abstract
Yeast prions are heritable protein-based elements, most of which are formed of amyloid aggregates that rely on the action of molecular chaperones for transmission to progeny. Prions can form distinct amyloid structures, known as ‘strains’ in mammalian systems, that dictate both pathological progression and cross-species infection barriers. In yeast these same amyloid structural polymorphisms, called ‘variants’, dictate the intensity of prion-associated phenotypes and stability in mitosis. We recently reported that [PSI+] prion variants differ in the fundamental domain requirements for one chaperone, the Hsp40/J-protein Sis1, which are mutually exclusive between 2 different yeast prions, demonstrating a functional plurality for Sis1.Citation1 Here we extend that analysis to incorporate additional data that collectively support the hypothesis that Sis1 has multiple functional roles that can be accomplished by distinct sets of domains. These functions are differentially required by distinct prions and prion variants. We also present new data regarding Hsp104-mediated prion elimination and show that some Sis1 functions, but not all, are conserved in the human homolog Hdj1/DNAJB1. Importantly, of the 10 amyloid-based prions indentified to date in Saccharomyces cerevisiae, the chaperone requirements of only 4 are known, leaving a great diversity of amyloid structures, and likely modes of amyloid-chaperone interaction, largely unexplored.
Abbreviations
ATP | = | adenosine triphosphate |
SDSPAGE | = | sodium dodecyl sulfate polyacrylamide gel electrophoresis |
SDDAGE | = | semi-denaturing detergent agarose gel electrophoresis |
Yeast prions are protein-based heritable elements found in the baker's yeast Saccharomyces cerevisiae, of which 12 have been identified to date.Citation2 Most yeast prions (10 of 12) are formed from amyloid aggregates of normally functional proteins, the 3 best-studied being [PSI+], [RNQ+] (often called [PIN+]), and [URE3].Citation3 Amyloid-based prions propagate to daughter cells by cytosolic transfer of small protein aggregates during cell division.Citation4 These amyloid aggregates, capable of replicating once inside the daughter cell and thereby recapitulating the prion phenotype, are commonly referred to as seeds, or propagons.Citation5
Analogous to viruses, which hijack the cellular replication machinery for their own propagation, yeast prions have become inexorably reliant on the action of the cytosolic molecular chaperone machinery for their propagation, most critically for the generation of additional propagons to buffer against dilution during mitosis.Citation4,6,7 Central to this process is the disaggregase Hsp104, a hollow, cylindrical-shaped hexamer with 12 functional Walker-type ATPase domains.Citation4,8 Hsp104 is thought to fragment amyloid aggregates to generate new propagons by translocation of individual monomeric prion protein units through its central cavity at the expense of ATP.Citation6,7 However, like its bacterial homolog ClpB, Hsp104 first requires the action of the Hsp70 chaperone system, and requisite co-chaperone Hsp40s (hereafter called J-proteins), for the disaggregation of amyloids in vivo.Citation9–11 In agreement with that notion, the yeast cytosolic J-protein Sis1 is required for all 4 prions ([PSI+], [RNQ+], [URE3], and [SWI+]) for which data are currently available,Citation10,12,13 indicating that it is likely an integral part of the so called “prion chaperone machinery” along with Hsp104 and the primary cytosolic Hsp70 Ssa.Citation4 Intriguingly, Sis1 is also capable of directing bacterial chaperones to propagate prions in yeast.Citation14 Because Sis1 is likely required for all amyloid-based yeast prions,Citation13 and because it also binds to other non-prion amyloids in yeast,Citation15,16 we have speculated recently that Sis1 may act as a general amyloid recognition factor,Citation1 recruiting Hsp70, and vicariously other chaperones like Hsp104, to prion aggregates and other amyloids. Current models assert that Ssa mediates a productive interaction with Hsp104 that allows threading of the prion polypeptide into the central cavity of Hsp104 and Sis1 is likely required to productively recruit Ssa to aggregates and stimulate prion peptide binding.Citation9,17 However, the precise biochemical mechanisms through which Sis1 accomplishes its essential functions in prion propagation are unclear because, though apparently universally essential, the specific requirements for its function by different prions vary dramatically,Citation1,10,18–20 an issue that is only further complicated by the existence of prion strains/variants (amyloid polymorphisms).Citation21
The existence of multiple structural amyloid variants formed by a given protein is a fundamental aspect of prion biology.Citation21–23 Originally termed ‘prion strains’, to denote specific isolates with distinct pathologies, amyloid polymorphisms are more often called ‘prion variants’ in yeast.Citation4,21 Studies of prion variants are important because amyloid structural variation in mammalian prions greatly affects species transmission barriers in addition to disease pathology.Citation23 In yeast, prion variants are often characterized as ‘strong’ or ‘weak’ denoting the intensity of the observed prion phenotype and, at least for the prion [PSI+], the degree of mitotic stability.Citation3,22 We and others have recently reported that amyloid structural polymorphism profoundly affects the observed chaperone requirements by yeast prions, particularly with respect to Sis1.Citation1,24–26 Here, we will review our most recent findings on this topic,Citation1 present some additional data, and discuss these results in the light of the results of 2 recent complementary investigations from other labs.Citation19,26 Taken together, the data from multiple investigations points to a model in which combinations of Sis1 domains can cooperate to perform functions that are differentially required by distinct prions and prion variants. Some, but not all, of these functions are conserved in the human homolog Hdj1/DNAJB1.
