ABSTRACT
The sequence of the prion protein gene (PRNP) affects susceptibility to spongiform encephalopathies, or prion diseases in many species. In white-tailed deer, both coding and non-coding single nucleotide polymorphisms have been identified in this gene that correlate to chronic wasting disease (CWD) susceptibility. Previous studies examined individual nucleotide or amino acid mutations; here we examine all nucleotide polymorphisms and their combined effects on CWD. A 626 bp region of PRNP was examined from 703 free-ranging white-tailed deer. Deer were sampled between 2002 and 2010 by hunter harvest or government culling in Illinois and Wisconsin. Fourteen variable nucleotide positions were identified (4 new and 10 previously reported). We identified 68 diplotypes comprised of 24 predicted haplotypes, with the most common diplotype occurring in 123 individuals. Diplotypes that were found exclusively among positive or negative animals were rare, each occurring in less than 1% of the deer studied. Only one haplotype (C, odds ratio 0.240) and 2 diplotypes (AC and BC, odds ratios of 0.161 and 0.108 respectively) has significant associations with CWD resistance. Each contains mutations (one synonymous nucleotide 555C/T and one nonsynonymous nucleotide 286G/A) at positions reported to be significantly associated with reduced CWD susceptibility. Results suggest that deer populations with higher frequencies of haplotype C or diplotypes AC and BC might have a reduced risk for CWD infection – while populations with lower frequencies may have higher risk for infection. Understanding the genetic basis of CWD has improved our ability to assess herd susceptibility and direct management efforts within CWD infected areas.
Abbreviations
AA | = | amino acid |
CWD | = | chronic wasting disease |
IDNR | = | Illinois Department of Natural Resources |
MCMC | = | Markov Chain Monte Carlo |
PRNP | = | prion protein gene |
PrP | = | Prion protein |
TSE | = | transmissible spongiform encephalopathy |
INTRODUCTION
Transmissible spongiform encephalopathies (TSEs) or prion diseases are fatal neurological disorders caused by the misfolding of a common protein (PrPC) into an infectious conformation (PrPSC).Citation1 Chronic wasting disease (CWD) is a TSE that occurs in free ranging cervids, including white-tailed deer, mule deer, elk, and moose.Citation2,3 Originally CWD was described in the 1970s in northern Colorado and southern WyomingCitation4,5 and has since spread to a number of other US states and Canadian provinces.Citation6,7 The first case of CWD detected east of the Mississippi river was in Wisconsin in 2002Citation8 and then Illinois later that year.Citation9-12
CWD is transmitted horizontally (and possibly verticallyCitation13) within white-tailed deer by pathogenic prions shed from the infected host in blood, saliva, urine and feces.Citation14,15 Furthermore, prions have been shown to persist in the environment potentially remaining infectious and causing CWD infection long after affected deer have dispersed.Citation16-18 Cross-species infection of TSEs are rare but fatal, most notably being variant Creutzfeldt-Jakob disease which is the result of bovine spongiform encephalopathy transmission to humans.Citation19 Chronic wasting disease is not known to affect humans; though, there is no consensus among researchers about the possibility of human infection. Studies using mouseCitation20 and primateCitation21 models suggest a strong barrier to human disease transmission. However, under certain experimental conditions cervid PrPSC is capable of converting human PrPC to produce PrPSC.Citation22,23
The PRNP gene was shown to affect prion disease susceptibility and progression in several species.Citation10,24-29 Because CWD is influenced by the expressed protein,Citation1 many studies have focused on the inferred amino acid sequence of PRNP. In white-tailed deer 2 polymorphisms at amino acid positions (aa) Q95H and G96S have been detected that are associated with reduced disease susceptibility.Citation26,27,Citation30 In a captive deer herd in Nebraska, individuals with at least one copy of serine (S) at aa96 were less likely to test positive for CWD.Citation30 Similarly, free ranging deer in Wisconsin were found to have reduced susceptibility to CWD among individuals with a histidine (H) at aa95 or one copy of aa96S; however, complete genetic resistance was not detected and further analysis linked aa96S to slowed disease progression.Citation26,27 Analyses of the PRNP nucleotide sequences corroborated the significance of aa95 and aa96, but also revealed that synonymous mutations were associated with CWD susceptibility.Citation10,28 Furthermore, the cumulative number of nucleotide deviations (both synonymous and non-synonymous) from the database derived consensus sequence for PRNP was found to have a negative correlation with the probability of CWD infection among white-tailed deer.Citation10
A majority of the studies to this point have focused on single locus polymorphisms and CWD susceptibility, with few considering naturally occurring diplotype sequences. One study examined 4 amino acid genotypes consisting of the Q95H and G96S loci (QQ/GG, QQ/GS, QQ/SS, and QH/GG) among free ranging white-tailed deer in Wisconsin, finding only genotypes with at least one copy of S less likely to be CWD positive.Citation29 It is important to note that only 2 amino acid loci were examined and synonymous changes were not addressed. Here we examine the nucleotide sequence of the PRNP gene comparing all observed combinations of synonymous and non-synonymous mutations in white-tailed deer. In this study we explore the relationship between PRNP diplotypes and CWD disease status with the goal of better understanding disease susceptibility.
