ABSTRACT
Amyloidogenic proteins have an increased propensity to reorganize into the highly structured, β sheet rich structures that characterize amyloid. The probability of attaining these highly structured assemblies is influenced by multiple factors, including amino acid composition and environmental conditions. Evolutionary selection for amino acid sequences that prevent amyloid formation could further modulate amyloid-forming propensity. Indeed, we have recently identified specific proline and lysine residues, contained within a highly conserved central region of prion protein (PrP), that impede PrP amyloid formation in vitro. These prolines are mutated in certain forms of the human familial genetic disease, Gerstmann-Straüssler-Schneiker (GSS) syndrome. Here, I discuss the influence of these proline and lysine residues on PrP amyloid formation and how such anti-amyloidogenic primary amino acid sequences might be modulated to influence protein amyloidogenicity.
KEYWORDS:
Amyloid fibrils can be characterized by their highly ordered, cross β structure.Citation1 Misfolding of proteins into amyloid has been implicated in a number of diseases including Alzheimer's, type II diabetes and prion disease. The propensity for amyloid formation is multifactorial and is influenced by amino acid sequence, protein concentration, post-translational modifications and environmental conditions. Protein expression levels are inversely correlated with their aggregation propensity, leading to the hypothesis that protein expression levels are evolutionarily maintained on the edge of solubility to optimize performance.Citation2 Maintaining protein expression at a solubility threshold means that even a single genetic mutation in a protein can result in increased aggregation and amyloid formation. Under certain experimental conditions, even highly soluble proteins can form amyloids, indicating that the ability to form amyloid is a generic characteristic shared among most proteins.Citation3,4 The possibility has been raised that aggregation avoidance is an important driving force in natural protein structure evolution.Citation4 Presumably, this evolutionary pressure to prevent amyloid formation would influence the primary amino acid content of a protein.
Evidence indicates that primary amino acid composition and sequence can indeed modulate amyloid formation.Citation5 Hydrophobic and aromatic amino acids are thought to promote prion amyloid formation whereas proline and charged residues strongly inhibit prion formation.Citation6 Inhibitory residues presumably impede amyloid formation due to the structural constraints and electrostatic repulsion of the proline and charged amino acid sidechains, respectively. Proline residues are often excluded from β-sheet secondary structure, likely due to the torsional conformational constraints the cyclic nature of proline imposes on the peptide backbone. Incorporation of charged amino acids into amyloid structure might introduce electrostatic repulsion, particularly in amyloid organized in a parallel in-register β-sheet architecture with identical amino acid sidechains stacked in a fibril.
Changes in the amino acid composition can markedly influence the propensity for prion amyloid formation. In yeast prions, removal of inhibitory amino acids (P,K,R,D,E) promotes prion amyloid formation of non-prion forming domains.Citation7,8 with amyloid-inhibitory amino acids being underrepresented in prion-like domains.Citation7 In filamentous fungus, the HET-s amyloid motif drives HET-s prion propagation to modulate a cell-death cascade as part of a non-self recognition mechanism.Citation9 The functional HET-s amyloid motif contains a decreased number of E, D and K and increased number of S and H residues.Citation10 Analysis of the evolutionary diversification of the HET-s amyloid motif shows distinct groupings into subfamilies and not a continuum of species, indicating the amyloid motif did not arise as a function of evolutionary drift.Citation10 Instead, such discrete family grouping suggests specific evolutionary selection for the amino acid composition of the amyloid motif. The influence of amino acid composition on amyloid formation is also seen with mammalian prion protein (PrP). Replacement of specific proline and lysine residues within a highly conserved central region of mammalian PrP with aliphatic or uncharged polar residues can promote amyloid formation.Citation11,12 Given that a single amino acid can prevent or promote amyloid formation suggests that there is specific evolutionary selection of primary amino acid sequences to prevent pathological aggregation or, in the case of some yeast and fungal prions, promote prion formation.
