Abstract
Transmissible spongiform encephalopathies (TSEs) are a group of neurodegenerative diseases caused by the misfolding of the cellular prion protein to an infectious form PrPSc. The intercellular transfer of PrPSc is a question of immediate interest as the cell-to-cell movement of the infectious particle causes the inexorable propagation of disease. We have previously identified tunneling nanotubes (TNTs) as one mechanism by which PrPSc can move between cells. Here we investigate further the details of this mechanism and show that PrPSc travels within TNTs in endolysosomal vesicles. Additionally we show that prion infection of CAD cells increases both the number of TNTs and intercellular transfer of membranous vesicles, thereby possibly playing an active role in its own intercellular transfer via TNTs.
Abbreviations
Ab | = | antibody |
PrPC | = | cellular prion protein |
CFP | = | cyan fluorescent protein |
ERC | = | endocytic recycling compartment |
ER | = | endoplasmic reticulum |
GFP | = | green fluorescent protein |
PM | = | plasma membrane |
RFP | = | red fluorescent protein |
PrPSc | = | scrapie prion protein |
TSEs | = | transmissible spongiform encephalopathies |
TNTs | = | tunneling nanotubes |
Introduction
The event of conversion of the cellular prion protein PrPC to its misfolded conformer PrPSc is the basis for a group of neurodegenerative diseases known as transmissible spongiform encephalopathies. Once formed, the pathogenic, infectious PrPSc is capable of recruitment and conversion of naïve PrPC molecules, resulting in the formation of oligomers and larger aggregates. These aggregates are both intracellular and externally deposited in neuronal plaquesCitation1 that are one of the hallmarks of prion neuropathology. This eventually results, by mechanisms that are not entirely clear, in inevitable and fatal neurodegeneration.Citation2
The intercellular transmission of the infectious protein has been demonstrated between different cell types, including between dendritic cells and neuronsCitation3, with many proposed routes comprising axonal transport, exosomes and tunneling nanotubes (TNTs).Citation4,5 In particular, we have previously demonstrated that PrPSc aggregates can transfer between neuronal cells using tunneling nanotubes.Citation5 TNTs are thin, actin-containing intercellular tubes, that may act as conduits that allow the passage of organelles, endocytic vesicles, protein aggregates and viruses between cells.Citation6,7, Citation8,9
The mechanism by which PrPSc transfers through TNTs has not been addressed. Because PrP is a GPI-anchored protein targeted to the outer leaflet of the plasma membrane we envision 2 possibilities by which PrPSc could transfer though TNTs: by diffusion along the surface of the TNT, consistent with its localization to the plasma membrane or within the tube itself inside specific organelles or vesicles. A recent study reported that PrPSc could form string-like aggregates on the plasma membrane surface, and the authors detect PrPSc strings on intercellular bridgesCitation10 thus suggesting that diffusion along the cell membrane surface might represent one method of transfer. On the other hand, numerous studies show that, in infected cells, a large percentage of PrPSc is found internally, along the endocytic and secretory pathways.Citation1,11,12 Furthermore, we have demonstrated that the endocytic recycling compartment (ERC) is a major intracellular site of PrPSc conversion.Citation13 Thus, a likely possibility is that the TNT-mediated prion transfer occurs using active transport of prion–containing vesicles.Citation14
In this study, we investigated the mechanism of PrPSc transfer through TNTs within intracellular organelles and vesicles. Using a chronically infected neuronal cell line (ScCAD),Citation5,13 we demonstrate that endocytic organelles are present within TNTs and PrPSc is colocalized with these vesicles, suggesting that the active transport of PrPSc-containing vesicles in TNTs indeed occurs. Additionally, we demonstrate that PrPSc increases both number of TNTs and transfer efficiency.
Organelles of the Recycling Pathway Enter TNTs
In order to understand by which mechanisms PrPSc is transferred via TNTs, we first determined which subcellular compartments could enter the TNTs formed between neuronal CAD cells. To this end we either used immunofluorescence to detect endogenous proteins or transfection with GFP-tagged protein markers of different subcellular compartments. We identified several vesicular markers inside tunneling nanotube-like structures formed between CAD, notably those for the early endosomes (EEA1), endosomal recycling compartments (ERC) (Rab11 and VAMP3) as well as lysosomes (LAMP1) (). On the other hand we did not find markers of the cis, medial or trans-Golgi nor of the post-Golgi carriers within TNT-like structures. One possible explanation for this finding is that as early endosomes and the ERC are involved in recycling of proteins between the intracellular environment and plasma membrane, their functions and position might make them more accessible to entering the membrane protrusions caused by TNT formation. However it should be noted that both the ER and Golgi have been reported inside TNT-like structures in cells of different originCitation15,16 therefore this could be also cell type specific.