Domain Requirements for SIS1 are strongly dependent upon [PSI+] Amyloid Polymorphisms
Sis1 is a multi-domain protein with an N-terminal J-domain followed by 2 unstructured regions of high glycine content, termed the glycine/phenylalanine-rich (G/F) and glycine/methionine-rich (G/M) regions ().Citation27 The C-terminal half of the protein contains a domain known to bind model hydrophobic peptides (CTD1), followed by a second putative peptide-binding domain of similar structure (CTD2) and a dimerization domain (DD).Citation27 Initially we focused our recent investigation on 2 well-characterized constructs of Sis1: Sis1–121, which possesses only the J-domain and G/F region, and Sis1-ΔG/F, which lacks only the G/F region ().Citation24 Both of these constructs propagate strong [PSI+] variants, while Sis1–121, but not Sis1-ΔG/F, maintains multiple variants of [RNQ+].Citation10,20,24,25 On the basis of these prior observations, we wondered whether [PSI+] prion variation would affect Sis1 requirements and, if so, would weak [PSI+] variants resemble [RNQ+] in their requirements for Sis1 function? We found that while all 4 strong [PSI+] variants that we examined were similarly maintained by both Sis1 constructs, the distinctions between weak and strong variants were significant.Citation1 Weak [PSI+] variants were stable when Sis1-ΔG/F is expressed but, surprisingly, could not be maintained by Sis1–121, indicating that these variants have markedly different requirements for Sis1 than either strong variants or the [RNQ+] variant used in our study.Citation1 One important question when evaluating these experimental results is whether or not they represent an inability of the chaperone to propagate the prion, or rather, an induced selection for a small population of prion-free cells which then overtake the culture due to induced prion toxicity. For example, others have observed that [PSI+] becomes cytotoxic when Sis1 activity is severely compromised in some strain backgrounds.Citation19,20,26 Likewise we observed [PSI+]-dependent toxicity in our strains when Sis1 was repressed.Citation1 By examining growth rates of cells with weak and strong variants during Sis1 repression, we were able to confirm the previous assertion made by Kirkland et al. that [PSI+]-dependent toxicity is positively correlated to [PSI+] variant stability, i.e., the number of propagons per cell.Citation1,20 However, the results of our experiments did not match those that would be expected if curing were due to a toxicity-dependent selection for [psi−] cells, since curing would be expected to occur more often for the more cytotoxic strong variants than for weak variants. Because we observed exactly the opposite pattern of loss, we concluded that prion-loss was due to an inability of specific Sis1 constructs to support prion propagation.Citation1 Importantly, we were also able to confirm all results in 2 distinct yeast genetic backgrounds, allowing us to rule out the possibility that an uncharacterized polymorphism of a particular lab strain is responsible for a specific experimental outcome.Citation1
Figure 1. Primary structure diagrams of Sis1 and Hdj1 (DNAJB1) expression constructs described in this manuscript. Protein regions are denoted using the following notation: J, J-domain; G/F, glycine/phenylalanine-rich region; G/M, glycine/methionine-rich region; CTD1/2, C-terminal peptide-binding domains I and II; DD, dimerization domain.Citation1,27 Dashed lines indicate where a region had been deleted.
