ABSTRACT
Temporal and spatial patterns of pathological changes such as loss of neurons and presence of pathological protein aggregates are characteristic of neurodegenerative diseases such as Amyotrophic Lateral Sclerosis, Frontotemporal Dementia, Alzheimer's disease and Parkinson's disease. These patterns are consistent with the propagation of protein misfolding and aggregation reminiscent of the prion diseases. There is a surge of evidence that suggests that large protein aggregates of a range of proteins are able to enter cells via macropinocytosis. Our recent work suggests that this process is activated by the binding of aggregates to the neuron cell surface. The current review considers the potential role of cell surface receptors in the triggering of macropinocytosis by protein aggregates and the possibility of utilizing macropinocytosis pathways as a therapeutic target.
Neurodegenerative diseases such as Amyotrophic Lateral Sclerosis (ALS), Frontotemporal Dementia (FTD), Alzheimer's disease, and Parkinson's disease have temporal and spatial patterns of pathological changes such as loss of neurons and presence of pathological protein aggregates.Citation1-4 The patterns that have been identified in neuropathological studies are have been suggested to be consistent with propagation of protein misfolding and aggregation reminiscent of the prion diseases.Citation5
Evidence from in vitro studies suggests that large protein aggregates of a range of proteins (SOD1, tau, α-synuclein, and proteins containing expanded polyglutamine repeats) are able to enter cells via an incompletely understood mechanism and propagate misfolding and aggregation.Citation6-14 Animal studies demonstrate that injection of brain or spinal cord extracts from transgenic mice expressing human tau, SOD1 or α-synuclein can seed pathology in transgenic mice at the sites of injection and initiate spread of pathology to other regions of the nervous system.Citation14-17 These data combined with the patterns of neurodegeneration provide strong evidence of prion-like protein misfolding propagation in neurodegenerative diseases such as Parkinson's disease and ALS. The precise mechanism of cell-to-cell propagation of protein misfolding in vivo remains unclear. There are several possible scenarios that may explain the propagation of protein misfolding, the most commonly proposed of which is the release of protein aggregates in to the extracellular space and subsequent uptake in to nearby or connected naïve neurons where seeding can then take place. In this model both aggregate release and subsequent uptake are requisite. Misfolded or aggregated material may be released from cells via an active mechanism, either within exosomes or naked, or alternatively, aggregates may be passively released from dead or dying cells. In reality, within a complex biological system it is likely that the release of aggregated protein may be a combination of each of the possible release mechanisms. This makes therapeutic targeting of aggregate release difficult, moreover, reducing the burden of misfolded protein by active release may be beneficial to the cell and thus its blockade detrimental. One aspect of protein misfolding propagation that may provide a more tractable therapeutic target is the entry of protein aggregates in to cells.
Work to date suggests that macropinocytosis may be responsible for the uptake of protein aggregates associated with various neurodegenerative diseases.Citation6,11,18-20 A range of studies examining several different types of protein aggregates, including those made from α-synuclein, Huntingtin (exon1Q44), and tau are consistent with an unconventional, bulk endocytosis route being responsible for their uptake.Citation12,18,21-24 A systematic study using SOD1 aggregates by Münch et. al conlcuded that protein aggregates enter into N2A cells via macropinocytosis.Citation6 These findings were subsequently corroborated in work that showed uptake of both extracellular wildtype and mutant SOD1 soluble forms into NSC-34 cells can be inhibited by small molecule inhibitors of macropinocytosis EIPA and rottlerin.Citation11,19 EIPA (5-(N-Ethyl-N-isopropyl)amiloride) is an amiloride analog that inhibits Na+/H+ exchangers which suppresses macropinocytosis due to the vulnerability of GTPase Rac1 to pH changes.Citation25 However, the small molecule inhibitors generally utilized to define macropinocytosis, such as EIPA, are not specific and have been shown to prevent various forms of endocytosis.Citation26 As a result, further work is required to more precisely define the precise mode of endocytosis responsible for protein aggregate uptake.