RESULTS
PRNP sequences were determined for 703 deer by PCR and Sanger sequencing: 579 tested for CWD (105 testing positive, 474 for which CWD was not detected) and 124 that were not tested. Analyses of disease risk were performed using a reduced dataset (N = 240) consisting of deer originating in counties with more than 5 cases of CWD confirmed by government monitoring between 2002–2010 (henceforth referred to as the “CWD infection area;” ). Additional tested and untested samples were obtained from areas with low risk of CWD (counties with fewer than 5 or no confirmed cases at the time of sampling) to better detect sequence variations, haplotypes, and diplotypes. Statistical significance was determined by logistic regression using the most frequent haplotype, diplotype, genotype, or nucleotide as the reference level. Within the analyzed 626 bp region of the PRNP gene 14 variable positions were identified, 10 previously reportedCitation10,28 and 4 novel sites (299G/A, 308A/T, 367G/A, and 372G/A). Of the 14 variable sites 6 are non-synonymous (3 novel and 3 previously reported) and result in a change to the amino acid sequence (). It is important to note that mutations at nt299, nt308, and nt367 (aa100S/N, aa103N/I and aa123A/T respectively) are of interest as the human equivalents (aa97, aa100, and aa120 respectively) are in close proximity to polymorphisms associated with prion disease susceptibility in humans.Citation31-33
FIGURE 1. Map of Illinois (orange) and Wisconsin (pink), showing the study area for samples collected between 2002 and 2010. Samples were collected from all counties in gray by hunter harvest or government culling. Counties within the CWD infection area (at least 5 confirmed cases of CWD during the sample period) are darkly shaded; statistical analyses of CWD susceptibility were restricted to individuals originating from these locations thus increasing the probability of disease exposure. Number of samples from each county is indicated below the county name.
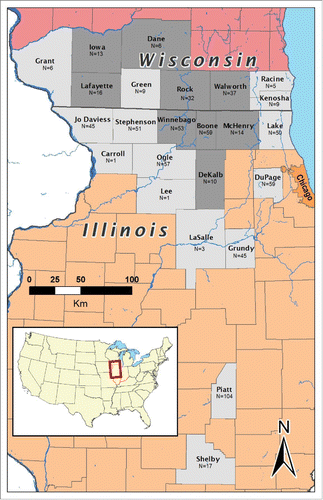
TABLE 1. Variable nucleotide positions for reconstructed haplotypes within the 629bp region of the PRNP gene
Haplotypes were generated from unphased sequences using PHASE v2.1.Citation34,35 Twenty-four haplotypes were predicted from 703 deer (N=1406 possible haplotype copies), with haplotype A occurring most frequently (). Nine haplotypes are found exclusively among negative (haplotypes L, Q, S, T, and W), positive (haplotype R) or untested deer (haplotypes U, V, and X); however, each of these haplotypes is rare with a frequency of occurrence less than 1% (). Seventeen haplotypes occurred within the CWD infection area and only haplotype C is significantly less likely to be found among deer infected with CWD (P < 0.001, OR = 0.240 and 95% CI = 0.104–0.503) ().
TABLE 2. Disease association for unique PRNP haplotypes among deer within the CWD infection area
A total of 68 unique diplotypes were identified among all sampled deer (including both tested and untested individuals from all sampled areas, N = 703). Diplotype AB is the most frequently detected, occurring in 123 deer (positive, negative, and untested deer; ). One diplotype (BF) is found exclusively among negative deer; 9 individuals (1%) carried this diplotype, of which 2 are found within the CWD infection area (). Fifty diplotypes are considered rare having a frequency of occurrence less than 1% and 38 of these are found exclusively among positive, negative or untested deer (3, 26, and 9 diplotypes respectively). Diplotypes AC and BC are significantly less likely to be found among deer infected with CWD (P < 0.05). Odd ratios for these diplotypes are 0.161 and 0.108 (95% CI = 0.024–0.654 and 0.006–0.623) respectively ().
TABLE 3. Frequency of PRNP diplotypes among all sampled white-tailed deer
TABLE 4. Disease association with PRNP diplotypes among white-tailed deer within the CWD infection area
Previous studies determined that polymorphisms aa95H and aa96S (nt285C and nt286A respectively) were significantly associated with reduced CWD susceptibility.Citation10,26-29 We reexamined these positions within our data (including only deer from the CWD infection area, N = 240), confirming aa96S as having a significant effect in reducing infection. We observed no difference in infection between deer with aa95H or aa95Q (nt285A or nt258C; N = 12 and 468 respectively; P = 0.076). However, CWD is less common among aa96S deer than aa96G deer (nt286A and nt286G respectively; N = 58 and 422 respectively; P < 0.001, OR = 0.295, 95% CI = 0.146–0.556) (). Furthermore, deer aa96 heterozygous are significantly less likely to be CWD positive compared to deer aa96G homozygous (P = 0.001, OR = 0.247, 95% CI = 0.104–0.552) (). Among the observed 2 locus genotypes (aa 95/96) only QQ/GS is significant (P < 0.001), having a reduced odds ratio (OR = 0.247, 95% CI = 0.101–0.542; ). It is important to note that while we did not observe a significant difference in infection among deer with the aa95H allele or aa96SS genotype, susceptibility might only be evident with a larger sampling providing greater statistical power.
