ABSTRACT
Mammalian prion proteins (PrPs) that cause transmissible spongiform encephalopathies are misfolded conformations of the host cellular PrP. The misfolded form, the scrapie PrP (PrPSc), can aggregate into amyloid fibrils that progressively accumulate in the brain, evolving to a pathological phenotype. A particular characteristic of PrPSc is to be found as different strains, related to the diversity of conformational states it can adopt. Prion strains are responsible for the multiple phenotypes observed in prion diseases, presenting different incubation times and diverse deposition profiles in the brain. PrP biochemical properties are also strain-dependent, such as different digestion pattern after proteolysis and different stability. Although they have long been studied, strain formation is still a major unsolved issue in prion biology. The recreation of strain-specific conformational features is of fundamental importance to study this unique pathogenic phenomenon. In our recent paper, we described that murine PrP, when expressed in bacteria, forms amyloid inclusion bodies that possess different strain-like characteristics, depending on the PrP construct. Here, we present an extra-view of these data and propose that bacteria might become a successful model to generate preparative amounts of prion strain-specific assemblies for high-resolution structural analysis as well as for addressing the determinants of infectivity and transmissibility.
BACKGROUND
Prions are misfolded, self-perpetuating and infectious entities derived from proteins natively expressed in the host. Once formed, prions can propagate in an auto-catalytic manner, recruiting the normal cellular protein to fold into its abnormal conformation.Citation1,2 Mammalian prion diseases, also termed transmissible spongiform encephalopathies (TSEs), are fatal neurodegenerative disorders that affect humans and other mammals. Despite they have been described since the late 18th century, the recognition that these diseases were caused by infectious proteins became clear only in the early 1980-s, largely due to the work of Stanley Prusiner.Citation3 Prusiner's studies were fueled by the work from Tikvah Alper on the infectious-agent resistance to extreme conditions, such as ionizing radiationCitation4; and also by the initial proposal of the protein-only hypothesis by Griffith in the mid 1960-s.Citation5 Nowadays, a prion-like behavior is also attributed to other proteins involved in neurodegenerative diseases, including Alzheimer's and Parkinson's diseases, besides the classical prion itself (TSEs).Citation6
Therefore, prion pathogenesis seems to be more general than previously thought, and it will likely pass from being the exception to become the general case in protein misfolding diseases (PMDs).
Despite diverging in primary sequence and structure, many misfolded proteins can aggregate into highly-ordered amyloid fibrils leading to the pathological condition called amyloidosis.Citation7 The amyloid state of a protein can convert the cellular form of a protein with the same or very similar primary sequence into its amyloid conformation, ultimately leading to its intracellular aggregation and cell-to-cell transmission.Citation8 The progressive accumulation of the aberrant protein in the neuronal tissue is the hallmark of the neurodegenerative PMDs.
One peculiar characteristic of mammalian prion proteins (PrPs) is the formation of strains, which have been related to the multiple conformations that could be adopted by the pathogenic scrapie PrP (PrPSc).Citation9 Prion strains are responsible for the wide spectrum of phenotypes observed for prion diseases. PrPScstrain isolates, when inoculated into hosts of their respective species, cause disease with different features, i.e. the length of incubation time, the pattern of protein deposition in the brain, and the extension of the histopathological damage.Citation10,11 Once it became evident that the strain properties of PrPSc were encoded by the particular conformation of the aggregated state, many efforts were devoted to recreate prions in vitro, and to study their biochemical and physical characteristics, e.g. their mechanism of aggregation, their structure and their resistance to PK-digestion. A first advance was made when recombinant C-terminal murine prion protein (mPrP89-230) produced in Escherichia coli (E. coli) was polymerized into amyloid aggregates in vitro and then inoculated intracerebrally into transgenic mice expressing PrP89-230. The mice developed neurologic dysfunction and the prion protein in their brain extracts showed resistance to protease digestion. These brain extracts were also able to transmit the disease to other mice but with low levels of infectivity.Citation12 Many subsequent works showed that highly infectious synthetic prions could not be generated in vitro without the addition of cofactors.Citation13,14 In fact, PrP is considered a ‘promiscuous’ protein because of its ability to bind to different classes of macromolecules, such as nucleic acids (NAs), lipids, glycosaminoglycans, or even metallic ions; all these cofactors have been shown to trigger changes in PrP conformation leading to aggregation and toxicity.Citation15-19 It appears that the minimal components necessary to facilitate PrP in vitro conversion and promote de novo prion formation with higher degree of infectivity are polyanions, mainly nucleic acid (NAs) molecules or lipids.Citation20-22 The challenging task of creating recombinant prions that hold strain-like conformational characteristics and infectivity responds to the difficulty in reproducing an in vitro environment with proper facilitating factors, such as molecular crowding, the presence of chaperones and proteases, continuous synthesis of the protein in the ribosome or the presence of interacting NAs or lipids. In this context, modeling prion amyloid formation inside simple organisms might provide a system to recapitulate prion protein aggregation under more biologically relevant conditions.Citation23