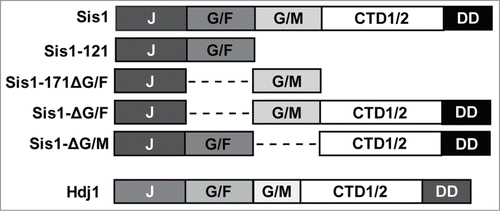
The glycine-rich regions of Sis1 are critical for both cell viability and prion propagation.Citation28 Because the G/M region is missing from Sis1–121 but maintained in Sis1-ΔG/F, we wondered whether this region might be capable of propagating weak [PSI+] variants when expressed without the C-terminal domains but still expressed in cis with the J-domain. Indeed, at least one weak variant, [PSI+]Sc37, was able to propagate when only Sis1–171ΔG/F () was expressed,Citation1 leading us to suspect that perhaps the G/M region is essential for weak [PSI+] variant propagation in the same way that the G/F region appears to be essential for [RNQ+] propagation, regardless of the variant.Citation24,25,28 Unexpectedly, Sis1-ΔG/M propagated all variants of [PSI+] and, moreover, propagated all variants without an apparent change in aggregate size visible by semi-denaturing detergent agarose gel electrophoresis (SDDAGE).Citation1 Because we repeatedly noticed aggregate size increases for weak variants propagated by either minimal construct (Sis1–121 or Sis1–171ΔG/F), these results were particularly informative in 2 ways: 1) they demonstrate that either glycine-rich region (G/F or G/M) can support cell viability and weak [PSI+] propagation as long as the proper protein context is provided at the C-terminus, and 2) both minimal constructs exhibit fragmentation defects that are completely ameliorated when the C-terminal domains are added back, revealing a previously unappreciated role for the C-terminal domains of Sis1 in [PSI+] prion propagation.Citation1 Two recent studies are in agreement with these findings. Regarding the role of the C-terminal domains of Sis1, Masison and colleagues recently published an investigation into the chaperone requirements of single variants of [PSI+], [RNQ+] and [URE3] and found previously undetected abilities of the C-terminal domains of both Sis1 and Ydj1 to direct Hsp70 and Hsp90 functions, underscoring the importance of these regions to aid in prion propagation.Citation19 Likewise, Stein and True also recently examined the role of [PSI+] prion variation in determining Sis1 domain requirements and, though they observed greater sensitivity toward Sis1 mutation by both of the weak variants they examined, they arrived at the same general conclusion that prion variation, particularly the distinction between weak and strong variants of [PSI+], greatly affected the requirements for Sis1 domains as our data discussed so far also suggests.Citation26
Partial Conservation of Sis1 Functions in the Human Homolog Hdj1/DNAJB1
One advantage of studying the prion-chaperone machinery in yeast is that specific functions that may be conserved in orthologous proteins can be easily tested using complementation assays. Of particular interest to us is the human homolog of Sis1, called Hdj1 or DNAJB1, which can not only rescue cell viability in a sis1-Δ strain but, incredibly, propagate the prion [RNQ+] in yeast.Citation27,28 As with Sis1, the minimum functional regions required for both these functions were narrowed down to the J-domain and glycine-rich regions.Citation28 Like [RNQ+], we found that Hdj1 can also propagate 4 different strong variants of [PSI+].Citation1 In sharp contrast, neither weak variant we examined was able to propagate, despite our reproduction of all experiments in 2 yeast genetic backgrounds, again illustrating that Hsp40 requirements are strongly affected by amyloid polymorphism.Citation1 This discovery that strong but not weak variants of [PSI+] can be propagated by Hdj1 was simultaneously reported by the True laboratory,Citation26 and yet these results conflict with an earlier report that Hdj1 was unable to propagate a specific variant of [PSI+].Citation20 Because the variant used in that study is also strong, it is likely that the discrepancy between our 3 reports is either an issue of protein expression levels or possibly an uncharacterized polymorphism in the genetic background used. Notably, both we and the True lab noted increased polymer sizes resolved on SDDAGE gels in cells expressing only Hdj1, indicative of a [PSI+] fragmentation defect in these cells.Citation1,26 These observations, together with the size increases observed with Sis1 truncation/deletion mutants described above, further support the notion that the primary role of Sis1/Hdj1 is in [PSI+] fragmentation as originally suggested by Higurashi et al. on the basis of kinetics data and SDDAGE analysis,Citation10 and also indicate that Hdj1 is somehow deficient in that function, but not deficient enough to cause loss of strong variants of [PSI+].