TABLE 5. Confirmation of PRNP nucleotide positions 285 and 286 (amino acid positions 95 and 96) previously reported as significant for reduced CWD susceptibility
DISCUSSION
In this study, we find reduced susceptibility to CWD infection among white-tailed deer with haplotype C (). We still observed individual deer positive for CWD with this haplotype, demonstrating a reduced susceptibility rather than a complete genetic resistance as is seen with other TSEs (e.g., scrapieCitation36,37). This haplotype had 2 different polymorphisms, 1 synonymous and 1 non-synonymous, both reported to be associated with decreased infection; nt286A (aa96S) and nt555T.Citation10,26,Citation29,30 Other haplotypes have similar mutations at nt286 and nt555 (e.g., haplotypes I, Q, and S); though, within the CWD infection area these haplotypes are not found at all (haplotype Q), occur infrequently (f < 0.01, haplotypes I and S), or are found exclusively among positive deer (haplotype I). A number of other haplotypes have the same mutations at either nt286 or nt555; again most are absent (haplotypes H, V, W and X), infrequent (f < 0.01, haplotypes N and P), or are found abundantly among positive deer (haplotype B) in the CWD infection area (). Rarity of these haplotypes prevents any meaningful association with changes in susceptibility (). The effects of mutations at nt286 and nt555 alone or in concert are unclear as other haplotypes with these polymorphisms occur infrequently and with varied susceptibility. An even larger sampling may be necessary to resolve this interaction.
Neither haplotypes with aa95H (nt285C) had a significantly reduced susceptibility to CWD (). Some previous studies reported the occurrence of this mutation among CWD negative deer only, which was interpreted as CWD resistance.Citation26,29 In this study and in the study by Kelly et al.Citation10 the aa95H mutation was found among deer positive for CWD; however, we find in a larger sampling (N=240) the frequency of aa95H to be lower than that found by Kelly et al.Citation10 and not significantly associated with resistance. We cannot preclude the importance of this mutation given that a significant difference in disease susceptibility may be possible with an even larger sample size providing greater statistical power (data not shown).
The presence of aa96S has been associated with slowed disease progression, longer life span among captive deer,Citation26,27 and does not appear to affect the rate at which prions are shed from infected individuals.Citation38 Additionally, CWD infected mule deer have been found to excrete pathogenic prions while asymptomatic.Citation39 This contributes to concerns that wild deer with aa96S may be shedding infectious prions into the environment for longer periods of time than deer lacking the mutation, but are not symptomatic or detectable by immunohistochemical procedures. On the other hand, studies using epidemiological modeling suggest that deer with aa96S under certain conditions may have a selective advantage for CWD resistance over those without.Citation40 With our data, we are unable to make accurate conclusions about detection, longevity, or increased risks of exposure to infectious prions. Nonetheless, our results do corroborate the importance of the polymorphism at G96S in reduced CWD susceptibility ().Citation26,30
Kelly et al.Citation10 found a negative correlation between the number of nucleotide deviations from the PRNP consensus sequence and CWD infection. The database derived consensus sequence reported is the same as the most common haplotype (haplotype A) in this study (). Haplotype C has 2 deviations from haplotype A; other haplotypes were found containing more deviations but were exceedingly rare (). These haplotypes (namely haplotypes I, N, Q, S, and X) were largely absent among CWD positive deer (only 2 positive deer were found each with a single copy of haplotype I) and their combined frequency was less than 1%. An increased number of polymorphisms may improve resistance to CWD, but the large sample size of this study (N=703) suggests that haplotypes with more than 2 nucleotide deviations are rare and would not be likely to have an appreciable effect on resistance or susceptibility within the population.
Examination of PRNP diplotypes revealed that individuals with at least one copy of haplotype C (specifically AC and BC) were less likely to test positive for CWD (). Other diplotypes containing at least one copy of haplotype C (mutations at aa96S and nt555T) had a low frequency of occurrence (<1%); therefore, individually these less frequent diplotypes may not be significant for CWD resistance but they could play a vital role in decreasing population-level susceptibility by increasing the frequency of the C haplotype over time through inheritance (i.e. herd immunity). Under ideal circumstances, determining genetic association with disease status is examined under controlled experimental conditions to account for all confounding factors;Citation41,42 though, this is not always possible when studying free ranging animals. To address this, other studies have attempted to use matched-case or paired-case control design to increase the likelihood that samples have a similar genetic background.Citation29 For this study, perfectly paired samples were not obtainable due to the nature of sampling through management and hunter harvest. Nonetheless, negative deer were selected from available samples to match with positive deer on the basis of age, sex, and geographic origin to minimize any potential bias. Additional samples were randomly selected outside of the CWD infection area. To avoid spurious results, statistical analyses were restricted to deer originating in the infected area as these animals are more likely to have been exposed to the disease than deer from counties without identified cases of CWD. The relationship between PRNP sequence and CWD status was found in multiple geographic locations at distances greater than the average home range of Illinois white-tailed deerCitation43-45 (i.e., deer with haplotype C were not restricted to one county and were found throughout the study area), suggesting that relatedness and family groups were not a confounding factor and that these results are a strong indication of low genetic susceptibility.