TABLE 1. Organelles found within TNTs in CAD cell lines
PrPSc Colocalizes with Endosomal Compartments Inside TNTs
To determine whether PrPSc was colocalized with any of the subcellular compartments found in TNTs, we used ScCAD, a CAD cell line stably propagating infectious PrPSc.Citation5 We have previously characterized the subcellular compartmentalization of PrPC and PrPSc in this cell line and showed that while PrPC was enriched at the plasma membrane (PM) and in the Golgi, PrPSc was found predominantly in the endocytic pathway, in early endosomes and late endosomes/lysosomes and specifically enriched in recycling endosomes (ERC).Citation13 Thus, the infectious protein may well be transported in these organelles along TNTs. After guanidium thiocyanate denaturation to reveal PrPSc epitopes (), we performed immunofluorescence for the different organelle markers described above, and analyzed the co-localization with PrPSc inside the TNT lumen. As shown in , we observed ∼28% of PrPSc within TNT-like structures colocalized with EEA1 while approximately 45% of PrPSc co-localized with Vamp3. The co-localization of PrPSc with markers for early endosomes and early recycling compartments is consistent with our previous work showing that the ERC is a site for conversion of PrPC to PrPSc and does suggest a continual recycling of surface PrP wherein conversion might be occurring, thus maintaining the persistent infection of the ScCAD.Citation13 It has been proposed that the ERC is a major storage organelle for cholesterolCitation17 and since PrP is GPI-anchored, and found predominantly in cholesterol-rich lipid raftsCitation18 the redistribution to the ERC environment together with its cellular positioning might provide an explanation for the higher observed colocalization within TNTs. In addition, spreading PrPSc within the ERC compartment where conversion occurs would provide the uninfected recipient cells with both the infectious seed and the propitious environment (the machinery for conversion) and thereby promote propagation of PrPSc through the recycling of PrPC through this organelle.
Figure 1. Co-localization between PrPSc and different sub-cellular organelles in TNT-like structures of ScCAD cells. (A) Representative immunofluorescence of PrP from CAD and ScCAD after guanidium thiocyanate denaturation. Images were acquired at exactly the same acquisition parameters. PrPSc puncta are clearly revealed by enhanced epitope detection after denaturation while PrPC signal is diminished. Scale bar: 10 μm. (B) Co-immunofluorescence for different organelle markers and PrPSc was performed after guanidium thiocyanate treatment to determine colocalization. Scale bar: 5 μm. (C) Quantification of the colocalization between PrPSc and the different organelle markers in TNT structures. The percentage of PrPSc particles co-localizing respectively with EEA1 (for early endosomes), Lamp1 (for lysosomes) and Vamp3 (ERC) was evaluated. (Mean + s.e.m).
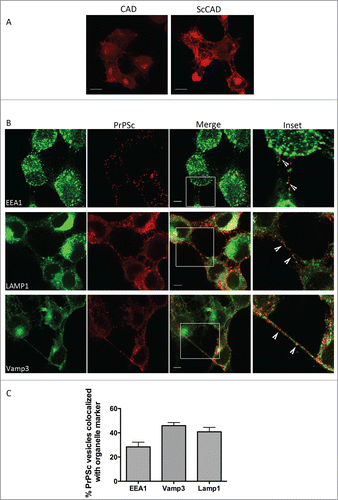
We also observed about 40% of PrPSc with Lamp1-positive structures inside TNTs. As ScCAD do not undergo apoptosis upon scrapie infection, certain mechanisms must operate in order to maintain a balance between scrapie production and degradation that allows the stably infected cell line to continue without the heavy cell death observed in normal progression of the disease. Thus, the large percentage of PrPSc found within lysosomes is consistent with continual degradationCitation13,19 of the misfolded conformer. Interestingly, while average lysosome number per cell is similar between CAD and ScCAD, we note a small but significant increase in average lysosome size in ScCAD (Fig. S1). This could be consistent with increased protein degradation and lysosomal load.
Together, the data suggests that PrPSc can spread through TNTs in the recycling compartments wherein they are converted, the lysosome and, to a lesser extent, in the early endosome.