Sis1 is also required for the curing of [PSI+] by overexpression of Hsp104,Citation20 though the mechanism by which curing occurs is debated.Citation9,29,30 Our recent findings raise the question of whether Hdj1 has maintained this functionality of Sis1 as well, a question that we were unable to address in our original investigation but will address here. We examined the effects of Hsp104 overexpression in W303 strains expressing Hdj1 in place of Sis1 (). Strikingly, while the plasmid pRS426-GPD-HSP104 produces Hsp104 levels sufficiently high to efficiently cure all variants of [PSI+] that we have examined to date, when moved into these cells, all 3 strong [PSI+] variants we examined were completely protected from Hsp104-dependent curing (). As with other Sis1/Hdj1 experiments, we sought to confirm that these results were not due to an uncharacterized polymorphism of the W303 genetic background and so we repeated these experiments with “isoprionic” strains of the 74D-694 genetic background bearing the same 3 strong [PSI+] variants. In this case, the results were highly surprising: while 2 variants, [PSI+]STR and [PSI+]VH, were fully resistant to curing, 74D-694 cells bearing [PSI+]Sc4 were cured consistently across multiple repetitions of the experiment (overall 39 out of 40 transformants examined over 4 experimental replicates, ). To confirm that the observed color phenotypes really correspond to the maintenance or loss of the prion per se, and not merely an alteration of the phenotype, we subjected Hsp104 overexpressing cells from both backgrounds to analysis by SDDAGE. Indeed, only the [PSI+]Sc4 variant from the 74D-694 genetic background shows loss of SDS-resistant aggregates (). Finally, we ruled out both the accidental co-expression of Sis1, or a lack of Hsp104 overexpression, as possible confounding factors affecting our results; as expected, Sis1 expression was absent from all strains tested () and Hsp104 levels are highly elevated, relative to cells prior to plasmid transformation, to a level that efficiently cures all 3 [PSI+] variants in both backgrounds in the presence of Sis1 (). Notably, the level of Hsp104 in the [PSI+]Sc4 74D-694 strain is slightly, but reproducibly, higher than in the strains maintaining other [PSI+] variants and so we cannot presently rule out whether the curing of this variant, specifically, and only in this genetic background, is due to this slight difference in Hsp104 levels. Regardless, the completely different experimental outcome observed for this one combination of both prion variant and yeast genetic background underscores the importance of reproducing experiments in multiple genetic backgrounds whenever feasible.
Figure 2. Hdj1 is deficient in replacing Sis1 in the curing of [PSI+] by Hsp104 overexpression. (A & B) [PSI+] cells of the W303 (panel A) or 74D-694 (panel B) genetic backgrounds bearing strong [PSI+] variants ([PSI+]STR, [PSI+]Sc4, or [PSI+]VH) and expressing Hdj1 in place of Sis1 (left columns) were transformed by a plasmid overexpressing Hsp104 ([pRS426-GPD-HSP104]) that normally cures all variants of [PSI+] (right columns). Color phenotype assays are shown for representative transformants (n ≥ 10 for each variant) following passage on media selecting for the Hsp104 overexpression plasmid. (C) Maintenance or loss of [PSI+] in cells shown in panel B was also confirmed by semi-denaturing detergent agarose gel electrophoresis (SDDAGE). Detergent resistant Sup35 aggregates indicative of the presence of [PSI+] were resolved by SDDAGE and visualized by immunoblot analysis using an antibody specific for Sup35. Control [psi−] cells were included for comparison. (D & E) Protein expression levels in cells from the 74D-694 genetic background were measured for Sis1 (panel D) and Hsp104 (panel E). Cell extracts from isolates in panel B were subjected to immunoblot analysis using antibody specific for Sis1 or Hsp104. A band cross-reacting with each antibody is shown as a loading control. Control [psi−] cells were included for comparison. Sis1 and Sup35 antibodies and methods for SDDAGE, SDSPAGE, and immunoblotting are the same as described in Harris et al.Citation1