Macropinocytosis is a type of endocytosis that is partly defined by pinosome size, macropinosomes can be several micron in diameter. Macropinocytosis can be constitutive or a transient process, generally triggered by growth factors but has been observed to be activated by particles such as bacteria, apoptotic bodies and viruses.Citation27 Its activation leads to formation of large membrane ruffles that can fold back on the cell allowing the internalisation of fluid, membrane and other particles into large vacuoles several micron in diameter.Citation28 The role of stimulation of macropinocytosis in aggregate uptake remains unclear.
Our recent work shows that SOD1 aggregates can bind to the cell surface and trigger activation of membrane ruffling in NSC-34 cells and iPSC generated human neuronsCitation20 consistent with stimulated rather than constitutive macropinocytosis. The engulfment of aggregates in to macropinosomes could explain the ease by which aggregates enter the cytosol. Macropinosomes are considered ‘leaky’ due to their lack of physical structure compared with coated vesicles.Citation29 Indeed, we observed vesicle rupture upon entry of SOD1 aggregates in to NSC-34 cells.Citation20 A characteristic signaling molecule associated with actin mobilization during macropinocytosis in the Rho GTPase Rac1. We found that application of SOD1 aggregates to NSC-34 cells resulted in an increase in the amount of activated Rac1, and also observed that the uptake of aggregated SOD1 in to NSC-34 and human iPSC derived motor neurons was suppressed by the Rac1 inhibitor W56.Citation20 These data are consistent with a triggering of macropinocytosis that involves an activation of Rac1. Moreover, our data indicate that aggregates made from other proteins such as α-synuclein, huntingtin-polyQ and TDP-43 also trigger membrane ruffling and macropinocytosis.Citation20 Indeed, this seems to be a generic response to cell surface interaction with protein aggregates since aggregates made from α-lactalbumin, that are not involved with disease, also trigger this response.
The question of how protein aggregates trigger macropinocytosis remains unanswered. To begin to shed light on the answer to this question one can turn to the virus uptake literature as the mechanisms of macropinocytosis activation have been more closely studied in this context. There are a several virusesCitation27 that have been shown to utilize macropinocytosis pathways to enter cells, including the Japanese encephalitis virus in neurons.Citation30 Receptor complexes on the cell surface are vital to the binding of viral particles and activation of macropinocytosis. Integrins such as β1,Citation31 αv,Citation32 and the heparan sulfate proteoglycansCitation33 have been reported to be involved in particle binding, while receptor tyrosine kinases such as ErbB-134 and EphA2Citation35 have been implicated in the triggering of the signaling cascade that stimulates macropinocytosis (). Little is known about the receptors that mediate protein aggregate induced macropinocytosis in neurons. However, heparan sulfate proteoglycans have been identified as an important part of the mechanism that allows tau, α-synuclein and prion protein aggregate uptakeCitation18,36,37 suggesting that the receptor complexes involved may be similar to that involved in virus particle uptake.
FIGURE 1. Cell surface receptors responsible for macropinocytosis and protein aggregate recognition. A) Receptor tyrosine kinases (RTK), integrins (INT) and heparan sulfate proteoglycans (HS) have been associated with the viral particle recognition and subsequent triggering of macropinocytosis. B) Scavenger receptors (SR), RAGE, integrins (INT), CD14 and toll like receptors (TLR) have all been found to play a role in recognition and subsequent proinflammatory response to protein aggregates of amyloid β peptide. C) Neurons have been shown to express scavenger receptors (SR), receptor tyrosine kinases (RTK), integrins (INT) and heparan sulfate proteoglycans which may be involved in triggering of macropinocytosis by protein aggregates.