The PRNP gene is variable within all species with some mutations affecting susceptibility to TSEs.Citation46-48 Scrapie infection in sheep is the classic example of genetic resistance to a prion disease, where individuals with 2 copies of amino acid sequence V136, R154, Q171 are susceptible to scrapie, and those with 2 copies of the sequence A136, R154, R171 are resistant.Citation36,37 Changes in the protein coding sequence have been shown to affect the ability of pathogenic prions to convert normal prion proteinsCitation31; accordingly, many studies have heavily examined the amino acid variations associated with CWD. Synonymous or silent mutations are often overlooked, but may have a greater effect on protein expression and conformation than expected.Citation49-53Other studies have found significant associations between individual synonymous mutations and CWD susceptibility.Citation10,28 The specific mechanisms involved between nucleotide variation (specifically synonymous mutations) and CWD are not known, but the rate at which PrPC conformations that are more favorable to PrPSC conversion are produced may be slowed by the presence of certain synonymous mutations.Citation51 Due to the low frequency of haplotypes with similar mutations as haplotype C, we cannot accurately conclude whether or not the specific combination of mutations or any one mutation alone is responsible for reduced CWD susceptibility. Nevertheless, haplotype and diplotype analyses provide more insight in gene-disease association than those restricted to alleles and genotypesCitation54 which are unable to detect additive effects.
A solid understanding of the genetics of CWD in white-tailed deer is vital to improve management of CWD on the landscape. Most TSEs are found in domestic or captive animals where management of infected individuals is feasible. For example, scrapie infected flocks can be handled through a process generally involving genetic testing, removal and destruction of infected or suspect animals, followed by decontamination of facilities and equipment.Citation55 Containment of free ranging deer in wild populations potentially infected with CWD and decontamination of the environment is not reasonably possible. The long term effects of CWD are not yet known but it is conceivable that an unmanaged infected population would be gradually extirpated as the disease progressesCitation56,57 or at least reduced to low densities with high disease prevalence.Citation58,59 Either outcome would have severe ecological effects (e.g., deer play a major role in affecting plant communitiesCitation60 and as a prey sourceCitation61,62) as well as negative economic impacts to hunting. Overall disease prevalence has remained at relatively low levels in Illinois compared to Wisconsin.Citation11 It is important to note that at the time of sampling, CWD had been found in 6 Illinois counties and has since been detected in 14.Citation9 Complete eradication of CWD among free ranging white-tailed deer may not be possible; however, an active containment effort in Illinois appears to have prevented significant increases in prevalence.Citation9,11,Citation12 Further examination of PRNP haplotype and diplotype frequencies across northern Illinois and southern Wisconsin in conjunction with population structure and movementCitation45,63,Citation64 will be useful in identifying localities with greater or reduced susceptibility risk. Effectiveness of CWD containment efforts can be aided through genetic testing and redirecting management resources.
MATERIALS AND METHODS
Deer Sampling and CWD Testing
Seven hundred three samples were collected between 2002 and 2010 from wild free-ranging white-tailed deer in Illinois and southern Wisconsin from both public hunting and government culling. For Illinois samples, obex and retropharyngeal lymph nodes were tested using USDA approved immunohistochemical (IHC) procedures to detect protease-resistant prion protein (PrPSC) at the Illinois Department of Agriculture Diagnostic Laboratories in Galesburg or Centralia and most positives were confirmed at the National Veterinary Services Laboratory. Untested samples originated from areas where CWD had not been detected or where there was a low risk at the time of sampling; these were included to determine the extent of PRNP variability. Tissues samples (skeletal muscle, mainly tongue) were archived for both CWD positive and negative deer. Wisconsin samples were tested for CWD by the Wisconsin Veterinary Diagnostic Laboratory by IHC or ELISA based procedures with all positives confirmed by IHC. At the time of sampling, detailed information including location (1.6 × 1.6 km area), sex, and age was recorded. Deer for this study were selected from a larger sampling; those originating outside of the CWD infected area were chosen randomly. Within the infected area to minimize bias, CWD negative deer were selected to match with positive deer on the basis of age, sex, and geographic origin.
PRNP Amplification and Sequencing
Genomic DNA was isolated from skeletal muscle using the Wizard Genomic DNA purification kit (Promega, Madison, WI) following the manufacturer's recommended protocol. A 626 bp region of the PRNP gene was amplified by polymerase chain reaction using previously published primers CWD-13 and CWD-LACitation65 or primers 223 and 224.Citation30 Amplification was performed in 40 ul reaction volumes following previously published protocols.Citation10,65
PCR amplicons were purified using the Wizard SV Gel and PCR Clean-Up System (Promega, Madison, WI). Products were then sequenced using the BigDye Terminator system (ABI), purified, and resolved on an ABI 3730XL DNA Sequencer at the University of Illinois Keck Center for Functional and Comparative Genomics. The software Sequencher (Gene Codes Corporation, Ann Arbor, MI) was used to edit and concatenate sequences. The identities of DNA sequences were confirmed using NCBI BLAST (http://www.ncbi.nlm.nih.gov/blast/Blast.cgi) and variable positions were identified by comparison to published DNA sequence. Open reading frames were confirmed and sequences translated in MEGA v6.0.Citation66 Sequences were checked for the absence of the aa138 mutation to ensure that all sequences were PRNP and not the processed pseudogeneCitation26; asparagine (N) at aa138 (nt413A) would indicate amplification of the pseudogene. If aa138N was detected with primers CWD-13 and CWD-LA, then the sequence was verified with primers 223 and 224 which were specifically designed to only amplify the functional gene.Citation30 Though it is possible that this mutation could also occur in the functional gene, we did not observe aa138N in any deer when both primer sets were used.