PrPSc Affects TNT Formation and Increases Intercellular Vesicle Transfer
We observed that the prion infected ScCAD cultures appeared to have considerably more intercellular TNT-like connections than CAD cells under our usual culture conditions (). We therefore asked if prion infection could influence TNT formation and in turn its intercellular transfer. Using ICY image analysis software to identify TNTs (see methods), we quantified the percentage of TNT-forming cells in ScCAD. As shown in , we recorded an ∼20% increase in the percentage of TNT-forming cells as compared to control CAD cultures. In order to address TNT functionality (i.e, the ability to allow transfer of vesicles between connected cells), we then investigated whether the increase in TNT-like structures also corresponded to an increased transfer of DiD-labeled vesicles from ScCAD cells to acceptor CAD populations. We have previously set up this type of transfer assay using FACS.Citation5 However, due to the nature of the infectious PrPSc particles FACS assays were not possible as the disinfection procedures were potentially damaging to the instrument. Therefore we developed a microscopy-based quantitative assay of the DiD-labeled vesicle transfer (see methods). As shown in , there was a significant increase in the percentage of acceptor cells which had received DiD-labeled vesicles after an overnight co-culture with donor ScCAD (109 out of 390 cells) when compared to the control experiment with CAD donors (66/403). Thus prion infection results in increased TNT formation and intercellular vesicle transfer.
Figure 2. Effect of PrPSc and PrPC on TNT formation and intercellular vesicle transfer. (A) Representative image of TNT formation in uninfected CAD cells (top panel) and chronically prion-infected ScCAD (bottom panel). In green, the plasma membrane labeled with WGA488 and red, the nucleus. (B) Quantification of TNT formation in these 2 cell lines show that prion infection increases TNT forming cells by ˜20% (p = 0.0393, one tailed t-test respectively). (C) Prion infection increases DiD-labeled vesicle transfer to mCherry-H2B-labeled acceptor cells (p= 0.0429). Medium CAD and medium ScCAD respectively indicate mCherry-H2B-labeled CAD cells that had been treated with medium from either DiD-labeled CAD or ScCAD as described in methods. Transfer does not seem to be secretion-mediated. (D) Representative image of TNT formation from cells overexpressing either GFP-vector (top panel) or GFP- PrPC (bottom panel). In green: GFP or GFP- PrPC respectively; in red: the plasma membrane labeled with WGA-546 and H2B-mCherry-labeled nuclei of acceptor cells. (E) Quantification of percentage of TNT forming cells in CAD overexpressing GFP or GFP- PrPC. GFP-PrP overexpression increases TNT forming cells (p = 0.05). (F) Quantification of the percentage of acceptor cells which contain transferred DiD-labeled vesicles after overnight co-culture shows no significant difference between GFP- or GFP- PrPC expressing cells. Scale bars, 10μm. For all graphs, the total number of cells counted (n) over 3 independent experiments is given on each respective column.
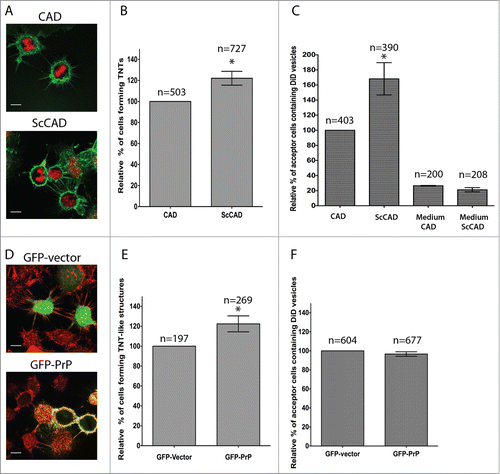
PrPC Levels Affect TNT Formation
PrPC has been shown to regulate filopodia and focal adhesion formation in different cell types, including mouse neuronal cells N2A and Drosophila S2 cells.Citation20 TNTs are distinguished from filopodia by their ability to form without the need for substrate adhesion. Current consensus defines TNTs as membranous actin-containing structures that do not touch the substratum. Although the mechanisms of their formation do appear to be different from filopodia, TNTs may however, arise from filopodia-like structures.Citation9,21,Citation22 We therefore addressed whether overexpression of PrPC also affected TNT formation, and performed similar TNT-counting and transfer experiments after overexpression of GFP-PrPC.
We transfected CAD cells with GFP-PrPC and quantified the number of transfected cells that produced TNTs. As shown in , the percentage of cells connected by TNT-like structures was increased in GFP-PrPC-CAD compared to the control cells transfected with GFP (GFP-CAD). Next we used the microscopy-based vesicle transfer assay to quantify the percentage of transfer of DiD-labeled vesicles from either GFP- or GFP- PrPC-transfected donor populations to acceptor cells that were labeled with H2B-mCherry to differentiate them from the donor cells. Surprisingly, however, the increase in TNT formation did not correspond to increased vesicle transfer from GFP-PrPC-CAD to untransfected cells (). We also performed the transfer assay using FACS that we had previously set upCitation5 and with this method we obtained the same results (Fig. S2), thus validating the microscopy transfer assay.
