statement
The data that support the findings of this study are available from the corresponding author, upon reasonable request.
Additional information
Funding
References
- Amon T, Amon B, Ofner E, Boxberger J. 2001. Precision of assessment of animal welfare by the TGI35L Austrian Needs Index. Acta Agric Scand Sect A Anim Sci Suppl. 30:114–117.
- Ashley NT, Barboza PS, Macbeth BJ, Janz DM, Cattet MRL, Booth RK, Wasser SK. 2011. Glucocorticosteroid concentrations in feces and hair of captive caribou and reindeer following adrenocorticotropic hormone challenge. Gen Comp Endocrinol. 172(3):382–391.
- Bartussek H. 1999. A review of the animal needs index (ANI) for the assessment of animals’ well-being in the housing systems for Austrian proprietary products and legislation. Livest Prod Sci. 61(2-3):179–192.
- Broom DM. 1991. Animal welfare: concepts and measurement. J Anim Sci. 69(10):4167–4175.
- Broom DM. 2001. Coping, stress and welfare. In: Broom DM, editor. Coping with challenge: welfare in animals including humans. Berlin: Dahlem University Press; p. 1–12.
- Butler WR, Des Bordes CK. 1980. Radioimmunoassay technique for measuring cortisol in milk. J Dairy Sci. 63(3):474–477.
- Canaes TS, Negrão JA, Paiva FA, Zaros M, Delgado TF. 2009. Physiologic and productive responses of Alpine goats submitted to transportation to a new dairy location. Arq Bras Med Vet Zootec. 61(4):935–940.
- Capdeville J, Veissier I. 2001. A method of assessing welfare in loose housed dairy cows at farm level, focusing on animal observations. Acta Agric Scand Sect A Anim Sci. 51(1):62–68.
- Castro-Gómez P, Fontecha J, Rodríguez-Alcalá LM. 2014. A high-performance direct transmethylation method for total fatty acids assessment in biological and foodstuff samples. Talanta. 128:518–523.
- Claycomb RW, Delwiche MJ. 1998. Biosensor for on-line measurement of bovine progesterone during milking. Biosens Bioelectron. 13(11):1173–1180.
- Comin A, Renaville B, Marchini E, Maiero S, Cairoli F, Prandi A. 2005. Technical note: direct enzyme immunoassay of progesterone in bovine milk whey. J Dairy Sci. 88(12):4239–4242.
- Costa A, De Marchi M, Battisti S, Guarducci M, Amatiste S, Bitonti G, Borghese A, Boselli C. 2020. On the effect of the temperature-humidity index on buffalo bulk milk composition and coagulation traits. Front Vet Sci. 7:577758.
- Daly SEJ, Di Rosso A, Owens RA, Hartmann PE. 1993. Degree of breast emptying explains changes in the fat content, but not fatty acid composition, of human milk. Exp Physiol. 78(6):741–755.
- Decarvalho M, Simensen E, Fjerdingby N, Larsen S, Zanella A. 2009. Measuring welfare indicators in dairy goats throughsalivary cortisol and somatic cell counts (SCC). In Husdyrforsøksmøtet Lillestrøm Norge. Ås, Norway: Norwegian University of Life Sciences (UMB); p. 499–502.
- De Rensis F, Garcia-Ispierto I, López-Gatius F. 2015. Seasonal heat stress: clinical implications and hormone treatments for the fertility of dairy cows. Theriogenology. 84(5):659–666.
- Diaz JR, Alejandro M, Romero G, Moya F, Peris C. 2013. Variation in milk cortisol during lactation in Murciano-Granadina goats. J Dairy Sci. 96(2):897–905.
- Frongia G, Peric T, Leoni G, Satta V, Berlinguer F, Muzzeddu M, Prandi A, Naitana S, Comin A. 2020. Assessment of cortisol and DHEA concentrations in griffon vulture (Gyps fulvus) feathers to evaluate its allostatic load. Ann Anim Sci. 20(1):85–96.
- Fukasawa M, Tsukada H. 2010. Relationship between milk cortisol concentration and the behavioral characteristics of postpartum cows introduced to a new group. Anim Sci J. 81(5):612–617.
- Gellrich K, Sigl T, Meyer HHD, Wiedemann S. 2015. Cortisol levels in skimmed milk during the first 22 weeks of lactation and response to short-term metabolic stress and lameness in dairy cows. J Anim Sci Biotechnol. 6(1):31.
- Gleixner A, Meyer HHD. 1997. Detection of estradiol and testosterone in hair of cattle by HPLC/EIA. Fresenius J Anal Chem. 357(8):1198–1201.
- Gygax L, Neuffer I, Kaufmann C, Hauser R, Wechsler B. 2006. Milk cortisol concentration in automatic milking systems compared with auto-tandem milking parlors. J Dairy Sci. 89(9):3447–3454.
- Hagen K, Lexer D, Palme R, Troxler J, Waiblinger S. 2004. Milking of Brown Swiss and Austrian Simmental cows in a herringbone parlour or an automatic milking unit. Appl Anim Behav Sci. 88(3-4):209–225.
- Hötzel MJ, Longo C, Balcão LF, Cardoso CS, Costa JH. 2014. A survey of management practices that influence performance and welfare of dairy calves reared in southern Brazil. PLoS One. 9(12):e114995.
- Kirovski D, Snoj T, Nedic S, Nedic D, Cebulj-Kadunc N, Kobal S. Non-invasive methods for detecting chronic stress in dairy cows. In Proceedings of the First Dairy Care Conference, Copenhagen, Denmark, 22–23 August 2014; p. 46.