Analysis
Haplotypes were generated from unphased sequences using PHASE v2.1.Citation34,35 Markov chain Monte Carlo (MCMC) samples were taken from a minimum of 100,000 steps, with a discarded burn-in of 10,000; samples were drawn every 100 MCMC steps. Five repetitions were performed and haplotype frequencies compared to verify consistent assignment. Logistic regression was calculated for haplotype, diplotype, genotype, or nucleotide, with each variable treated as categorical data and the most frequent for each as the reference level. Disease status was binary, with infected deer as one and uninfected deer as zero. Odds ratios were calculated for significant variables (alpha 0.05); ratios less than one were considered to have reduced CWD susceptibility. All calculations were performed in R version 3.0.0 Citation67 with R Studio v0.98.1083.Citation68
DISCLOSURE OF POTENTIAL CONFLICTS OF INTEREST
No potential conflicts of interest were disclosed.
ACKNOWLEDGMENTS
We thank the Illinois Department of Natural Resources for their efforts in collecting samples and the hunting community of Illinois and Wisconsin for their willingness to have deer tested for CWD. For technical assistance we thank the students and technicians of the collaborative Novakofski and Mateus-Pinilla laboratories.
FUNDING
This study was supported by the US Fish and Wildlife Service Federal Aid in Wildlife Restoration Project (W-146-R), the Illinois Natural History Survey, and the University of Illinois Office of the Vice Chancellor for Research.
REFERENCES
- Prusiner SB. Prions. Proc Natl Acad Sci U S A 1998; 95:13363-83; PMID:9811807; http://dx.doi.org/10.1073/pnas.95.23.13363
- Belay ED, Maddox RA, Williams ES, Miller MW, Gambetti P, Schonberger LB. Chronic wasting disease and potential transmission to humans. Emerg Infect Dis 2004; 10:977-84; PMID:15207045; http://dx.doi.org/10.3201/eid1006.031082
- Baeten LA, Powers BE, Jewell JE, Spraker TR, Miller MW. A natural case of chronic wasting disease in a free-ranging moose (Alces alces shirasi). J Wildl Dis 2007; 43:309-14; PMID:17495319; http://dx.doi.org/10.7589/0090-3558-43.2.309
- Williams ES, Young S. Chronic wasting disease of captive mule deer - spongiform encephalopathy. J Wildl Dis 1980; 16:89-98; PMID:7373730; http://dx.doi.org/10.7589/0090-3558-16.1.89
- Williams ES, Young S. Spongiform encephalopathy of Rocky-Mountain elk. J Wildl Dis 1982; 18:465-71; PMID:7154220; http://dx.doi.org/10.7589/0090-3558-18.4.465
- Chronic wasting disease: Frequently asked questions [Internet]. Springfield, Illinois: Illinois Department of Natural Resources, 2015. [cited 2015 Aug 6] Avialable from: http://www.dnr.illinois.gov/programs/CWD/Pages/FAQs/aspx.
- Richards BJ, Slota P. Chronic wasting disease (CWD) [Internet]. Madison, Wisconsin: USGS National Wildlife Health Center, 2015. [cited 2015 Apr 29] Available from: http://www.nwhc.usgs.gov/disease_information/chronic_wasting_disease/.
- Joly DO, Ribic CA, Langenberg JA, Beheler K, Batha CA, Dhuey BJ, Rolley RE, Bartelt G, Van Deelen TR, Samuel MD. Chronic wasting disease in free-ranging Wisconsin white-tailed deer. Emerg Infect Dis 2003; 9:599-601; PMID:12737746; http://dx.doi.org/10.3201/eid0905.020721
- Shelton P, McDonald P. Illinois chronic wasting disease: 2013-2014 surveillance and management report [Internet]. 2014:1-15. [Cited 2015 Aug 6] Available from: https://www.dnr.illinois.gov/programs/CWD/Documents/CWDAnnualReport20132014.pdf.