The results with PrPC were striking, as it appeared to uncouple TNT formation from vesicle transfer. However, because PrPC has been shown to increase filopodiaCitation20 we cannot exclude the possibility that the TNT-like structures that we observe following PrP overexpression are not veritable or fully developed TNTs, but dorsal filopodia connecting distant cells. While TNTs in CAD have been shown to be quite diverse in structure – displaying great heterogeneity in terms of both length (ranging from ∼10–80 μm) and diameter (from less than 186 nm to more than 800 nm)5, they can also arise from a subset of dorsal filopodia.Citation21 Thus the structures we observe might be an intermediate stage of TNTs forming from such structures. On the other hand, it might be also possible that the formation of more TNTs does not lead automatically to an increase in vesicle transfer, as this is not a simple diffusion mechanism but involves the action of molecular motors and very possibly, gating mechanisms. Because very little is known about the mechanism or regulation of vesicle transfer inside TNTs this issue should be further assessed.
We demonstrate here for the first time that prion trafficking through TNTs can occur by transport in endosomal vesicles. The work also provides for the first time experimental evidence to support the modeling study by Kuznetsov et al.Citation23 which predicted that prion transfer through TNTs was more likely to occur via active transport of vesicles than through passive diffusion on the plasma membrane. Interestingly, Rouvinski et al.Citation10 have shown PrPSc strings on the surface of intercellular bridges between ScGT1 cells and shown that on the cell body they have a slow speed of diffusion (0–3 μm/5 min). We do not rule out the possibility that diffusion along the plasma membrane may also contribute to intercellular prion transfer through TNTs, however we have not observed this phenomenon in CAD cells. As TNTs are highly dynamic structures that form and break quickly, often in a matter of minutes,Citation5 it seems likely that directed transport of prion in vesicles would be more efficient than slow-diffusing surface PrPSc aggregates.
Interestingly, the results also suggest that the accumulation of PrPSc itself acts as an inducer of increased intercellular vesicle transfer and may open new avenues in the study of TNT formation.
The data indicate that PrPSc accumulation but not PrPC influences functional TNT formation, however the basis for this difference is not clear. This does not appear to be a question of difference in the proportion of TNTs formed, since there is not a significant difference between the percentage of TNT-forming cells in ScCAD or GFP- PrPC-CAD. One possibility is the role of cholesterol; prion-infected cells are shown to have increased cholesterol levelsCitation24 and cholesterol has been implicated in TNT formation: cholesterol depletion has been shown to cause retraction of TNTs.Citation25 Additionally, cholesterol is a major constituent of lipid raftsCitation26 and affects membrane curvature.Citation27 Lipid rafts often act as signaling platforms through which many processes can be regulated, including the formation of filopodia and PrPC has also been implicated in a number of signaling roles.Citation20,28 Thus, one possible explanation is that PrPSc accumulation causes a perturbation in cholesterol levels and redistribution as well, affecting signaling pathways involved in active transport through TNTs.
Prion infection is also known to increase ER stress and the unfolded protein response,Citation29,30 a multi-armed process that among other things causes the increased splicing of a transcription factor Xbp1. Xbp1s translocates to the nucleus where it induces the expression of genes involved in lipid biosynthesis.Citation31 Thus, ER stress and cholesterol accumulation might be linked.
It also seems reasonable to speculate that the subcellular distribution of the infectious versus the native protein might have a role together with cholesterol.Citation13,19,Citation32 Since PrPC is targeted primarily to the plasma membrane and PrPSc is predominantly internally distributed, this change could affect normal signaling or cytoskeletal movements as well. Certainly many reports suggest that normal physiological function is altered during prion infection. For example Uchiyama et al.Citation12, show that post-Golgi trafficking of other raft-associated membrane proteins is disturbed in prion-infected N2A cells. There is also increased signaling activity such as from Src kinases.Citation33 As noted above, there is also increased endocytic recycling. It is possible that increased endocytic recycling could result in the accumulation of signaling endosomes that might affect transfer. Alternatively, PrPC might regulate transfer in some way and this function is perturbed by the formation of the aggregate-prone PrPSc, which results in dysregulation of this effect.
Although these data clearly indicate that intracellular and intercellular trafficking are important in prion diseases, these pathways should be further explored to assess their specific contribution to prion propagation.