In many instances, heterologous protein expression in bacteria results high intracellular protein concentration, where the aggregation pathway tend to dominate over the folding one, and thus insoluble deposits of the recombinant protein are formed. Different studies showed that amyloidogenic proteins, when expressed in bacteria, form inclusion bodies with common structural and functional features with the highly ordered aggregated amyloids found in the original host species.Citation24,25
The first characterization of the amyloid properties of the bacterial IBs formed by a prionic protein was that of the fungal HET-S prion protein.Citation26 Transmission electron microscopy (TEM) combined with solid-state NMR spectroscopy revealed that E. coli IBs of the HET-s prion forming domain consist of fibrillar structures that are almost identical to the molecular structure of HET-s amyloid fibrils assembled in vitro; and these HET-s IBs also showed prion infectivity when transfected into the natural host.Citation26 Another report provided clear evidence that the bacterial cytoplasm can support the formation of infectious prion aggregates, as shown for the NM-Sup35 yeast protein.Citation27 The NM-Sup35 bacterial IBs where later shown to possess amyloid-like properties. The infectivity of these aggregates was modulated by the environmental conditions in which they were formed.Citation28 Importantly, the NM-Sup35 prion conformation can be propagated in bacteria for over a hundred generations, even when the cells can no longer produce the protein that serves as the trigger for the initial conversion.Citation19 Seminal work from Giraldo's group showed that N-terminal domain of a bacterial protein, RepA, could form amyloid-like fibrils within bacteria upon interaction with defined double-stranded DNA sequences.Citation29 This protein, when modified, generated a prion-like disease in bacteria that can be transmitted from mother to daughter cells.Citation30 More recently, this group showed that this bacterial prionoid can seed amyloid aggregation of mutants from the same protein,Citation31 and characterized the bacterial nucleoid as the initial site of assemble of these prion-like aggregates.Citation32 This bacterial amyloidosis, shared some characteristics with mammalian prion diseases, mainly transmissibility and the presence of phenotypically distinct strains.
Altogether, those observations provided solid evidences that the IBs molecular structure can resemble the architecture of amyloid fibrils, in such a way that both the prion strain phenomenon and the infectious nature, which are dependent on the specific protein conformational state, seem to be conserved in these morphologically different aggregates deposits. A remaining question is whether, like their fungal counterparts, mammalian prions could form amyloid intracellular aggregates when expressed in bacteria. We have answered this question in our recent paperCitation33 where we selected two mammalian prion constructs, the wild-type murine PrP encompassing residues 23-231 (PrPWT) and the C-terminal domain of murine PrP (PrP90–231), and expressed them in bacteria. As expected, recombinant PrPs accumulate in the insoluble bacterial fraction as IBs. We purified and studied the conformational characteristics of the PrPs IBs, as well as their biochemical strain-like properties. Our recent study provides the first demonstration that PrPs from mice can form amyloids inside bacterial IBs with common characteristics to PrP amyloids formed in vitro or even the amyloid PrPSc in vivo. Moreover, although possessing similar secondary structure, PrPWT IBs and PrP90-231 IBs exhibit conformational diversity, as they bind to amyloid dyes (Congo red and thioflavin T) at different levels, possess different morphology, different stability against urea-induced unfolding and also different resistance to proteinase K digestion. It is known that amyloid spread requires a high degree of sequential and conformational specificity; only amyloids with sequence identity can form cross-β-sheet interactions able to be incorporated into the amyloid fibrils. This sequential/conformational specificity is probably responsible for the species barrier between two different prions. However, the rules dictating the species barriers as well as effects of the environmental conditions on that barrier are still not clear. In our study, we show that the PrPWT amyloid IBs are able to seed in vitro the amyloid polymerization of purified recombinant PrPWT, reducing the lag-phase of the sigmoidal reaction. But, interestingly, when we tried to seed the same reaction with the PrP90-231 IBs, we could not accelerate the polymerization, which indicates that, despite the fact that the 2 PrP forms share ˜70% of the sequence, the lack of sequential identity at the N-terminus and/or the different structural properties of the aggregates, preclude the establishment of appropriate protein-protein contacts. Finally, the conformational differences among the PrPs IBs result in different toxicity of the 2 PrP IBs resistant cores after PK-digestion when added to neuroblastoma-cultured cells. We believe that the intracellular aggregates retain strain-like features, a characteristic unique for prion proteins (from yeast and mammals). We show that some of these features, such as different migration pattern after digestion with proteinase-K, and different stability are conserved after IBs extraction ().