- Kelly AC, Mateus-Pinilla NE, Diffendorfer J, Jewell E, Ruiz MO, Killefer J, Shelton P, Beissel T, Novakofski J. Prion sequence polymorphisms and chronic wasting disease resistance in Illinois white-tailed deer (Odocoileus virginianus). Prion 2008; 2:28-36; PMID:19164895; http://dx.doi.org/10.4161/pri.2.1.6321
- Manjerovic MB, Green ML, Mateus-Pinilla N, Novakofski J. The importance of localized culling in stabilizing chronic wasting disease prevalence in white-tailed deer populations. Prev Vet Med 2014; 113:139-45; PMID:24128754; http://dx.doi.org/10.1016/j.prevetmed.2013.09.011
- Mateus-Pinilla N, Weng H-Y, Ruiz MO, Shelton P, Novakofski J. Evaluation of a wild white-tailed deer population management program for controlling chronic wasting disease in Illinois, 2003-2008. Prev Vet Med 2013; 110:541-8; PMID:23558033; http://dx.doi.org/10.1016/j.prevetmed.2013.03.002
- Nalls AV, McNulty E, Powers J, Seelig DM, Hoover C, Haley NJ, Hayes-Klug J, Anderson K, Stewart P, Goldmann W, et al. Mother to offspring transmission of chronic wasting disease in Reeves' Muntjac deer. Plos One 2013; 8:e71844; http://dx.doi.org/10.1371/journal.pone.0071844
- Gough KC, Maddison BC. Prion transmission prion excretion and occurrence in the environment. Prion 2010; 4:275-82; PMID:20948292; http://dx.doi.org/10.4161/pri.4.4.13678
- Haley NJ, Mathiason CK, Carver S, Zabel M, Telling GC, Hoover EA. Detection of chronic wasting disease prions in salivary, urinary, and intestinal tissues of deer: potential mechanisms of prion shedding and transmission. J Virol 2011; 85:6309-18; PMID:21525361; http://dx.doi.org/10.1128/JVI.00425-11
- Johnson CJ, Phillips KE, Schramm PT, McKenzie D, Aiken JM, Pedersen JA. Prions adhere to soil minerals and remain infectious. PLoS Pathog 2006; 2:e32-e; PMID:16617377; http://dx.doi.org/10.1371/journal.ppat.0020032
- Nichols TA, Pulford B, Wyckoff AC, Meyerett C, Michel B, Gertig K, Hoover EA, Jewell JE, Telling GC, Zabel MD. Detection of protease-resistant cervid prion protein in water from a CWD-endemic area. Prion 2009; 3:171-83; PMID:19823039; http://dx.doi.org/10.4161/pri.3.3.9819
- Kuznetsova A, McKenzie D, Banser P, Siddique T, Aiken JM. Potential role of soil properties in the spread of CWD in western Canada. Prion 2014; 8:92-9; PMID:24618673; http://dx.doi.org/10.4161/pri.28467
- Maheshwari A, Fischer M, Gambetti P, Parker A, Ram A, Soto C, Concha-Marambio L, Cohen Y, Belay ED, Maddox RA, et al. Recent US case of variant Creutzfeldt-Jakob disease - global implications. Emerg Infect Dis J 2015; 21:750; http://dx.doi.org/10.3201/eid2105.142017
- Kurt TD, Telling GC, Zabel MD, Hoover EA. Trans-species amplification of Prp(CWD) and correlation with rigid loop 170N. Virology 2009; 387:235-43; PMID:19269662; http://dx.doi.org/10.1016/j.virol.2009.02.025
- Race B, Meade-White KD, Miller MW, Barbian KD, Rubenstein R, LaFauci G, Cervenakova L, Favara C, Gardner D, Long D, et al. Susceptibilities of nonhuman primates to chronic wasting disease. Emerg Infect Dis 2009; 15:1366-76; PMID:19788803; http://dx.doi.org/10.3201/eid1509.090253
- Barria MA, Telling GC, Gambetti P, Mastrianni JA, Soto C. Generation of a new form of human PrPSc in vitro byinterspecies transmission from cervid prions. J Biol Chem 2011; 286:7490-5; PMID:21209079; http://dx.doi.org/10.1074/jbc.M110.198465
- Davenport KA, Henderson DM, Mathiason CK, Hoover EA. Insights into CWD and BSE species barriers using real-time conversion. J Virol 2015; 89(18):9524-31; PMID:26157118
- Carlson GA, Kingsbury DT, Goodman PA, Coleman S, Marshall ST, Dearmond S, Westaway D, Prusiner SB. Linkage of prion protein and scrapie incubation-time genes. Cell 1986; 46:503-11; PMID:3015416; http://dx.doi.org/10.1016/0092-8674(86)90875-5
- White SN, Spraker TR, Reynolds JO, O'Rourke K. Association analysis of PRNP gene region with chronic wasting disease in Rocky Mountain elk. BMC Res Notes 2010; 3:7pp.-pp; http://dx.doi.org/10.1186/1756-0500-3-314
- Johnson C, Johnson J, Vanderloo JP, Keane D, Aiken JM, McKenzie D. Prion protein polymorphisms in white-tailed deer influence susceptibility to chronic wasting disease. J Gen Virol 2006; 87:2109-14; PMID:16760415; http://dx.doi.org/10.1099/vir.0.81615-0
- Johnson CJ, Herbst A, Duque-Velasquez C, Vanderloo JP, Bochsler P, Chappell R, McKenzie D. Prion protein polymorphisms affect chronic wasting disease progression. Plos One 2011; 6:e17450.