The highly conserved central region, or central lysine cluster (CLC), of mammalian PrP contains four lysine and two proline residues within a short amino acid span encompassing residues 101-110. Based on primary amino acid sequence alone, this region would be predicted to be highly anti-amyloidogenic. We considered the possibility that there is evolutionary pressure to select for the CLC lysine and proline residues to prevent PrP amyloid formation, particularly in long-lived mammals. To examine this, we looked at the correlation between the amino acid sequence of the central lysine cluster (CLC) and mammalian longevity or susceptibility to prion disease.Citation11 While we did not observe an obvious relationship between the sequence of the central lysine cluster (CLC) and longevity or prion disease susceptibility, we did observe that substitution of the K or P residues within the CLC region accelerated PrP amyloid formation in vitro.Citation11 Substitution of P102 to leucine alone was sufficient to accelerate spontaneous PrP amyloid formation.Citation11 Collective substitution of all 4 lysine and 2 proline residues contained within the CLC with asparagine and alanine residues respectively accelerated PrP amyloid formation to a degree that it was no longer influenced by the addition of a prion seed.Citation11 Thus, the proline and lysine composition of the relatively short CLC can strongly impede amyloid formation of full-length PrP.Citation11,12 Mutation of the CLC prolines, P102 or P105, is associated with the most common form of genetic prion disease, Gerstmann-Straüssler-Scheinker syndrome. Collectively, this suggests that the P and K residues play a role in impeding amyloid formation by PrP and highlight the CLC as a critical region in mediating PrP amyloidogenicity. Alternatively, substitution of the CLC proline and lysine residues could destabilize the native state of PrP and consequently increase PrP amyloidogenicity. While there is no apparent relationship between the CLC sequence and mammalian longevity, the high degree of conservation among diverse mammalian species is consistent with there being evolutionary pressure to maintain the lysine and proline residues contained within the CLC to prevent PrP amyloid formation. Additionally, or alternatively, the precise sequence of the CLC region may be critical for the normal physiological function of native prion protein, PrPC.
Despite their anti-amyloidogenic properties, substitution of the CLC proline and lysine residues is not a requirement for refolding of PrPC into infectious prions (PrPSc). So, in the absence of genetic substitution of the amyloid-prohibitive residues contained, how are the anti-amyloid effects of the CLC overcome to allow PrPSc amyloid formation? Possibilities include secondary modification of the CLC conformation or side-chain structure and/or coordination of cofactors that could effectively replicate the impacts of proline or lysine substitution within the CLC.
The replacement of a single amino acid, such as proline, can promote amyloid formation in PrP and other amyloidogenic proteins, such as the P301S tau mutant.Citation13,14 However, amyloid formation can also be modulated through post-translational modifications, including proline isomerization. Amyloid formation of β2-microglobulin (β2M) is triggered by a cis-trans peptidyl-prolyl isomerization event at Pro32.Citation15 An increase in the population of trans Pro32 isomers allows amyloid assembly of β2M at neutral pH in the absence of denaturants, glycosaminoglycans, Cu2+, or the increased salt concentrations that are typically used experimentally to promote β2M amyloid formation.Citation15 NMR studies indicate that an N-terminally truncated β2M with increased amyloid potential because of a favored trans Pro32 isomer is able to catalyze the amyloid formation of nonamyloidogenic β2M.Citation16 This is attributed to a direct interaction of the two molecules and an increase in the trans-isomer of the X-Pro32 bond to promote amyloid assembly.Citation16 Regulation of proline residues has also been implicated in amyloid formation by other proteins including Aβ and tau. A peptidyl-prolyl isomerase (PPIase), Pin1, regulates amyloid precursor protein (APP) processing and the phosphorylation and subsequent aggregation of tau. Pin1 is a phosphorylation-specific PPIase, recognizing Pro-directed phosphorylated Ser/Thr peptide sequences. Proline-directed kinases and phosphatases are conformation specific and bind only in the presence a specific proline isomer.Citation17-19 This conformer specificity allows the proline isomerization state to influence amyloid formation on multiple levels. First, a specific proline isomer itself could prevent amyloid formation. Second, proline isomerization could alter the binding of a phosphatase or kinase and thereby alter post-translational modifications that could contribute to the propensity for amyloid formation, such as tau hyperphosphorylation. Thus, proline isomerization could effectively act as a surrogate for genetic substitution in modulating amyloid-forming propensity.