![Figure 2. Hdj1 is deficient in replacing Sis1 in the curing of [PSI+] by Hsp104 overexpression. (A & B) [PSI+] cells of the W303 (panel A) or 74D-694 (panel B) genetic backgrounds bearing strong [PSI+] variants ([PSI+]STR, [PSI+]Sc4, or [PSI+]VH) and expressing Hdj1 in place of Sis1 (left columns) were transformed by a plasmid overexpressing Hsp104 ([pRS426-GPD-HSP104]) that normally cures all variants of [PSI+] (right columns). Color phenotype assays are shown for representative transformants (n ≥ 10 for each variant) following passage on media selecting for the Hsp104 overexpression plasmid. (C) Maintenance or loss of [PSI+] in cells shown in panel B was also confirmed by semi-denaturing detergent agarose gel electrophoresis (SDDAGE). Detergent resistant Sup35 aggregates indicative of the presence of [PSI+] were resolved by SDDAGE and visualized by immunoblot analysis using an antibody specific for Sup35. Control [psi−] cells were included for comparison. (D & E) Protein expression levels in cells from the 74D-694 genetic background were measured for Sis1 (panel D) and Hsp104 (panel E). Cell extracts from isolates in panel B were subjected to immunoblot analysis using antibody specific for Sis1 or Hsp104. A band cross-reacting with each antibody is shown as a loading control. Control [psi−] cells were included for comparison. Sis1 and Sup35 antibodies and methods for SDDAGE, SDSPAGE, and immunoblotting are the same as described in Harris et al.Citation1](/cms/asset/99b68984-3cbd-4bc9-8845-0c8cefdb4f73/kprn_a_1020268_f0002_c.gif)
Despite the apparent variability of experimental outcomes, these new data, taken together, establish that Hdj1 is highly deficient in its ability to replace Sis1 in the curing of strong [PSI+] by Hsp104 overexpression. Curiously, a previous investigation reported that the homodimerization domain of Sis1 is critical for Hsp104-mediated curing of a single strong [PSI+] variant.Citation20 Since Hdj1 has a homodimerization domain, an immediate reason for the distinct inability of Hdj1 to fully complement Sis1 in this assay is not obvious. One possibility is that a critical aspect of Sis1 dimerization may be the formation of an internal cleft between monomers with specific geometry. Because Hdj1 is shorter than Sis1, it is possible that the Hdj1 dimer has a geometry that is largely incompatible with full participation in Hsp104-mediated curing, at least for most variants of [PSI+]. These observations further support the notion that Hdj1 has maintained some but not all functions of Sis1 which may be due, at least in part, to additional functional differentiation of Hsp40 isoforms in mammalian evolution; there are at least 41 J-proteins in humans compared to only 23 in yeast.Citation27 Case in point, we are unable to examine whether Hdj1 can replace Sis1 in the curing of weak [PSI+] variants by Hsp104 overexpression because, as noted above, these variants cannot be maintained by Hdj1.Citation1,26
As a final note about these new experimental results, while the mechanism by which Hsp104 overexpression cures [PSI+] is currently a matter of some debate,Citation9,29–31 our data provide new support for a model in which [PSI+] aggregates increase in size during Hsp104-mediated curing. Distinct size shifts toward higher molecular weight polymers were apparent in the lysates of [PSI+] cells overexpressing Hsp104 when compared to the same cells prior to transformation (). These shifts were highly reproducible across multiple replicates and so these data, observed here for the first time with an Hsp40 member that is deficient in providing the necessary function, corroborate prior observations that [PSI+] polymer size (as observed on SDDAGE gels) increases prior to curing by Hsp104 overexpression.Citation31,32
Two Prions have Mutually Exclusive Requirements for Sis1 Demonstrating Functional Plurality
In a previous investigation, one of us (JKH) and co-workers found that that [PSI+] is cured much slower than [RNQ+] upon repression of Sis1 expression and that both a strong and weak variant of [PSI+] could be propagated by Sis1ΔG/F, which cures [RNQ+].Citation10 Based on those observations, we previously postulated a ‘stringency’ model for Sis1 activity where these 2 prions require a presumably singular function of Sis1, but to different extents.Citation10 A presumption in that model is that the Sis1ΔG/F construct, which does not support [RNQ+], must be fundamentally less active than Sis1–121, which supports [RNQ+]. The results from our most recent investigation negate this stringency model as we found that these 2 Sis1 constructs reciprocally supported a variant of [RNQ+] and weak variants of [PSI+].Citation1 To rule out any issues involving small experimental variations, or differences in specific cell isolates, we conducted shuffling experiments to further clarify this point by using cells carrying 2 prions simultaneously ([RNQ+] and [PSI+]Sc37). We demonstrated that either prion could be selected for, at the expense of the