Figure 1. Mammalian PrP IBs formation in bacteria and their characterization by different techniques. Two constructs of murine PrP were selected (1) and E. coli cells were transformed with plasmids harboring either PrPWT or PrP90-231 cDNAs (2). Induction of recombinant expression of both PrPs resulted in their accumulation in the insoluble bacterial fraction, known as IBs, which were purified upon appropiated cell lysis and extensive washing (3). SDS-PAGE analysis revealed that both PrP IBs are constituted mainly by the recombinant mammalian PrPs (4). Transmission electron microscopy, binding to amyloid-specific dyes and FTIR spectroscopy analysis of isolated PrPs IBs revealed that mammalian PrPs IBs possess an amyloid-like nature (5). Furthermore, digestion with proteinase-K (PK), seeding of the amyloid polymerization reaction and stability studies (6), indicate that these IBs may display prion strain-like properties. It remains to be investigated whether the isolated mammalian PrPs IBs behave as true infectious entities upon intracerebral injection in mice. Accordingly, clinical and histopathological features, as well as transmissibility will be followed to confirm the development of prion disease characteristics in animal models.
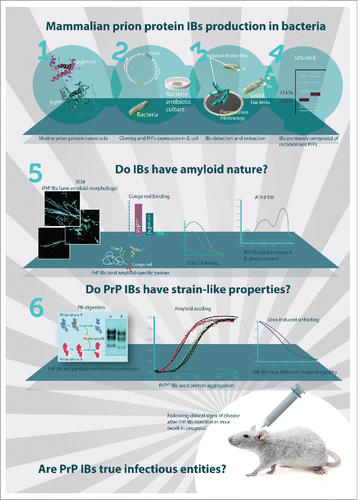
APPLICATIONS OF BACTERIALLY GENERATED MAMMALIAN PRION AMYLOIDS
There are several proposals for the structure of infectious PrPSc, but to date there is still no consensus about the 3D high-resolution conformation of the scrapie PrP.Citation34-36 One of the main challenges is to obtain homogeneous material from infected brain in sufficient amount for the structural studies. Besides, there are also safety limitations for the study of bona fide infectious mammalian prions. Although there are obvious differences in post-translational changes in the prokaryotic and eukaryotic cytoplasm, the generated recombinant prion proteins might become a valuable source of homogeneous but conformationally different assemblies produced with high yield for future transmissibility and structural studies. Besides, our bacteria-generated mammalian PrPs might be useful as seeds for amplification of strain-specific prions and their subsequent study, in protocols such as PMCA (protein misfolding cyclic amplification) and RT-QuIC (real time quaking induced conversion).Citation37,38 Our prion-forming system can also be used for the screening of drugs that inhibit aggregation and amyloid formation, as already proposed for other amyloid proteins.Citation23
CONCLUSION AND PERSPECTIVES
Our previous work provided clear experimental evidence that yeast prions can form amyloids in prokaryotes that can be transmitted to naïve yeasts that will gain the prion-state, confirming infectivity.Citation28 Recently, we demonstrated that modeling mammalian PrP amyloids inside bacteria might be a useful tool to understand the conformational variability of prions. We showed that the IBs obtained upon expression of mammalian PrP in bacteria contain seeding competent amyloid-like structures. We need to get now a more detailed picture about the neurotoxic activity of these IBs. It remains to be confirmed in vivo if we are recapitulating a real prion strain phenomenon. We are performing in vivo experiments to check if PrP IBs can trigger the conversion of host PrP into an aggregated state that retains the PK-resistance and the stability profiles of the seed. Our next study will answer whether bacteria-produced mammalian PrP IBs have only amyloid nature or are indeed infectious to mammals and can be considered bona fide prions (). For that purpose, PrP IBs will be administered to healthy transgenic mice by intracerebral injection and the animals will be evaluated for disease progression. Clinical and histopathological parameters will be followed to identify prion disease characteristics. If clinical symptoms of TSE appear, disease-incubation time and western blot analysis of brain-derived material will be assessed to see whether they correlate with strain-like properties of different inoculated IBs.