- Wilson GA, Nakada SM, Bollinger TK, Pybus MJ, Merrill EH, Coltman DW. Polymorphisms at the PRNP gene influence susceptibility to chronic wasting disease in two species of deer (Odocoileus Spp.) in western Canada. J Toxicol Environ Health A 2009; 72:1025-9; http://dx.doi.org/10.1080/15287390903084264
- Blanchong JA, Heisey DM, Scribner KT, Libants SV, Johnson C, Aiken JM, Langenberg JA, Samuel MD. Genetic susceptibility to chronic wasting disease in free-ranging white-tailed deer: Complement component C1q and Prnp polymorphisms. Infect Genet Evol 2009; 9:1329-35; http://dx.doi.org/10.1016/j.meegid.2009.08.010
- O'Rourke KI, Spraker TR, Hamburg LK, Besser TE, Brayton KA, Knowles DP. Polymorphisms in the prion precursor functional gene but not the pseudogene are associated with susceptibility to chronic wasting disease in white-tailed deer. J Gen Virol 2004; 85:1339-46; PMID:15105552; http://dx.doi.org/10.1099/vir.0.79785-0
- Asante EA, Smidak M, Grimshaw A, Houghton R, Tomlinson A, Jeelani A, Jakubcova T, Hamdan S, Richard-Londt A, Linehan JM, et al. A naturally occurring variant of the human prion protein completely prevents prion disease. Nature 2015; 522:478-+; PMID:26061765; http://dx.doi.org/10.1038/nature14510
- Collinge J, Palmer MS, Dryden AJ. Genetic predisposition to iatrogenic Creutzfeldt-Jakob Disease. Lancet 1991; 337:1441-2; PMID:1675319; http://dx.doi.org/10.1016/0140-6736(91)93128-V
- Ghetti B, Dlouhy SR, Giaccone G, Bugiani O, Frangione B, Farlow MR, Tagliavini F. Gerstmann-Straussler-Scheinker disease and the Indiana kindred. Brain Pathol 1995; 5:61-75; PMID:7767492; http://dx.doi.org/10.1111/j.1750-3639.1995.tb00578.x
- Stephens M, Scheet P. Accounting for decay of linkage disequilibrium in haplotype inference and missing-data imputation. Am J Hum Genet 2005; 76:449-62; PMID:15700229; http://dx.doi.org/10.1086/428594
- Stephens M, Smith NJ, Donnelly P. A new statistical method for haplotype reconstruction from population data. Am J Hum Genet 2001; 68:978-89; PMID:11254454; http://dx.doi.org/10.1086/319501
- Baylis M, Goldmann W. The genetics of scrapie in sheep and goats. Curr Mol Med 2004; 4:385-96; PMID:15354869; http://dx.doi.org/10.2174/1566524043360672
- Roden JA, Nieuwhof GJ, Bishop SC, Jones DA, Haresign W, Gubbins S. Breeding programmes for TSE resistance in British sheep - I. Assessing the impact on prion protein (PrP) genotype frequencies. Prev Vet Med 2006; 73:1-16; PMID:16169614; http://dx.doi.org/10.1016/j.prevetmed.2005.08.002
- Henderson DM, Denkers ND, Hoover CE, Garbino N, Mathiason CK, Hoover EA. Longitudinal Detection of Prion Shedding in Saliva and Urine by CWD-Infected Deer by RT-QuIC. J Virol 2015:JVI. 01118-15.
- Tamgüeney G, Miller MW, Wolfe LL, Sirochman TM, Glidden DV, Palmer C, Lemus A, DeArmond SJ, Prusiner SB. Asymptomatic deer excrete infectious prions in faeces. Nature 2009; 461:529-U90; PMID:19741608; http://dx.doi.org/10.1038/nature08289
- Robinson SJ, Samuel MD, Johnson CJ, Adams M, McKenzie DI. Emerging prion disease drives host selection in a wildlife population. Ecol Appl 2012; 22:1050-9; PMID:22645831; http://dx.doi.org/10.1890/11-0907.1
- Jewell JE, Conner MM, Wolfe LL, Miller MW, Williams ES. Low frequency of PrP genotype 225SF among free-ranging mule deer (Odocoileus hemionus) with chronic wasting disease. J Gen Virol 2005; 86:2127-34; PMID:16033959; http://dx.doi.org/10.1099/vir.0.81077-0
- Race B, Meade-White K, Miller MW, Fox KA, Chesebro B. In vivo comparison of chronic wasting disease infectivity from deer with variation at prion protein residue 96. J Virol 2011; 85:9235-8; PMID:21697479; http://dx.doi.org/10.1128/JVI.00790-11
- Brinkman TJ, Deperno CS, Jenks JA, Haroldson BS, Osborn RG. Movement of female white-tailed deer: Effects of climate and intensive row-crop agriculture. J Wildl Management 2005; 69:1099-111; http://dx.doi.org/10.2193/0022-541X(2005)069%5b1099:MOFWDE%5d2.0.CO;2
- Nixon CM, Mankin PC, Etter DR, Hansen LP, Brewer PA, Chelsvig JE, Esker TL, Sullivan JB. White-tailed deer dispersal behavior in an agricultural environment. American Midland Naturalist 2007; 157:212-20; http://dx.doi.org/10.1674/0003-0031(2007)157%5b212:WDDBIA%5d2.0.CO;2
- Green ML, Manjerovic MB, Mateus-Pinilla N, Novakofski J. Genetic assignment tests reveal dispersal of white-tailed deer: implications for chronic wasting disease. J Mammal 2014; 95:646-54; http://dx.doi.org/10.1644/13-MAMM-A-167
- Novakofski J, Brewer MS, Mateus-Pinilla N, Killefer J, McCusker RH. Prion biology relevant to bovine spongiform encephalopathy. J Anim Sci 2005; 83:1455-76; PMID:15890824