other, depending upon which construct of Sis1 was shuffled in.Citation1 Notably, this experiment verified that the domain requirements for the 2 prions are mutually exclusive and, more importantly, demonstrated for the first time that Sis1 must accomplish at least 2 biochemically distinct functions in prion maintenance that are differentially required by distinct prion variants.Citation1 Stein and True recently found similar results when examining 5 variants of [RNQ+] as the domain requirements for Sis1 were highly variable, and in the case of 2 variants, fully distinct from one another.Citation26 However, despite multiple investigations into the Sis1 domain requirements of [PSI+], still no construct has been identified that separates cell viability from strong [PSI+] maintenance.Citation1,19,20,26 Together all data support the notion that strong [PSI+] variants require minimal functional contribution from Sis1 to fragment aggregates at a rate that allows stable propagation, while, as noted above, Sis1 domain requirements for weak [PSI+] variants and [RNQ+] variants vary widely and in some cases are completely distinct. The emerging picture is one where multiple domains of Sis1 can coordinate in alternate ways to maintain specific prions and prion variants. Though not withstanding additional possibilities, these divergent Sis1 functions are most likely either alternate means of regulating Hsp70 activity to accomplish prion fragmentation, or alternate modes of binding and recruiting Hsp70 to aggregates. Previous work, also from the True lab, is consistent with the latter hypothesis, suggesting that [RNQ+] variants present distinct surfaces for chaperone binding by combining different sets of non-adjacent amyloidogenic regions into the amyloid cores of different variants.Citation21,25 Our data,Citation1 and that of others,Citation33 also support a similar notion that [PSI+] variants may likewise present distinct chaperone binding surfaces which affect their ability to interact with, and therefore become remodeled by, the Hsp40/70/104 chaperone machinery in a Sis1-dependent manner. Given the collective data from our 2 laboratories, we favor the latter model where combinations of Sis1 domains mediate alternate modes of binding to amyloids and thereby recruiting and activating Hsp70 at amyloid surfaces, but certainly additional experimentation is needed to make any definitive determinations.
Future Directions: The Chaperone Requirements of Most Prions Remain Undetermined
Sis1 is absolutely required for the propagation of at least 4 prions,Citation10,12,13 and yet these prions exhibit enormous differences in requirements for Sis1 activity,Citation1,18–20,24–26 demonstrating a diversity of functionality that has only been revealed due to the exploration of chaperone requirements for multiple amyloid structures, i.e., multiple prions and prion variants. Importantly, of the 10 identified amyloid-forming prions in yeast, investigations into the role of Hsp70 and its co-chaperones in prion propagation have so far been limited to just 4: [PSI+], [RNQ+], [URE3], and [SWI+]. As such, the potential roles of J-proteins, nucleotide exchange factors, or the 4 isoforms of Ssa have not been systematically investigated for [MOT3+], [ISP+], [OCT+], [NSI+], [MOD+], and [NUP100+]. Given the apparent, profound diversity of prion structures and chaperone requirements revealed so far, it is likely that other modes of chaperone-dependent or perhaps chaperone-independent prion transmission may exist. Indeed, the amyloid forming prion [ISP+] has been reported to propagate independently of Hsp104 activity, indicating that its propagation may be either entirely chaperone independent or rely upon other chaperone systems which remain to be identified.Citation34 Critically, the full spectrum of amyloid diversity in vivo, and thus the gamut of chaperone functional complexity, will remain obscured until more prion•chaperone interactions can be investigated.
Disclosure of Potential Conflicts of Interest
No potential conflicts of interest were disclosed.
Funding
This work was supported by the Lafayette College Chemistry Department, the EXCEL research scholarship program, and by the National Institute of General Medical Sciences of the National Institutes of Health under Award Number R15GM110606. The content is solely the responsibility of the authors and does not necessarily represent the official views of the National Institutes of Health. The funders had no role in study design, data collection and analysis, decision to publish, or preparation of the manuscript.