Methods
Cell Culture and Transfection
The mouse catecholaminergic neuronal cell line CAD and its chronically 139A prion-infected counterpart ScCAD were grown in Gibco's OptiMEM supplemented with 10% fetal bovine serum and 1% Penicillin-Streptomycin. ScCAD cell line was derived from a newly thawed CAD population. After freshly thawing CAD, cells were divided between 2 flasks and one flask was treated with 139A-infected brain homogenate. After 3–4 passages, the cells were considered to be chronically infected as they maintained infection upon subsequent passages. CAD and ScCAD were used at similar passage numbers (between 3–10) for experiments.
Cells were transiently transfected with Lipofectamine 2000 (Invitrogen) according to the manufacturer's instructions.
Detection of Organelles in TNTs
ScCAD cell lines were transfected with pGFP-Rab11 (ERC), pRFP-Rab6 (trans-Golgi) and pGFP-Vamp3 (ERC and early endosomes) and cultured for 24 hours before fixation with 4% PFA. To detect endogenous markers of organelles within TNTs, antibodies were used as described in .
Colocalization Studies
ScCAD cultures were plated on Ibidi™ dishes for 24 h. Cells were fixed with 4% PFA. After permeabilization with 0.1% TX-100, PrPSc epitopes were revealed by denaturation with 3M guanidium thiocyanate for 5 mins, before washing and detection with Sha31 antibody (mouse, SPIbio). Primary antibodies to detect markers of lysosomes (anti-Lamp1, rat), early endosomes (anti-EEA1, rabbit) and ERC (Anti-Vamp3, rabbit, Abcam) were used simultaneously with Sha31, followed by extensive washing with PBS. Secondary antibodies to Sha31 were conjugated to Alexa546 (red) and secondary antibodies to the organelle markers were coupled to Alexa488. Coverslips were mounted in Aquapolymount™. As colocalizations were performed on endosomal compartments of the order of 30–50 nm in size, which is usually below the confocal microscopy resolution limits (∼200 nm), we acquired images at stringent parameters as described below. However, the resolution limit necessarily prevents single vesicles (as opposed to 2 closely clustered vesicles) from being distinguished. 16-bit Z-stack images through the cell volume were acquired on a Zeiss LSM700 confocal microscope using a plan apochromat objective (63X oil, NA1.4) to prevent chromatic aberration. All images were acquired close to the Nyquist sampling rate in order to perform image deconvolution. This was necessary as the point spread function (PSF) of the fluorophores is distorted by the passage of light through the objective. This results in blurring of emitted light from very small points, such as from endosomes, which can affect the spatial resolution of vesicles, and affect colocalization analyses. Thus, to further improve resolution of the PSF and attempt to resolve the blurry out-of-focus light back as closely to its point of origin as possible, images were deconvolved using Huygens Essential software (Scientific Volume Imaging) using a theoretical PSF based on the LSM700 microscope parameters and the Classic Maximum Likelihood Estimation (CMLE) algorithm. Colocalization analysis was carried out on deconvolved images using an objects-based colocalization method in the ICY softwareCitation34 (http://icy.bioimageanalysis.org/). Object sizes were set at scale 2 (i.e 4–7 pixels), with an intensity threshold to minimize background signal and analyzed stack by stack to obtain a quasi 3-dimensional picture. Between 30–50 TNTs, each containing on average 3–5 vesicular structures, were analyzed per organelle marker.
TNT-Counting Experiments
CADs cells were plated on T25 flasks overnight and transfected separately the next day with either pGFP-PrP, pGFP-vector or pH2B-mcherry using Lipofectamine 2000. After 24 hours, 1 × 105 GFP-PrP or GFP-vector–transfected cells were co-cultured overnight with 1 × 105 cells transfected with H2B-mcherry. The cells were then fixed with fixative solution 1 (2% PFA, 0.05% glutaraldehyde and 0.2 M HEPES in PBS) for 20 minutes at room temperature, followed by a second 20 minutes fixation with fixative solution 2 (4% PFA and 0.2 M HEPES in PBS). The cells were gently washed with PBS and labeled with WGA-Rhodamine to label the plasma membrane and thereby mark the TNT membrane (1:300 in PBS) for 20 minutes at room temperature, washed and sealed with Aqua-Polymount (Polysciences, Inc.). Image stacks covering the whole cellular volume were acquired using a LSM700 confocal microscope (40X oil plan apochromat objective). To evaluate the number of TNT-connected cells, manual analysis was performed and only the number of GFP- or GFP-PrP-transfected cells which possessed TNTs were counted. As previously described in CAD cellsCitation5 intercellular connections were considered to be TNTs if they contained actin and were WGA-labeled tubes connecting 2 distant cells without touching the substratum, i.e., occuring in the middle of the cellular volume. By counting only connections in the middle to upper stacks of the cell and not at the base, we eliminate the possibility of counting filopodial extensions. Average length (ranging from ∼10–80 μm) and diameter (from less than 186 nm to more than 800 nm).Citation5 Each experiment was performed at least in triplicate. Image analyses of raw data, such as Z-projections, were obtained using ICY software. Similar experiments were carried out with the ScCAD; here, 1 × 105 ScCAD were co-cultured overnight with pH2B-mCherry-CAD cells and fixation and TNT counting were carried out as described above.