Infectious mammalian transmissible spongiform encephalopathy (TSE) prions can be generated de novo with the addition of cofactors including phospholipids and RNA.Citation20-23 Cofactors, particularly polyanionic cofactors shown to support prion formation, are likely coordinated at polybasic regions such as the central lysine cluster. These cofactors would presumably neutralize the charges of the four highly conserved lysine residues adjacent to the disease-linked prolines 102 and 105. The anionic cofactor POPG has been shown to bind at the CLC and adjacent hydrophobic region to induce refolding of PrP into a protease-resistant form.Citation24 POPG binding and PrP refolding is decreased with substitution of P102 or P105, but not the CLC lysine residues, indicating a role of the CLC proline residues in coordinating cofactor binding. Cofactor binding itself could influence the isomer equilibrium of P102 and P105. The cis-trans proline isomer equilibrium undergoes slow exchange in the absence of PPIases. It has been suggested, at least for β2M, that the proline isomer equilibrium is likely also influenced by other cofactors including GAGS, lipids, and Cu2+ Citation15,25 to induce amyloid refolding. Multiple studies have indicated that copper can influence proline isomerization states.Citation26,27 Regardless of whether cofactors influence proline isomerization within the CLC, the charge neutralizing effects of a polyanionic binding partner would help overcome the inhibitory effects of the CLC lysines to promote amyloid formation.
In bona fide infectious prions, residues inclusive of ~90-231 are tightly packed into a protease-resistant, solvent exclusive amyloid core. Thus, refolding of PrPC into PrPSc requires the incorporation of the highly charged CLC polybasic region with its two proline residues. The lack of a high-resolution structure of PrPSc currently precludes a structural understanding of how the CLC is accommodated into such a tightly packed amyloid core. Nevertheless, any modulation of the CLC, through proline isomerization or cofactor binding would presumably promote the incorporation of the anti-amyloidogenic CLC. In the context of parallel in register β-sheet models for PrPSc architecture that we have previously proposed,Citation28 cofactors would access the CLC along one side of the amyloid fibril (). This could allow large cofactor molecules, such as glycosaminoglycans, to bind to the CLC, potentially across multiple PrP monomers. Regardless if PrPSc occurs in a parallel in-register β-sheet or another architecture, the incorporation of the CLC and any potential accessory molecules or cofactors into the tightly folded amyloid core must be accommodated.
FIGURE 1. Amyloid formation can be influenced by both amino acid composition and secondary modulation of these amino acid residues. 1) Primary amino acid composition and sequence can influence the propensity for amyloid formation with hydrophobic and aromatic residues promoting amyloid formation and proline and charged residues impeding amyloid formation. The central lysine cluster (CLC) of mammalian PrP includes four lysine and 2 proline residues that prevent PrP amyloid formation. 2) Amino acids can be further modulated by proline isomerization, post-translational modifications, or cofactor binding to alter the amyloid propensity conferred by the primary amino acid sequence. In the case of PrP, polyanionic lipids or glycosaminoglycans (pictured in green) could bind to the CLC (in blue) to neutralize the anti-amyloid effects of the basic CLC lysine residues and promote PrP amyloid formation.
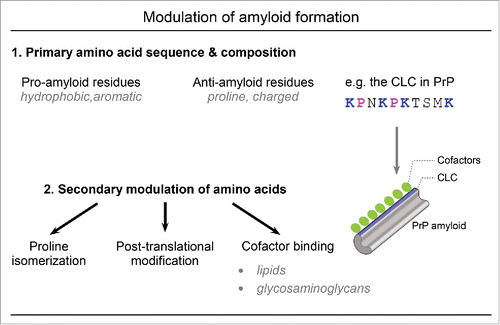
The CLC in PrP represents a highly conserved region with anti-amyloidogenic properties that can largely be attributed to the high proline and lysine amino acid composition. Given the detrimental outcome of PrP amyloid formation in mammals, there is presumably evolutionary interest in preventing pathological amyloid refolding and this may involve specific selection for the proline and lysine residues contained within the CLC. The role of the CLC prolines in predisposition to genetic prion diseases and the CLC in coordinating cofactors that support de novo prion generation suggest that the CLC may represent a critical “switch” region in PrP amyloid formation. Understanding the specific mechanisms by which the CLC influences PrP amyloid formation, with consideration given to proline isomerization, chaperone binding and cofactor coordination at this site may provide critical insights into preventing PrP amyloid formation. Furthermore, elucidation of how the CLC modulates PrP amyloid formation will provide general insights into how the anti-amyloidogenic properties of proline and lysine residues could influence other protein amyloids.
DISCLOSURE OF POTENTIAL CONFLICTS OF INTEREST
No potential conflicts of interest were disclosed.