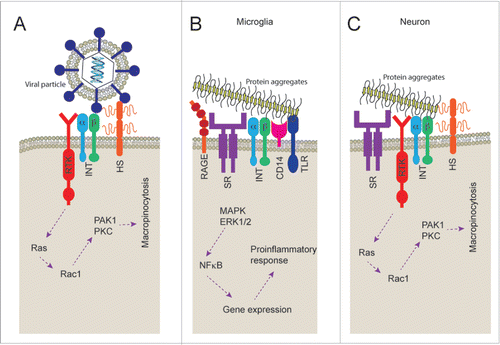
While little is known about the neuronal receptor complexes associated with binding of protein aggregates, a wide range of receptors have been shown to be involved in the binding of protein aggregates to the cell surface of microglia.Citation38,39 A group of receptors, known as pattern recognition receptors, are often responsible for this process. These receptors bind to pathogen associated molecular patterns (PAMPs) and danger associated molecular patterns (DAMPs) that may be responsible for the recognition of protein aggregates. It is possible that these pattern recognition receptors bind to the repetitive patterns found on aggregates formed from various proteins, indeed the fibrillar structure of amyloid fibrils are thought to have a generic core structure. The binding of particles to these microglial receptor complexes results in activation of signaling cascades, including activation of MAP kinase and ERK1/2, subsequently resulting in cytokine release.Citation40 Scavenger receptors CD36Citation41 and SR-A,Citation42 the receptor for advanced glycation end products (RAGE),Citation43,44 integrin α6β1-integrin and integrin associated CD47,Citation39 formyl peptide receptor 2/formyl peptide receptor-like 1 (FPR2/FPRL1),Citation45 CD33,Citation46 CD14 and Toll-like receptors (TLR) 2/4Citation47 have all been shown to be involved in microglial binding of protein aggregates made of Aβ peptide. In addition, we have previously shown that microglia binding of aggregated SOD1 is supressed by pre-treatment with methyl-β-cyclodextrin (MβCD), fucoidan and lipopolysaccharide (LPS) suggesting that binding is dependent on lipid raft formation, scavenger receptors and CD14.Citation48 The binding of fibrils to microglial cell surface is mediated not by individual receptors but though large receptor complexes () involving signaling receptors such as TLR2/4. This process of receptor complex formation, in some cases, is dependent on lipid raft formation to allow membrane protein organization.Citation49
Neurons express heparan sulfate proteoglycans,Citation50 RAGE,Citation51 CD36,Citation52 integrinsCitation32 and receptor tyrosine kinases such as EphA2 and EphA4.Citation53 This is consistent with neurons having all the machinery to bind aggregates and to trigger macropinocytosis with a receptor complex similar to that involved in viral uptake and somewhat similar to that of microglial cell surface recognition (). Indeed, a macropinocytosis-like process regulates growth cone membrane recycling, growth cone collapse, axon retraction and turning during development and injury.Citation54-58 Future work will be needed to further characterize the composition of the neuron specific set of cell surface proteins that are responsible for the binding, triggering of macropinocytosis and uptake of protein aggregates. Given that blocking all macropinocytosis in the brain, including microglial and astrocytic, may be detrimental this knowledge could be used to define a specific target to block neuronal uptake of protein aggregates. Blocking uptake may effectively supress propagation of protein aggregation, as the cytoplasmic invasion of protein aggregates, like those of SOD1, are a necessary part of the prion-like propagation process. However, due to the role of a macropinocytic process in growth cone and axon developmentCitation54-58 the effect of inhibition of macropinocytosis on neurogenesis should be studied. Regardless, a receptor tyrosine kinase EphA4 has been implicated in ALS disease progression. In humans with ALS, EphA4 expression was found to inversely correlate with disease onset and survival, and loss-of-function mutations in EPHA4 were found to be associated with slower disease progression and longer survival.Citation59 It is tempting to speculate that EphA4 might contribute to the activation of macropinocytosis and uptake of protein aggregates, which might be slowed in its absence or inhibition. Identifying such a target that will supress the neuronal macropinocytosis of protein aggregates but will allow the microglial phagocytosis and degradation of such particles is vital. Blocking all endocytosis of aggregates may be detrimental.
In summary, propagation of protein misfolding and aggregation is implicated in the progressive nature of several neurodegenerative diseases such as Alzheimer's disease, Parkinson's disease and ALS. In order for propagation to proceed aggregates must be able to gain access to the cytosol of naïve neurons and current evidence suggests that stimulated macropinocytosis is responsible for this uptake. The receptor complexes responsible for this are still to be defined, but this information may provide therapeutic targets that could halt or slow the spread of pathology.
DISCLOSURE OF POTENTIAL CONFLICTS OF INTEREST
No potential conflicts of interest were disclosed.