DiD Vesicle Transfer Experiments
Microscopic transfer assay
GFP-PrP or GFP-vector transfected cells (donor) were incubated with vybrant DiD cell-labeling solution (1:3000 in complete medium) at 37°C for 1 hour to label vesicle membranes, washed with 0.01% trypsine 3 times to remove unbound dye. 1 × 105 of the donor cells were co-cultured with 1 × 105 H2B-mcherry-CAD cells (acceptor population) for 24 hours, then fixed with 4% PFA. Image stacks covering the whole cellular volume were acquired using a confocal-microscope (Zeiss LSM700). To evaluate the number of acceptor cells which had received the labeled vesicles, Z-projections of different microscope fields were counted for the number of double-positive cells (i.e., H2B-mCherry and DiD-positive cells). ScCAD cell lines were also stained as above to act as the donor population and co-cultured with H2B-mCherry-CAD. A medium transfer control was performed to eliminate the possibility that DiD staining in the acceptor population resulted from secretion. Briefly, CAD or ScCAD donor cells were loaded with DiD as described above and washed. After 24 hours incubation, medium was collected from the respective cell type, spun down at 1000 rpm to remove floating cells and the supernatant was applied over mCherry-H2B-labeled cells. After overnight incubation cells were counted for the presence of DiD.
FACS transfer assay
Donor cells were transfected, loaded with DiD and co-cultured as described above. In this case acceptor cells were transfected with cyan fluorescent protein (CFP). Cells were washed after co-culture and passed through sterile 40 μm cell strainers to remove clumps. After fixation, cells were passed through a CyAn ADB Flow CytometryAnalyser (Dako cytomation, Beckman Coulter) to detect DiD and CFP. Data was analyzed using Kaluza Flow analysis software (Beckman Coulter).
Statistical Tests
The p value from Student's paired t-tests was used to evaluate the significance of all the quantifications presented.
DISCLOSURE OF POTENTIAL CONFLICTS OF INTEREST
No potential conflicts of interest were disclosed.
Supplemental_Material.doc
Download MS Word (157.5 KB)Acknowledgments
The authors thank all lab members for discussion.
Funding
SZ is supported by a PhD fellowship from the Chinese Scholarship Council. GSV is supported by fellowships from the Fondation Recherche Médicale and the Bourse Pasteur-Roux, Institut Pasteur. This work is supported by research grants from the European Commission FP7 PRIORITY contract number 222887, the MI CARNOT ICSA/PMI, grants from the region Ile-de-France (IDF DIM-MALINF 2013), Agence Nationale de la Recherche (ANR-14-JPCD-0002–01) and Equipe FRM (Fondation Recherche Medicale) 2014 (DEQ20140329557) to CZ.