Overall, despite speculative, the possibility that mammalian prion amyloid assemblies generated in bacteria would display transmissible and infectious properties in mammalian hosts is worth to be explored, since it would open a myriad of novel opportunities to study the structure and biology of these intriguing proteins.
ABBREVIATIONS
IB | = | inclusion body |
PrP | = | prion protein |
TSE | = | transmissible spongiform encephalopathy |
DISCLOSURE OF POTENTIAL CONFLICTS OF INTEREST
No potential conflicts of interest were disclosed.
Funding
This work was supported by Ministry of Education of Brazil [CAPES process number: 99999.002869/2014-04 to the fellow student B.M]; and by Ministerio de Economia y Competividad, Spain [BFU2013-44763-P to S.V.]; by ICREA [ICREA Academia 2009 to S.V.] and by FAPERJ, CNPq and INBEB from Brazil to Y.C.
REFERENCES
- Prusiner SB, Prusiner SB, Scott MR, Scott MR, DeArmond SJ, DeArmond SJ, Cohen FE, Cohen FE. Prion protein biology-review. Cell 1998; 93:337-48; PMID:9590169; http://dx.doi.org/10.1016/S0092-8674(00)81163-0.
- Caughey B, Baron GS, Chesebro B, Jeffrey M. Getting a grip on prions: oligomers, amyloids, and pathological membrane interactions. Annu Rev Biochem 2009; 78:177-204; PMID:19231987; http://dx.doi.org/10.1146/annurev.biochem.78.082907.145410.
- Prusiner SB. Novel proteinaceous infectious particles cause scrapie. Science 1982; 216:136-44; PMID:6801762; http://dx.doi.org/10.1126/science.6801762.
- Alper T, Cramp WA, Haig DA, Clarke MC. Does the agent of scrapie replicate without nucleic acid? Nature 1967; 214:764-6; PMID:4963878; http://dx.doi.org/10.1038/214764a0
- Griffith JS. Nature of the scrapie agent: self-replication and scrapie. Nature 1967; 215:1043-4; PMID:4964084; http://dx.doi.org/10.1038/2151043a0
- Brettschneider J, Tredici K Del, LeeVM-Y, Trojanowski JQ. Spreading of pathology in neurodegenerative diseases: a focus on human studies. Nat Rev Neurosci 2015; 16:109-20; PMID:25588378; http://dx.doi.org/10.1038/nrn3887.
- Chiti F, Dobson CM. Protein misfolding, functional amyloid, and human disease. Annu Rev Biochem 2006; 75:333-66; PMID:16756495; http://dx.doi.org/10.1146/annurev.biochem.75.101304.123901.
- Guo JL, Lee VMY. Cell-to-cell transmission of pathogenic proteins in neurodegenerative diseases. Nat Med 2014; 20:130-8; PMID:24504409; http://dx.doi.org/10.1038/nm.3457.
- Baskakov IV. Switching in amyloid structure within individual fibrils: Implication for strain adaptation, species barrier and strain classification. FEBS Lett 2009; 583:2618-22; PMID:19482025; http://dx.doi.org/10.1016/j.febslet.2009.05.044.
- Bruce ME, McConnell I, Fraser H, Dickinson AG. The disease characteristics of different strains of scrapie in Sinc congenic mouse lines: implications for the nature of the agent and host control of pathogenesis. J Gen Virol 1991; 72:595-603.