REFERENCES
- Braak H, Alafuzoff I, Arzberger T, Kretzschmar H, Del Tredici K. Staging of Alzheimer disease-associated neurofibrillary pathology using paraffin sections and immunocytochemistry. Acta Neuropathologica 2006; 112(4): p:389-404; PMID:16906426; http://dx.doi.org/10.1007/s00401-006-0127-z
- Braak H, Del Tredici K, Rub U, de Vos RA, Jansen Steur EN, Braak E. Staging of brain pathology related to sporadic Parkinson's disease. Neurobiol Aging 2003; 24(2): p:197-211; PMID:12498954; http://dx.doi.org/10.1016/S0197-4580(02)00065-9
- Brettschneider J, Del Tredici K, Irwin DJ, Grossman M, Robinson JL, Toledo JB, Fang L, Van Deerlin VM, Ludolph AC, Lee VM, et al. Sequential distribution of pTDP-43 pathology in behavioral variant frontotemporal dementia (bvFTD). Acta Neuropathologica 2014; 127(3):423-39
- Brettschneider J, Del Tredici K, Toledo JB, Robinson JL, Irwin DJ, Grossman M, Suh E, Van Deerlin VM, Wood EM, Baek Y, et al. Stages of pTDP-43 pathology in amyotrophic lateral sclerosis. Annals Neurol 2013; 74(1): p:20-38; http://dx.doi.org/10.1002/ana.23937
- Aguzzi A. Cell biology: Beyond the prion principle. Nature 2009; 459(7249): p:924-5; PMID:19536253; http://dx.doi.org/10.1038/459924a
- Münch C, O'Brien J, Bertolotti A. Prion-like propagation of mutant superoxide dismutase-1 misfolding in neuronal cells. Proc Natl Acad Sci U S A 2011; 108(9):3548-53; PMID:21321227; http://dx.doi.org/10.1073/pnas.1017275108
- Furukawa Y, Kaneko K, Watanabe S, Yamanaka K, Nukina N. Intracellular seeded aggregation of mutant Cu,Zn-superoxide dismutase associated with amyotrophic lateral sclerosis. FEBS Lett 2013; 587(16): p:2500-5; PMID:23831581; http://dx.doi.org/10.1016/j.febslet.2013.06.046
- Hansen C, Angot E, Bergstrom AL, Steiner JA, Pieri L, Paul G, Outeiro TF, Melki R, Kallunki P, Fog K, et al. α-Synuclein propagates from mouse brain to grafted dopaminergic neurons and seeds aggregation in cultured human cells. J Clin Invest 2011; 121(2): p:715-25; PMID:21245577; http://dx.doi.org/10.1172/JCI43366
- Volpicelli-Daley LA, Luk KC, Patel TP, Tanik SA, Riddle DM, Stieber A, Meaney DF, Trojanowski JQ, Lee VM. Exogenous α-synuclein fibrils induce Lewy body pathology leading to synaptic dysfunction and neuron death. Neuron 2011; 72(1): p:57-71; PMID:21982369; http://dx.doi.org/10.1016/j.neuron.2011.08.033
- Guo JL, Lee VM. Neurofibrillary tangle-like tau pathology induced by synthetic tau fibrils in primary neurons over-expressing mutant tau. FEBS Lett 2013; 587(6): p:717-23; PMID:23395797; http://dx.doi.org/10.1016/j.febslet.2013.01.051
- Grad LI, Yerbury JJ, Turner BJ, Guest WC, Pokrishevsky E, O'Neill MA, Yanai A, Silverman JM, Zeineddine R, Corcoran L, et al. Intercellular propagated misfolding of wild-type Cu/Zn superoxide dismutase occurs via exosome-dependent and -independent mechanisms. Proc Natl Acad Sci U S A 2014; 111(9): p:3620-5; PMID:24550511; http://dx.doi.org/10.1073/pnas.1312245111
- Desplats P, Lee HJ, Bae EJ, Patrick C, Rockenstein E, Crews L, Spencer B, Masliah E, Lee SJ. Inclusion formation and neuronal cell death through neuron-to-neuron transmission of α-synuclein. Proc Natl Acad Sci U S A 2009; 106(31): p:13010-5; PMID:19651612; http://dx.doi.org/10.1073/pnas.0903691106