REFERENCES
- Godsave SF, Wille H, Kujala P, Latawiec D, DeArmond SJ, Serban A, Prusiner SB, Peters PJ. Cryo-immunogold electron microscopy for prions: toward identification of a conversion site. J Neurosci 2008; 28(47):12489–99; PMID:19020041; http://dx.doi.org/10.1523/JNEUROSCI.4474-08.2008
- Aguzzi A, Falsig J. Prion propagation, toxicity and degradation. Nat Neurosci 2012; 15(7):936–9; PMID:22735515; http://dx.doi.org/10.1038/nn.3120
- Langevin C, Gousset K, Costanzo M, Richard-Le Goff O, Zurzolo C. Characterization of the role of dendritic cells in prion transfer to primary neurons. Biochem J 2010; 431(2):189–98; PMID:20670217; http://dx.doi.org/10.1042/BJ20100698
- Arellano-Anaya ZE, Huor A, Leblanc P, Lehmann S, Provansal M, Raposo G, Andréoletti O, Vilette D. Prion strains are differentially released through the exosomal pathway. Cell Mol Life Sci 2014; 72:1185–96; Epub ahead of print; PMID:25227242; http://dx.doi.org/10.1007/s00018-014-1735-8
- Gousset K, Schiff E, Langevin C, Marijanovic Z, Caputo A, Browman DT, Chenouard N, de Chaumont F, Martino A, Enninga J, et al. Prions hijack tunnelling nanotubes for intercellular spread. Nat Cell Biol 2009; 11(3):328–36; PMID:19198598; http://dx.doi.org/10.1038/ncb1841
- Rustom A, Saffrich R, Markovic I, Walther P, Gerdes HH. Nanotubular highways for intercellular organelle transport. Science 2004; 303(5660):1007–10; PMID:14963329; http://dx.doi.org/10.1126/science.1093133
- Gerdes HH, Carvalho RN. Intercellular transfer mediated by tunneling nanotubes. Curr Opin Cell Biol 2008; 20(4):470–5; PMID:18456488; http://dx.doi.org/10.1016/j.ceb.2008.03.005
- Sowinski S, Alakoskela JM, Jolly C, Davis DM. Optimized methods for imaging membrane nanotubes between T cells and trafficking of HIV-1. Methods 2011; 53(1):27–33; PMID:20382227; http://dx.doi.org/10.1016/j.ymeth.2010.04.002
- Abounit S, Zurzolo C. Wiring through tunneling nanotubes–from electrical signals to organelle transfer. J Cell Sci 2012; 125(Pt 5):1089–98; PMID:22399801; http://dx.doi.org/10.1242/jcs.083279
- Rouvinski A, Karniely S, Kounin M, Moussa S, Goldberg MD, Warburg G, Lyakhovetsky R, Papy-Garcia D, Kutzsche J, Korth C, et al. Live imaging of prions reveals nascent PrPSc in cell-surface, raft-associated amyloid strings and webs. J Cell Biol 2014; 204(3):423–41; PMID:24493590; http://dx.doi.org/10.1083/jcb.201308028
- Jeffrey M, McGovern G, Goodsir CM, Brown KL, Bruce ME. Sites of prion protein accumulation in scrapie-infected mouse spleen revealed by immuno-electron microscopy. J Pathol 2000 191(3):323–32; PMID:10878556; http://dx.doi.org/10.1002/1096-9896(200007)191:3%3c323::AID-PATH629%3e3.0.CO;2-Z
- Uchiyama K, Muramatsu N, Yano M, Usui T, Miyata H, Sakaguchi S. Prions disturb post-Golgi trafficking of membrane proteins. Nat Commun 2013; 4:1846; PMID:23673631; http://dx.doi.org/10.1038/ncomms2873
- Marijanovic Z, Caputo A, Campana V, Zurzolo C. Identification of an intracellular site of prion conversion. PLoS Pathog 2009; 5(5):e1000426; PMID:19424437; http://dx.doi.org/10.1371/journal.ppat.1000426
- Marzo L, Gousset K, Zurzolo C. Multifaceted roles of tunneling nanotubes in intercellular communication. Front Physiol 2012 Apr 10; 3:72; PMID:22514537; http://dx.doi.org/10.3389/fphys.2012.00072
- Wang Y, Cui J, Sun X, Zhang Y. Tunneling-nanotube development in astrocytes depends on p53 activation. Cell Death Differ 2011; 18(4):732–42; PMID:21113142; http://dx.doi.org/10.1038/cdd.2010.147
- Kadiu I, Gendelman HE. Human immunodeficiency virus type 1 endocytic trafficking through macrophage bridging conduits facilitates spread of infection. J Neuroimmune Pharmacol 2011 Dec; 6(4):658–75; ; http://dx.doi.org/10.1007/s11481-011-9298-z
- Hao M, Lin SX, Karylowski OJ, Wüstner D, McGraw TE, Maxfield FR. Vesicular and non-vesicular sterol transport in living cells. The endocytic recycling compartment is a major sterol storage organelle. J Biol Chem 2002; 277(1):609–17; PMID:11682487; http://dx.doi.org/10.1074/jbc.M108861200