- Hecker R, Taraboulos A, Scott M, Pan KM, Yang SL, Torchia M, Jendroska K, DeArmond SJ, Prusiner SB. Replication of distinct scrapie prion isolates is region specific in brains of transgenic mice and hamsters. Genes Dev 1992 6:1213-28; PMID:1628828; http://dx.doi.org/10.1101/gad.6.7.1213.
- Legname G, Baskakov IV, Nguyen H-OB, Riesner D, Cohen FE, DeArmond SJ, Prusiner SB. Synthetic mammalian prions. Science 2004; 305:673-6; PMID:15286374; http://dx.doi.org/10.1126/science.1100195.
- Makarava N, Kovacs GG, Savtchenko R, Alexeeva I, Budka H, Rohwer RG, Baskakov I V. Genesis of mammalian prions: from non-infectious amyloid fibrils to a transmissible prion disease. PLoS Pathog 2011 7:e1002419; PMID:22144901; http://dx.doi.org/10.1371/journal.ppat.1002419.
- Makarava N, Kovacs GG, Savtchenko R, Alexeeva I, Ostapchenko VG, Budka H, Rohwer RG, Baskakov I V. A new mechanism for transmissible prion diseases. J Neurosci 2012; 32:7345-55; PMID:22623680; http://dx.doi.org/10.1523/JNEUROSCI.6351-11.2012.
- Cordeiro Y, Machado F, Juliano L, Juliano MA, Brentani RR, Foguel D, Silva JL. DNA converts cellular prion protein into the β-Sheet conformation and inhibits prion peptide aggregation. J Biol Chem 2001; 276:49400-9; PMID:11604397; http://dx.doi.org/10.1074/jbc.M106707200.
- Macedo B, Millen TA, Braga CACA, Gomes MPB, Ferreira PS, Kraineva J, Winter R, Silva JL, Cordeiro Y. Nonspecific prion protein-nucleic acid interactions lead to different aggregates and cytotoxic species. Biochemistry 2012; 51:5402-13; PMID:22691027; http://dx.doi.org/10.1021/bi300440e.
- Critchley P, Kazlauskaite J, Eason R, Pinheiro TJT. Binding of prion proteins to lipid membranes. Biochem Biophys Res Commun 2004; 313:559-67; PMID:14697227; http://dx.doi.org/10.1016/j.bbrc.2003.12.004.
- Vieira TCRG, Reynaldo DP, Gomes MPB, Almeida MS, Cordeiro Y, Silva JL. Heparin binding by murine recombinant prion protein leads to transient aggregation and formation of rna-resistant species. J Am Chem Soc 2011; 133:334-44; PMID:21142149; http://dx.doi.org/10.1021/ja106725p.
- Yuan AH, Garrity SJ, Nako E, Hochschild A. Prion propagation can occur in a prokaryote and requires the ClpB chaperone. Elife 2014; 3:e0294910.7554:eLife.02949; PMID:25122461.
- Deleault NR, Harris BT, Rees JR, Supattapone S. Formation of native prions from minimal components in vitro. Proc Natl Acad Sci U S A 2007; 104:9741-6; PMID:17535913; http://dx.doi.org/10.1073/pnas.0702662104.
- Wang F, Wang X, Yuan C-G, Ma J. Generating a prion with bacterially expressed recombinant prion protein. Science 2010; 327:1132-5; PMID:20110469; http://dx.doi.org/10.1126/science.1183748.
- Miller MB, Wang DW, Wang F, Noble GP, Ma J, Woods VL, Li S, Supattapone S. Cofactor molecules induce structural transformation during infectious prion formation. Structure 2013; 21:2061-8; PMID:24120764; http://dx.doi.org/10.1016/j.str.2013.08.025.
- Villar-Piqué A, Ventura S. Modeling amyloids in bacteria. Microb Cell Fact 2012; 11:166; http://dx.doi.org/10.1186/1475-2859-11-166.
- Morell M, Bravo R, Espargaró A, Sisquella X, Avilés FX, Fernàndez-Busquets X, Ventura S. Inclusion bodies: Specificity in their aggregation process and amyloid-like structure. Biochim Biophys Acta - Mol Cell Res 2008; 1783:1815-25; http://dx.doi.org/10.1016/j.bbamcr.2008.06.007.