- Ren PH, Lauckner JE, Kachirskaia I, Heuser JE, Melki R, Kopito RR. Cytoplasmic penetration and persistent infection of mammalian cells by polyglutamine aggregates. Nat Cell Biol 2009; 11(2): p:219-25; PMID:19151706; http://dx.doi.org/10.1038/ncb1830
- Clavaguera F, Bolmont T, Crowther RA, Abramowski D, Frank S, Probst A, Fraser G, Stalder AK, Beibel M, Staufenbiel M, et al. Transmission and spreading of tauopathy in transgenic mouse brain. Nat Cell Biol 2009; 11(7): p:909-13; PMID:19503072; http://dx.doi.org/10.1038/ncb1901
- Ayers JI, Fromholt S, Koch M, DeBosier A, McMahon B, Xu G, Borchelt DR. Experimental transmissibility of mutant SOD1 motor neuron disease. Acta Neuropathol 2014; 128(6): p:791-803; PMID:25262000; http://dx.doi.org/10.1007/s00401-014-1342-7
- Mougenot AL, Nicot S, Bencsik A, Morignat E, Verchere J, Lakhdar L, Legastelois S, Baron T. Prion-like acceleration of a synucleinopathy in a transgenic mouse model. Neurobiol Aging 2012; 33(9): p:2225-8; PMID:21813214; http://dx.doi.org/10.1016/j.neurobiolaging.2011.06.022
- Ayers JI, Fromholt SE, O'Neal VM, Diamond JH, Borchelt DR. Prion-like propagation of mutant SOD1 misfolding and motor neuron disease spread along neuroanatomical pathways. Acta Neuropathol 2015; 131(1):103-14
- Holmes BB, DeVos SL, Kfoury N, Li M, Jacks R, Yanamandra K, Ouidja MO, Brodsky FM, Marasa J, Bagchi DP, et al. Heparan sulfate proteoglycans mediate internalization and propagation of specific proteopathic seeds. Proc Natl Acad Sci U S A 2013; 110(33): p:E3138-47; PMID:23898162; http://dx.doi.org/10.1073/pnas.1301440110
- Sundaramoorthy V, Walker AK, Yerbury J, Soo K, Farg MA, Hoang V, Zeineddine R, Spencer D, Atkin JD. Extracellular wildtype and mutant SOD1 induces ER-Golgi pathology characteristic of amyotrophic lateral sclerosis in neuronal cells. Cell Mol Life Sci, 2013; 70(21): p:4181-45; PMID:23765103; http://dx.doi.org/10.1007/s00018-013-1385-2
- Zeineddine R, Pundavela JF, Corcoran L, Stewart EM, Do-Ha D, Bax M, Guillemin G, Vine KL, Hatters DM, Ecroyd H, et al. SOD1 protein aggregates stimulate macropinocytosis in neurons to facilitate their propagation. Mol Neurodegener 2015; 10(1): p:57; PMID:26520394; http://dx.doi.org/10.1186/s13024-015-0053-4
- Lee H, Suk J, Bae E, Lee J, Paik S, Lee S, Assembly-dependent endocytosis and clearance of extracellular alphasynuclein. Int J Biochem Cell Biol 2008; 40(9): p:1835-49; PMID:18291704; http://dx.doi.org/10.1016/j.biocel.2008.01.017
- Trevino RS, Lauckner JE, Sourigues Y, Pearce MM, Bousset L, Melki R, Kopito RR. Fibrillar structure and charge determine the interaction of polyglutamine protein aggregates with the cell surface. J Biol Chem 2012; 287(35): p:29722-8; PMID:22753412; http://dx.doi.org/10.1074/jbc.M112.372474
- Frost B, Jacks RL, Diamond MI. Propagation of Tau misfolding from the outside to the inside of a cell. J Biol Chem, 2009; 284: p:12845-52; PMID:19282288; http://dx.doi.org/10.1074/jbc.M808759200