ACKNOWLEDGMENTS
I would like to thank Drs. Byron Caughey, Clayton Winkler and Bradley Groveman for helpful comments on the manuscript and Anita Mora and Ryan Kissinger for graphics assistance.
FUNDING
This work was supported by the Intramural Research Program of the National Institute of Allergy and Infectious Diseases.
REFERENCES
- Eisenberg D, Jucker M. The Amyloid State of Proteins in Human Diseases. Cell 2012; 148(6): p:1188-203; PMID:22424229; http://dx.doi.org/10.1016/j.cell.2012.02.022
- Tartaglia GG, Pechmann S, Dobson CM, Vendruscolo M. Life on the edge: a link between gene expression levels and aggregation rates of human proteins. Trends Biochem Sci 2007; 32(5): p. 204-6; PMID:17419062; http://dx.doi.org/10.1016/j.tibs.2007.03.005
- Chiti F, Webster P, Taddei N, Clark A, Stefani M, Ramponi G, Dobson CM. Designing conditions for in vitro formation of amyloid protofilaments and fibrils. Proc Natl Acad Sci U S A 1999; 96(7): p:3590-4; PMID:10097081; http://dx.doi.org/10.1073/pnas.96.7.3590
- Dobson CM. Protein misfolding, evolution and disease. Trends.Biochem.Sci 1999; 24(9): p. 329-32; PMID:10470028; http://dx.doi.org/10.1016/S0968-0004(99)01445-0
- Ross ED, Baxa U, Wickner RB. Scrambled prion domains form prions and amyloid. Mol Cell Biol 2004; 24(16): p:7206-13; PMID:15282319; http://dx.doi.org/10.1128/MCB.24.16.7206-7213.2004
- Toombs JA, McCarty BR, Ross ED. Compositional determinants of prion formation in yeast. Mol Cell Biol 2010; 30(1): p:319-32; PMID:19884345; http://dx.doi.org/10.1128/MCB.01140-09
- Paul KR, Hendrich CG, Waechter A, Harman MR, Ross ED. Generating new prions by targeted mutation or segment duplication. Proc Natl Acad Sci U S A, 2015; 112(28): p:8584-9; PMID:26100899; http://dx.doi.org/10.1073/pnas.1501072112
- Gonzalez Nelson AC, Paul KR, Petri M, Flores N, Rogge RA, Cascarina SM, Ross ED. Increasing prion propensity by hydrophobic insertion. PLoS One 2014; 9(2): p:e89286; PMID:24586661; http://dx.doi.org/10.1371/journal.pone.0089286
- Saupe SJ, Daskalov A. The [Het-s] prion, an amyloid fold as a cell death activation trigger. PLoS Pathog 2012; 8(5): p:e1002687; PMID:22654661; http://dx.doi.org/10.1371/journal.ppat.1002687
- Daskalov A, Dyrka W, Saupe SJ. Theme and variations: evolutionary diversification of the HET-s functional amyloid motif. Sci Rep 2015; 5. p:12494; PMID:26219477; http://dx.doi.org/10.1038/srep12494
- Kraus A, Anson KJ, Raymond LD, Martens C, Groveman BR, Dorward DW, Caughey B. Prion protein prolines 102 and 105 and the surrounding lysine cluster impede amyloid formation. J Biol Chem 2015; 290(35):21510-22; PMID:26175152; http://dx.doi.org/10.1074/jbc.M115.665844
- Groveman BR, Kraus A, Raymond LD, Dolan MA, Anson KJ, Dorward DW, Caughey B. Charge neutralization of the central lysine cluster in prion protein (PrP) promotes PrP(Sc)-like folding of recombinant PrP amyloids. J Biol Chem 2015; 290(2): p:1119-28; PMID:25416779; http://dx.doi.org/10.1074/jbc.M114.619627
- Allen B, Ingram E, Takao M, Smith MJ, Jakes R, Virdee K, Yoshida H, Holzer M, Craxton M, Emson PC, et al. Abundant tau filaments and nonapoptotic neurodegeneration in transgenic mice expressing human P301S tau protein. J Neurosci 2002; 22(21): p:9340-51; PMID:12417659
- Iba M, Guo JL, McBride JD, Zhang B, Trojanowski JQ, Lee VM. Synthetic tau fibrils mediate transmission of neurofibrillary tangles in a transgenic mouse model of Alzheimer's-like tauopathy. J Neurosci 2013; 33(3): p:1024-37; PMID:23325240; http://dx.doi.org/10.1523/JNEUROSCI.2642-12.2013