- de Groot NS, Sabate R, Ventura S. Amyloids in bacterial inclusion bodies. Trends Biochem Sci 2009; 34:408-16.
- Wasmer C, Benkemoun L, Sabaté R, Steinmetz MO, Coulary-Salin B, Wang L, Riek R, Saupe SJ, Meier BH. Solid-state NMR spectroscopy reveals that E. coli inclusion bodies of HET-s(218-289) are amyloids. Angew Chem Int Ed Engl 2009; 48:4858-60; PMID:19472238; http://dx.doi.org/10.1002/anie.200806100.
- Garrity SJ, Sivanathan V, Dong J, Lindquist S, Hochschild A. Conversion of a yeast prion protein to an infectious form in bacteria. Proc Natl Acad Sci U S A 2010; 107:10596-601; PMID:20484678; http://dx.doi.org/10.1073/pnas.0913280107.
- Espargaró A, Sabate R, Ventura S. Thioflavin-S staining coupled to flow cytometry. A screening tool to detect in vivo protein aggregation. Mol. Biosyst 2012; 8:2839; http://dx.doi.org/10.1039/c2mb25214g.
- Giraldo R. Defined DNA sequences promote the assembly of a bacterial protein into distinct amyloid nanostructures. Proc Natl Acad Sci U S A 2007 104:17388-93; PMID:17959784; http://dx.doi.org/10.1073/pnas.0702006104.
- Giraldo R, Moreno-Díaz de la Espina S, Fernández-Tresguerres ME, Gasset-Rosa F. RepA-WH1 prionoid: a synthetic amyloid proteinopathy in a minimalist host. Prion 2011; 5:60-4; PMID:21293179; http://dx.doi.org/10.4161/pri.5.2.14913.
- Molina-García L, Giraldo R. Aggregation interplay between variants of the RepA-WH1 prionoid in Escherichia coli. J Bacteriol 2014; 196:2536-42; http://dx.doi.org/10.1128/JB.01527-14.
- Moreno-Del Álamo M, de la Espina SM-D, Fernández-Tresguerres ME, Giraldo R. Pre-amyloid oligomers of the proteotoxic RepA-WH1 prionoid assemble at the bacterial nucleoid. Sci Rep 2015; 5:14669; PMID:26423724; http://dx.doi.org/10.1038/srep14669.
- Macedo B, Sant'Anna R, Navarro S, Cordeiro Y, Ventura S. Mammalian prion protein (PrP) forms conformationally different amyloid intracellular aggregates in bacteria. Microb Cell Fact 2015; 14:174; PMID:26536866; http://dx.doi.org/10.1186/s12934-015-0361-y.
- Govaerts C, Wille H, Prusiner SB, Cohen FE. Evidence for assembly of prions with left-handed β-helices into trimers. Proc Natl Acad Sci U S A 2004; 101:8342-7; PMID:15155909; http://dx.doi.org/10.1073/pnas.0402254101.
- Diaz-Espinoza R, Soto C. High-resolution structure of infectious prion protein: the final frontier. Nat Struct Mol Biol 2012; 19:370-7; PMID:22472622; http://dx.doi.org/10.1038/nsmb.2266.
- Groveman BR, Dolan MA, Taubner LM, Kraus A, Wickner RB, Caughey B. Parallel in-register intermolecular β-sheet architectures for prion-seeded prion protein (PrP) amyloids. J Biol Chem 2014; 289:24129-42; PMID:25028516; http://dx.doi.org/10.1074/jbc.M114.578344.
- Saborio GP, Permanne B, Soto C. Sensitive detection of pathological prion protein by cyclic amplification of protein misfolding. Nature 2001; 411:810-3; PMID:11459061; http://dx.doi.org/10.1038/35081095.
- Wilham JM, Orrú CD, Bessen RA, Atarashi R, Sano K, Race B, Meade-White KD, Taubner LM, Timmes A, Caughey B. Rapid end-point quantitation of prion seeding activity with sensitivity comparable to bioassays. PLoS Pathog 2010; 6(12):e1001217.