- Wu JW, Herman M, Liu L, Simoes S, Acker CM, Figueroa H, Steinberg JI, Margittai M, Kayed R, Zurzolo C, Paolo GD, et al. Small misfolded Tau species are internalized via bulk endocytosis and anterogradely and retrogradely transported in neurons. J Biol Chem 2013; 288(3): p:1856-70; PMID:23188818; http://dx.doi.org/10.1074/jbc.M112.394528
- Koivusalo M, Welch C, Hayashi H, Scott CC, Kim M, Alexander T, Touret N, Hahn KM, Grinstein S. Amiloride inhibits macropinocytosis by lowering submembranous pH and preventing Rac1 and Cdc42 signaling. J Cell Biol 2010; 188(4): p:547-63; PMID:20156964; http://dx.doi.org/10.1083/jcb.200908086
- Ivanov AI. Pharmacological inhibition of endocytic pathways: is it specific enough to be useful? Methods Mol Biol 2008; 440: p:15-33; PMID:18369934; http://dx.doi.org/10.1007/978-1-59745-178-9_2
- Mercer J, Helenius A. Gulping rather than sipping: macropinocytosis as a way of virus entry. Curr Opin Microbiol 2012; 15(4): p:490-9; PMID:22749376; http://dx.doi.org/10.1016/j.mib.2012.05.016
- Meier O, Boucke K, Hammer SV, Keller S, Stidwill RP, Hemmi S, Greber UF. Adenovirus triggers macropinocytosis and endosomal leakage together with its clathrin-mediated uptake. J Cell Biol 2002; 158(6): p:1119-31; PMID:12221069; http://dx.doi.org/10.1083/jcb.200112067
- Conner SD, Schmid SL. Regulated portals of entry into the cell. Nature 2003; 422(6927): p:37-44; PMID:12621426; http://dx.doi.org/10.1038/nature01451
- Kalia M, Khasa R, Sharma M, Nain M, Vrati S. Japanese encephalitis virus infects neuronal cells through a clathrin-independent endocytic mechanism. J Virol 2013; 87(1): p:148-62; PMID:23055570; http://dx.doi.org/10.1128/JVI.01399-12
- Izmailyan R, Hsao JC, Chung CS, Chen CH, Hsu PW, Liao CL, Chang W. Integrin beta1 mediates vaccinia virus entry through activation of PI3K/Akt signaling. J Virol 2012; 86(12): p:6677-87; PMID:22496232; http://dx.doi.org/10.1128/JVI.06860-11
- Schmid RS, Anton ES. Role of integrins in the development of the cerebral cortex. Cereb Cortex 2003; 13(3): p:219-24; PMID:12571112; http://dx.doi.org/10.1093/cercor/13.3.219
- Akula SM, Wang FZ, Vieira J, Chandran B. Human herpesvirus 8 interaction with target cells involves heparan sulfate. Virol 2001; 282(2): p:245-55; http://dx.doi.org/10.1006/viro.2000.0851
- Sanchez EG, Quintas A, Perez-Nunez D, Nogal M, Barroso S, Carrascosa AL, Revilla Y. African swine fever virus uses macropinocytosis to enter host cells. PLoS Pathog 2012; 8(6): p:e1002754; PMID:22719252; http://dx.doi.org/10.1371/journal.ppat.1002754
- Hahn AS, Kaufmann JK, Wies E, Naschberger E, Panteleev-Ivlev J, Schmidt K, Holzer A, Schmidt M, Chen J, Konig S, et al. The ephrin receptor tyrosine kinase A2 is a cellular receptor for Kaposi's sarcoma-associated herpesvirus. Nat Med 2012; 18(6): p:961-6; PMID:22635007; http://dx.doi.org/10.1038/nm.2805
- Horonchik L, Tzaban S, Ben-Zaken O, Yedidia Y, Rouvinski A, Papy-Garcia D, Barritault D, Vlodavsky I, Taraboulos A. Heparan sulfate is a cellular receptor for purified infectious prions. J Biol Chem 2005; 280(17): p:17062-7; PMID:15668247; http://dx.doi.org/10.1074/jbc.M500122200