- Makin HLJ, Honour JW, Shackleton CHL, Griffiths WJ. 2010. General methods for the extraction, purification, and measurement of steroids by chromatography and mass spectrometry. In: Makin H, Gower D, editors. Steroid analysis. Dordrecht: Springer. p. 163–282.
- Marsico G, Micera E, Dimatteo S, Minuti F, Vicenti A, Zarrilli A. 2009. Evaluation of animal welfare and milk production of goat fed on diet containing hydroponically germinating seeds. Ital J Anim Sci. 8(sup2):625–627.
- McEwen BS. 2003. Interacting mediators of allostasis and allostatic load: towards an understanding of resilience in aging. Metabolism. 52(10 Suppl 2):10–16.
- McEwen BS. 2007. Physiology and neurobiology of stress and adaptation: central role of the brain. Physiol Rev. 87(3):873–904.
- Moberg GP. 2000. Biological response to stress: implications for animal welfare. In: Moberg GP, Mench JA, editors. The biology of animal stress. Oxon: CABI Publishing; p. 1–21.
- Neglia G, Vecchio D, Russo M, Di Palo R, Pacelli C, Comin A, Gasparrini B, Campanile G. 2012. Efficacy of PGF(2α) on pre-ovulatory follicle and corpus luteum blood flow. Reprod Domest Anim. 47(1):26–31.
- Negrão JA. 2008. Hormone release and behavior during suckling and milking in Gir, Gir × Holstein, and Holstein cows. J Anim Sci. 86(13 Suppl):21–26.
- Nielsen NI, Larsen T, Bjerring M, Ingvartsen KL. 2005. Quarter health, milking interval, and sampling time during milking affect the concentration of milk constituents. J Dairy Sci. 88(9):3186–3200.
- Olmos G, Mee JF, Hanlon A, Patton J, Murphy JJ, Boyle L. 2009. Peripartum health and welfare of Holstein-Friesian cows in a confinement-TMR system compared to a pasture-based system. Anim. Welf. 18:467–476.
- Palme R, Touma C, Arias N, Dominchin MF, Lepschy M. 2013. Steroid extraction: get the best out of faecal samples. Wiener Tierarztliche Monatsschrift. 100(9-10):238–246.
- Peric T, Comin A, Corazzin M, Montillo M, Cappa A, Campanile G, Prandi A. 2013. Short communication: hair cortisol concentrations in Holstein-Friesian and crossbreed F1 heifers. J Dairy Sci. 96(5):3023–3027.
- Peric T, Comin A, Corazzin M, Montillo M, Prandi A. 2020. Comparison of AlphaLISA and RIA assays for measurement of wool cortisol concentrations. Heliyon. 6(10):e05230.
- Pope GS, Majzlik I, Ball PJH, Leaver JD. 1976. Use of progesterone concentration in plasma and milk in the diagnosis of pregnancy in domestic cattle. Brit Vet J. 132(5):497–506.
- Prandi A, Peric T, Corazzin M, Comin A, Colitti M. 2018. A first survey on hair cortisol of an alpine ibex (Capra ibex ibex) population. Anim Sci Pap Rep. 36:57–74.
- Reimers TJ, Cowan RG, Davidson HP, Colby ED. 1981. Validation of radioimmunoassay for triiodothyronine, thyroxine, and hydrocortisone (cortisol) in canine, feline, and equine sera. Am J Vet Res. 42(11):2016–2021.
- Rico DE, Marshall ER, Choi J, Kaylegian KE, Dechow CD, Harvatine KJ. 2014. Within-milking variation in milk composition and fatty acid profile of Holstein dairy cows. J Dairy Sci. 97(7):4259–4268.
- Rottman LW, Ying Y, Zhou K, Bartell PA, Harvatine KJ. 2014. The daily rhythm of milk synthesis is dependent on the timing of feed intake in dairy cows. Physiol Rep. 2(6):e12049.
- Rushen J, de Passillé AM, von Keyserlingk MAG, Weary DM. 2008. The welfare of cattle publisher. Dordrecht: Springer.
- Sevi A. 2009. Animal-based measures for welfare assessment. Ital J Anim Sci. 8(sup2):904–911.
- Sevi A, Annicchiarico G, Albenzio M, Taibi L, Muscio A, Dell’Aquila S. 2001. Effects of solar radiation and feeding time on behavior, immune response, and production of lactating ewes under high ambient temperature. J Dairy Sci. 84(3):629–640.
- Thinh NC, Yoshida C, Long ST, Yusuf M, Nakao T. 2011. Adrenocortical response in cows after intramuscular injection of long-acting adrenocorticotropic hormone (tetracosactide acetate zinc suspension). Reprod Domest Anim. 46(2):296–300.
- Trevisi E, Lombardelli R, Minuti A, Bertoni G. 2009. Adrenal responsiveness to a low-dose ACTH challenge in early and late lactating dairy cows. Ital J Anim Sci. 8(sup2):661–663.
- van Eerdenburg FJCM, Di Giacinto AM, Hulsen J, Snel B, Stegeman JA. 2021. A new, practical animal welfare assessment for dairy farmers. Animals. 11(3):881.
- Van Reenen CG, Van der Werf JTN, Bruckmaier RM, Hopster H, Engel B, Noordhuizen JPTM, Blokhuis HJ. 2002. Individual differences in behavioral and physiological responsiveness of primiparous dairy cows to machine milking. J Dairy Sci. 85(10):2551–2561.
- Welfare Quality®. 2009. Welfare Quality® assessment protocol for cattle. Lelystad: Welfare Quality® Consortium.
- Whay HR, Main DCJ, Green LE, Webster AJF. 2003. An animal-based welfare assessment of group-housed calves on UK dairy farms. Anim Welf. 12(4):611–617.