- Eichner T, Radford SE. A generic mechanism of beta2-microglobulin amyloid assembly at neutral pH involving a specific proline switch. J Mol Biol 2009; 386(5): p:1312-26; PMID:19452600; http://dx.doi.org/10.1016/j.jmb.2009.01.013
- Eichner T, Kalverda AP, Thompson GS, Homans SW, Radford SE. Conformational conversion during amyloid formation at atomic resolution. Mol Cell 2011; 41(2): p:161-72; PMID:21255727; http://dx.doi.org/10.1016/j.molcel.2010.11.028
- Brown NR, Noble ME, Endicott JA, Johnson LN. The structural basis for specificity of substrate and recruitment peptides for cyclin-dependent kinases. Nat Cell Biol 1999; 1(7): p. 438-43; PMID:10559988; http://dx.doi.org/10.1038/15674
- Zhou XZ, Kops O, Werner A, Lu PJ, Shen M, Stoller G, Küllertz G, Stark M, Fischer G, Lu KP. Pin1-dependent prolyl isomerization regulates dephosphorylation of Cdc25C and tau proteins. Mol Cell 2000; 6(4): p:873-83; PMID:11090625; http://dx.doi.org/10.1016/S1097-2765(05)00083-3
- Weiwad M, Küllertz G, Schutkowski M, Fischer G. Evidence that the substrate backbone conformation is critical to phosphorylation by p42 MAP kinase. FEBS Lett 2000; 478(1-2): p:39-42; PMID:10922466; http://dx.doi.org/10.1016/S0014-5793(00)01794-4
- Wang F, Wang X, Yuan CG, Ma J. Generating a prion with bacterially expressed recombinant prion protein. Science 2010; 327(5969): p:1132-5; PMID:20110469; http://dx.doi.org/10.1126/science.1183748
- Deleault NR, Piro JR, Walsh DJ, Wang F, Ma J, Geoghegan JC, Supattapone S. Isolation of phosphatidylethanolamine as a solitary cofactor for prion formation in the absence of nucleic acids. Proc Natl Acad Sci U S A 2012; 109(22): p:8546-51; PMID:22586108; http://dx.doi.org/10.1073/pnas.1204498109
- Deleault NR, Harris BT, Rees JR, Supattapone S. Formation of native prions from minimal components in vitro. Proc Natl Acad Sci USA 2007; 104: p:9741-6; PMID:17535913; http://dx.doi.org/10.1073/pnas.0702662104
- Miller MB, Wang DW, Wang F, Noble GP, Ma J, Woods VL, Jr, Li S, Supattapone S. Cofactor molecules induce structural transformation during infectious prion formation. Structure 2013; 21(11): p:2061-8; PMID:24120764; http://dx.doi.org/10.1016/j.str.2013.08.025
- Wang F, Yin S, Wang X, Zha L, Sy MS, Ma J. Role of the highly conserved middle region of prion protein (PrP) in PrP-lipid interaction. Biochemistry 2010; 49(37): p:8169-76; PMID:20718504; http://dx.doi.org/10.1021/bi101146v
- Eichner T, Radford SE. Understanding the complex mechanisms of beta2-microglobulin amyloid assembly. FEBS J 2011; 278(20): p:3868-83; PMID:21595827; http://dx.doi.org/10.1111/j.1742-4658.2011.08186.x
- Gaggelli E, D'Amelio N, Gaggelli N, Valensin G. Metal ion effects on the cis/trans isomerization equilibrium of proline in short-chain peptides: a solution NMR study. Chembiochem 2001; 2(7-8): p:524-9; PMID:11828485; http://dx.doi.org/10.1002/1439-7633(20010803)2:7/8<524::AID-CBIC524>3.0.CO;2-P
- Cox CFD, Marthy NN, Lectka T, Copper(II)-Catalyzed Amide Isomerization: Evidence for N-Coordination. J Am Chem Soc 1996. 118: p:5332-3; http://dx.doi.org/10.1021/ja960693c
- Groveman BR, Dolan MA, Taubner LM, Kraus A, Wickner RB, Caughey B. Parallel in-register intermolecular β-sheet architectures for prion-seeded prion protein (PrP) amyloids. J Biol Chem 2014; 289(35): p:24129-42; PMID:25028516; http://dx.doi.org/10.1074/jbc.M114.578344