- Sarnataro D, Campana V, Paladino S, Stornaiuolo M, Nitsch L, Zurzolo C. PrP(C) association with lipid rafts in the early secretory pathway stabilizes its cellular conformation. Mol Biol Cell 2004; 15:4031–42; PMID:15229281; http://dx.doi.org/10.1091/mbc.E03-05-0271
- Marzo L, Marijanovic Z, Browman D, Chamoun Z, Caputo A, Zurzolo C. 4-hydroxytamoxifen leads to PrPSc clearance by conveying both PrPC and PrPSc to lysosomes independently of autophagy. J Cell Sci 2013; 126(Pt 6):1345–54; PMID:23418355; http://dx.doi.org/10.1242/jcs.114801
- Schrock Y, Solis GP, Stuermer CA. Regulation of focal adhesion formation and filopodia extension by the cellular prion protein (PrPC). FEBS Lett 2009; 583(2):389–93; PMID:19116153; http://dx.doi.org/10.1016/j.febslet.2008.12.038
- Gousset K, Marzo L, Commere PH, Zurzolo C. Myo10 is a key regulator of TNT formation in neuronal cells. J Cell Sci 2013; 126(Pt 19):4424–35; PMID:23886947; http://dx.doi.org/10.1242/jcs.129239
- Bukoreshtliev NV, Wang X, Hodneland E, Gurke S, Barroso JF, Gerdes HH. Selective block of tunneling nanotube (TNT) formation inhibits intercellular organelle transfer between PC12 cells. FEBS Lett 2009; 583(9):1481–8; PMID:19345217; http://dx.doi.org/10.1016/j.febslet.2009.03.065
- Kuznetsov IA, Kuznetsov AV. A minimal model of prion transport through a tunneling nanotube. Nanotube Ther 2012; 1:3–11, ISSN (Online) 2299-1026; PMID:23477810; http://dx.doi.org/10.2478/nanoth-2012-0002
- Bach C, Gilch S, Rost R, Greenwood AD, Horsch M, Hajj GN, Brodesser S, Facius A, Schädler S, Sandhoff K, et al. Prion-induced activation of cholesterogenic gene expression by Srebp2 in neuronal cells. J Biol Chem 2009; 284(45):31260–9; PMID:19748890; http://dx.doi.org/10.1074/jbc.M109.004382
- Lokar M, Kabaso D, Resnik N, Sepčić K, Kralj-Iglič V, Veranič P, Zorec R, Iglič A. The role of cholesterol-sphingomyelin membrane nanodomains in the stability of intercellular membrane nanotubes. Int J Nanomedicine 2012; 7:1891–902; PMID:22605937
- Simons K, Ikonen E. Functional rafts in cell membranes. Nature 1997; 387(6633):569–72; PMID:9177342; http://dx.doi.org/10.1038/42408
- Huttner WB, Zimmerberg J. Implications of lipid microdomains for membrane curvature, budding and fission. Curr Opin Cell Biol 2001; 13(4):478–84; PMID:11454455; http://dx.doi.org/10.1016/S0955-0674(00)00239-8
- Linden R, Martins VR, Prado MA, Cammarota M, Izquierdo I, Brentani RR. Physiology of the prion protein. Physiol Rev 2008; 88(2):673–728; PMID:18391177; http://dx.doi.org/10.1152/physrev.00007.2007
- Torres M, Castillo K, Armisén R, Stutzin A, Soto C, Hetz C. Prion protein misfolding affects calcium homeostasis and sensitizes cells to endoplasmic reticulum stress. PLoS One 2010; 5(12):e15658; PMID:21209925; http://dx.doi.org/10.1371/journal.pone.0015658
- Moreno JA, Halliday M, Molloy C, Radford H, Verity N, Axten JM, Ortori CA, Willis AE, Fischer PM, Barrett DA, et al. Oral treatment targeting the unfolded protein response prevents neurodegeneration and clinical disease in prion-infected mice. Sci Transl Med 2013; 5(206):206ra138; PMID:24107777; http://dx.doi.org/10.1126/scitranslmed.3006767
- Hetz C. The unfolded protein response: controlling cell fate decisions under ER stress and beyond. Nat Rev Mol Cell Biol 2012; 13(2):89–102; PMID:22251901; http://dx.doi.org/10.1038/nrm3270
- Browman D, Zurzolo C. Not on the menu: autophagy-independent clearance of prions. Prion 2013; 7(4):286–90; PMID:23907058; http://dx.doi.org/10.4161/pri.25809
- Gyllberg H, Löfgren K, Lindegren H, Bedecs K. Increased Src kinase level results in increased protein tyrosine phosphorylation in scrapie-infected neuronal cell lines. FEBS Lett 2006; 580(11):2603–8; PMID:16647068; http://dx.doi.org/10.1016/j.febslet.2006.03.092
- de Chaumont F, Dallongeville S, Chenouard N, Hervé N, Pop S, Provoost T, Meas-Yedid V, Pankajakshan P, Lecomte T, Le Montagner Y, et al. Icy: an open bioimage informatics platform for extended reproducible research. Nat Methods 2012 Jun 28; 9(7):690–6; PMID:22743774; http://dx.doi.org/10.1038/nmeth.2075