REFERENCES
- Harris JM, Nguyen PP, Patel MJ, Sporn ZA, Hines JK. Functional diversification of hsp40: distinct j-protein functional requirements for two prions allow for chaperone-dependent prion selection. PLoS Genet 2014; 10:e1004510; PMID:25058638; http://dx.doi.org/10.1371/journal.pgen.1004510
- Kondrashkina AM, Antonets KS, Galkin AP, Nizhnikov AA. Prion-like determinant [NSI+] decreases the expression of the SUP45 gene in saccharomyces cerevisiae. Mol Biol 2014; 48:688-93; http://dx.doi.org/10.1134/S0026893314050069
- Wickner RB, Edskes HK, Kryndushkin D, McGlinchey R, Bateman D, Kelly A. Prion diseases of yeast: amyloid structure and biology. Semin Cell Dev Biol 2011; 22(5):469-75; PMID:21345375; http://dx.doi.org/10.1016/j.semcdb.2011.02.021
- Liebman SW, Chernoff YO. Prions in yeast. Genetics 2012; 191:1041-72; PMID:22879407; http://dx.doi.org/10.1534/genetics.111.137760
- Cox B, Ness F, Tuite M. Analysis of the generation and segregation of propagons: entities that propagate the [PSI+] prion in yeast. Genetics 2003; 165:23-33; PMID:14504215
- Winkler J, Tyedmers J, Bukau B, Mogk A. Chaperone networks in protein disaggregation and prion propagation. J Struct Biol 2012; 179:152-60; PMID:22580344; http://dx.doi.org/10.1016/j.jsb.2012.05.002
- Satpute-Krishnan P, Langseth SX, Serio TR. Hsp104-dependent remodeling of prion complexes mediates protein-only inheritance. PLoS Biol 2007; 5:e24; PMID:17253904; http://dx.doi.org/10.1371/journal.pbio.0050024
- Chernoff YO, Lindquist SL, Ono B, Inge-Vechtomov SG, Liebman SW. Role of the chaperone protein Hsp104 in propagation of the yeast prion-like factor [psi+]. Science 1995; 268:880-4; PMID:7754373; http://dx.doi.org/10.1126/science.7754373
- Winkler J, Tyedmers J, Bukau B, Mogk A. Hsp70 targets Hsp100 chaperones to substrates for protein disaggregation and prion fragmentation. J Cell Biol 2012; 198:387-404; PMID:22869599; http://dx.doi.org/10.1083/jcb.201201074
- Higurashi T, Hines JK, Sahi C, Aron R, Craig EA. Specificity of the J-protein Sis1 in the propagation of 3 yeast prions. Proc Natl Acad Sci U S A 2008; 105:16596-601; PMID:18955697; http://dx.doi.org/10.1073/pnas.0808934105
- Rosenzweig R, Moradi S, Zarrine-Afsar A, Glover JR, Kay LE. Unraveling the mechanism of protein disaggregation through a ClpB-DnaK interaction. Science 2013; 339:1080-3; PMID:23393091; http://dx.doi.org/10.1126/science.1233066
- Sondheimer N, Lopez N, Craig EA, Lindquist S. The role of Sis1 in the maintenance of the [RNQ+] prion. Embo J 2001; 20:2435-42; PMID:11350932; http://dx.doi.org/10.1093/emboj/20.10.2435
- Hines JK, Li X, Du Z, Higurashi T, Li L, Craig EA. [SWI], the prion formed by the chromatin remodeling factor Swi1, is highly sensitive to alterations in Hsp70 chaperone system activity. PLoS Genet 2011; 7:e1001309; PMID:21379326; http://dx.doi.org/10.1371/journal.pgen.1001309
- Reidy M, Miot M, Masison DC. Prokaryotic chaperones support yeast prions and thermotolerance and define disaggregation machinery interactions. Genetics 2012; 192:185-93; PMID:22732191; http://dx.doi.org/10.1534/genetics.112.142307
- Park SH, Kukushkin Y, Gupta R, Chen T, Konagai A, Hipp MS, Hayer-Hartl M, Hartl FU. PolyQ proteins interfere with nuclear degradation of cytosolic proteins by sequestering the Sis1p chaperone. Cell 2013; 154:134-45; PMID:23791384; http://dx.doi.org/10.1016/j.cell.2013.06.003
- Yang Z, Hong JY, Derkatch IL, Liebman SW. Heterologous gln/asn-rich proteins impede the propagation of yeast prions by altering chaperone availability. PLoS Genet 2013; 9:e1003236; PMID:23358669; http://dx.doi.org/10.1371/journal.pgen.1003236
- Tipton KA, Verges KJ, Weissman JS. In vivo monitoring of the prion replication cycle reveals a critical role for Sis1 in delivering substrates to Hsp104. Mol Cell 2008; 32:584-91; PMID:19026788; http://dx.doi.org/10.1016/j.molcel.2008.11.003