- Heaton MP, Leymaster KA, Freking BA, Hawk DA, Smith TPL, Keele JW, Snelling WM, Fox JM, Chitko-McKown CG, Laegreid WW. Prion gene sequence variation within diverse groups of US sheep, beef cattle, and deer. Mamm Genome 2003; 14:765-77; PMID:14722726; http://dx.doi.org/10.1007/s00335-003-2283-y
- Robinson SJ, Samuel MD, O'Rourke KI, Johnson CJ. The role of genetics in chronic wasting disease of North American cervids. Prion 2012; 6:153-62; PMID:22460693; http://dx.doi.org/10.4161/pri.19640
- Duan JB, Wainwright MS, Comeron JM, Saitou N, Sanders AR, Gelernter J, Gejman PV. Synonymous mutations in the human dopamine receptor D2 (DRD2) affect mRNA stability and synthesis of the receptor. Hum Mol Genet 2003; 12:205-16; PMID:12554675; http://dx.doi.org/10.1093/hmg/ddg055
- Kudla G, Murray AW, Tollervey D, Plotkin JB. Coding-sequence determinants of gene expression in Escherichia coli. Science 2009; 324:255-8; PMID:19359587; http://dx.doi.org/10.1126/science.1170160
- Sauna ZE, Kimchi-Sarfaty C. Understanding the contribution of synonymous mutations to human disease. Nat Rev Genet 2011; 12:683-91; PMID:21878961; http://dx.doi.org/10.1038/nrg3051
- Biro JC. Coding nucleic acids are chaperons for protein folding: A novel theory of protein folding. Gene 2013; 515:249-57; PMID:23266645; http://dx.doi.org/10.1016/j.gene.2012.12.048
- Pereira RM, Mesquita P, Batista M, Baptista MC, Barbas JP, Pimenta J, Santos IC, Marques MR, Vasques MI, Pereira MS, et al. Doppel gene polymorphisms in Portuguese sheep breeds: Insights on ram fertility. Anim Reprod Sci 2009; 114:157-66; PMID:19028030; http://dx.doi.org/10.1016/j.anireprosci.2008.10.003
- Zuo L, Wang K, Luo X. Use of diplotypes - matched haplotype pairs from homologous chromosomes - in gene-disease association studies. Shanghai Arch Psychiatry 2014; 26:165-70; PMID:25114493
- US. Department of Agriculture - Animal and Plant Health Inspection Service. Scrapie eradication uniform methods and rules. 2005.
- Gross JE, Miller MW. Chronic wasting disease in mule deer: disease dynamics and control. J Wildl Management 2001; 65:205-15; http://dx.doi.org/10.2307/3802899
- Almberg ES, Cross PC, Johnson CJ, Heisey DM, Richards BJ. Modeling routes of chronic wasting disease transmission: environmental prion persistence promotes deer population decline and extinction. Plos One 2011; 6:e19896; PMID:21603638; http://dx.doi.org/10.1371/journal.pone.0019896
- Wasserberg G, Osnas EE, Rolley RE, Samuel MD. Host culling as an adaptive management tool for chronic wasting disease in white-tailed deer: a modelling study. J Appl Ecol 2009; 46:457-66; PMID:19536340; http://dx.doi.org/10.1111/j.1365-2664.2008.01576.x
- Miller MW, Swanson HM, Wolfe LL, Quartarone FG, Huwer SL, Southwick CH, Lukacs PM. Lions and prions and deer demise. Plos One 2008; 3:e4019.
- Rooney TP, Waller DM. Direct and indirect effects of white-tailed deer in forest ecosystems. Forest Ecol Management 2003; 181:165-76; http://dx.doi.org/10.1016/S0378-1127(03)00130-0
- Kilgo JC, Ray HS, Vukovich M, Goode MJ, Ruth C. Predation by coyotes on white-tailed deer neonates in South Carolina. J Wildl Management 2012; 76:1420-30; http://dx.doi.org/10.1002/jwmg.393
- Kunkel KE, Mech LD. Wolf and bear predation on white-tailed deer fawns in northeastern Minnesota. Canadian J Zool-Revue Canadienne De Zoologie 1994; 72:1557-65; http://dx.doi.org/10.1139/z94-207
- Kelly AC, Mateus-Pinilla NE, Brown W, Ruiz MO, Douglas MR, Douglas ME, Shelton P, Beissel T, Novakofski J. Genetic assessment of environmental features that influence deer dispersal: implications for prion-infected populations. Population Ecology 2014; 56:327-40; http://dx.doi.org/10.1007/s10144-013-0427-9
- Kelly AC, Mateus-Pinilla NE, Douglas M, Douglas M, Brown W, Ruiz MO, Killefer J, Shelton P, Beissel T, Novakofski J. Utilizing disease surveillance to examine gene flow and dispersal in white-tailed deer. J Appl Ecol 2010; 47:1189-98; http://dx.doi.org/10.1111/j.1365-2664.2010.01868.x
- Johnson C, Johnson J, Clayton M, McKenzie D, Aiken J. Prion protein gene heterogeneity in free-ranging white-tailed deer within the chronic wasting disease affected region of Wisconsin. J Wildl Dis 2003; 39:576-81; PMID:14567218; http://dx.doi.org/10.7589/0090-3558-39.3.576
- Tamura K, Stecher G, Peterson D, Filipski A, Kumar S. MEGA6: Molecular Evolutionary Genetics Analysis Version 6.0. Mol Biol Evol 2013; 30:2725-9; PMID:24132122; http://dx.doi.org/10.1093/molbev/mst197
- R Core Team. R: A language and environment for statistical computing (version 3.0.0). 2013. Vienna, Austria: R Foundation for Statistical Computing. Available at http://www.R-project.org/
- RStudio. RStudio: Integrated development environment for R 2012.