- Hines JK, Craig EA. The sensitive [SWI (+)] prion: new perspectives on yeast prion diversity. Prion 2011; 5:164-8; PMID:21811098; http://dx.doi.org/10.4161/pri.5.3.16895
- Reidy M, Sharma R, Shastry S, Roberts BL, Albino-Flores I, Wickner S, Masison DC. Hsp40s specify functions of Hsp104 and Hsp90 protein chaperone machines. PLoS Genet 2014; 10:e1004720; PMID:25329162; http://dx.doi.org/10.1371/journal.pgen.1004720
- Kirkland PA, Reidy M, Masison DC. Functions of yeast Hsp40 chaperone Sis1p dispensable for prion propagation but important for prion curing and protection from prion toxicity. Genetics 2011; 188:565-77; PMID:21555396; http://dx.doi.org/10.1534/genetics.111.129460
- Stein KC, True HL. Prion strains and amyloid polymorphism influence phenotypic variation. PLoS Pathog 2014; 10:e1004328; PMID:25188330; http://dx.doi.org/10.1371/journal.ppat.1004328
- Derkatch IL, Chernoff YO, Kushnirov VV, Inge-Vechtomov SG, Liebman SW. Genesis and variability of [PSI] prion factors in Saccharomyces cerevisiae. Genetics 1996; 144:1375-86; PMID:8978027
- Prusiner SB. Biology and genetics of prions causing neurodegeneration. Annu Rev Genet 2013; 47:601-23; PMID:24274755; http://dx.doi.org/10.1146/annurev-genet-110711-155524
- Hines JK, Higurashi T, Srinivasan M, Craig EA. Influence of prion variant and yeast strain variation on prion-molecular chaperone requirements. Prion 2011; 5:238-44; PMID:22156732; http://dx.doi.org/10.4161/pri.17818
- Stein KC, True HL. Extensive diversity of prion strains is defined by differential chaperone interactions and distinct amyloidogenic regions. PLoS Genet 2014; 10:e1004337; PMID:24811344; http://dx.doi.org/10.1371/journal.pgen.1004337
- Stein KC, True HL. Structural variants of yeast prions show conformer-specific requirements for chaperone activity. Mol Microbiol 2014; 93:1156-71; PMID:25060529
- Kampinga HH, Craig EA. The HSP70 chaperone machinery: J proteins as drivers of functional specificity. Nat Rev Mol Cell Biol 2010; 11:579-92; PMID:20651708; http://dx.doi.org/10.1038/nrm2941
- Lopez N, Aron R, Craig EA. Specificity of class II Hsp40 Sis1 in maintenance of yeast prion [RNQ+]. Mol Biol Cell 2003; 14:1172-81; PMID:12631732; http://dx.doi.org/10.1091/mbc.E02-09-0593
- Park YN, Zhao X, Yim YI, Todor H, Ellerbrock R, Reidy M, Eisenberg E, Masison DC, Greene LE. Hsp104 overexpression cures Saccharomyces cerevisiae [PSI+] by causing dissolution of the prion seeds. Eukaryot Cell 2014; 13:635-47; PMID:24632242; http://dx.doi.org/10.1128/EC.00300-13
- Helsen CW, Glover JR. A new perspective on Hsp104-mediated propagation and curing of the yeast prion [PSI (+) ]. Prion 2012; 6:234-9; PMID:22561166; http://dx.doi.org/10.4161/pri.19913
- Klaips CL, Hochstrasser ML, Langlois CR, Serio TR. Spatial quality control bypasses cell-based limitations on proteostasis to promote prion curing. Elife 2014; 3:e04288; PMID:25490068; http://dx.doi.org/10.7554/eLife.04288
- Kryndushkin DS, Alexandrov IM, Ter-Avanesyan MD, Kushnirov VV. Yeast [PSI+] prion aggregates are formed by small Sup35 polymers fragmented by Hsp104. J Biol Chem 2003; 278:49636-43; PMID:14507919; http://dx.doi.org/10.1074/jbc.M307996200
- Frederick KK, Debelouchina GT, Kayatekin C, Dorminy T, Jacavone AC, Griffin RG, Lindquist S. Distinct prion strains are defined by amyloid core structure and chaperone binding site dynamics. Chem Biol 2014; 21:295-305; PMID:24485763; http://dx.doi.org/10.1016/j.chembiol.2013.12.013
- Radchenko E, Rogoza T, Khokhrina M, Drozdova P, Mironova L. SUP35 expression is enhanced in yeast containing [ISP+], a prion form of the transcriptional regulator Sfp1. Prion 2011; 5:317-22; PMID:22156729; http://dx.doi.org/10.4161/pri.18426