- Schonberger O, Horonchik L, Gabizon R, Papy-Garcia D, Barritault D, Taraboulos A. Novel heparan mimetics potently inhibit the scrapie prion protein and its endocytosis. Biochem Biophys Res Commun 2003; 312(2): p:473-9; PMID:14637161; http://dx.doi.org/10.1016/j.bbrc.2003.10.150
- Fu H, Liu B, Frost JL, Hong S, Jin M, Ostaszewski B, Shankar GM, Costantino IM, Carroll MC, Mayadas TN, et al, Complement component C3 and complement receptor type 3 contribute to the phagocytosis and clearance of fibrillar Abeta by microglia. Glia 2012; 60(6): p:993-1003; PMID:22438044; http://dx.doi.org/10.1002/glia.22331
- Bamberger ME, Harris ME, McDonald DR, Husemann J, Landreth GE. A cell surface receptor complex for fibrillar β-amyloid mediates microglial activation. J Neurosci 2003; 23(7): p:2665-74; PMID:12684452
- Hook S, Roberts K, Kumita JR, Yerbury JJ. The interplay of protein aggregates, microglia and neuroinflammation in neurodegenerative disease, in Microglia: Physiology, Regulation and Health Implications, Giffard ER, Editor. 2015, Nova Publishers: New York. p:157-204
- Coraci IS, Husemann J, Berman JW, Hulette C, Dufour JH, Campanella GK, Luster AD, Silverstein SC, El-Khoury JB. CD36, a class B scavenger receptor, is expressed on microglia in Alzheimer's disease brains and can mediate production of reactive oxygen species in response to β-amyloid fibrils. Am J Pathol 2002; 160(1): p:101-12; PMID:11786404; http://dx.doi.org/10.1016/S0002-9440(10)64354-4
- El Khoury J, Hickman SE, Thomas CA, Cao L, Silverstein SC, Loike JD. Scavenger receptor-mediated adhesion of microglia to β-amyloid fibrils. Nature 1996; 382(6593): p:716-9; PMID:8751442; http://dx.doi.org/10.1038/382716a0
- Yan SD, Chen X, Fu J, Chen M, Zhu H, Roher A, Slattery T, Zhao L, Nagashima M, Morser J, et al. RAGE and amyloid-β peptide neurotoxicity in Alzheimer's disease. Nature 1996; 382(6593): p:685-91; PMID:8751438; http://dx.doi.org/10.1038/382685a0
- Deane R, Yan SD, Submamaryan RK, LaRue B, Jovanovic S, Hogg E, Welch D, Manness L, Lin C, Yu J, et al. RAGE mediates amyloid-β peptide transport across the blood-brain barrier and accumulation in brain. Nat Med 2003; 9(7): p:907-913; PMID:12808450; http://dx.doi.org/10.1038/nm890
- Le Y, Gong W, Tiffany HL, Tumanov A, Nedospasov S, Shen W, Dunlop NM, Gao JL, Murphy PM, Oppenheim JJ, et al. Amyloid (β)42 activates a G-protein-coupled chemoattractant receptor, FPR-like-1. J Neurosci 2001; 21(2): p:RC123; PMID:11160457
- Malik M, Simpson JF, Parikh I, Wilfred BR, Fardo DW, Nelson PT, Estus S. CD33 Alzheimer's risk-altering polymorphism, CD33 expression, and exon 2 splicing. J Neurosci 2013; 33(33): p:13320-5; PMID:23946390
- Reed-Geaghan, E.G., J.C. Savage, A.G. Hise, and G.E. Landreth, CD14 and toll-like receptors 2 and 4 are required for fibrillar A{β}-stimulated microglial activation. J Neurosci 2009; 29(38): p:11982-92; PMID:19776284
- Roberts K, Zeineddine R, Corcoran L, Li W, Campbell IL, Yerbury JJ. Extracellular aggregated Cu/Zn superoxide dismutase activates microglia to give a cytotoxic phenotype. Glia 2013; 61(3): p:409-419; PMID:23281114
- Zeng Y, Tao N, Chung KN, Heuser JE, Lublin DM. Endocytosis of oxidized low density lipoprotein through scavenger receptor CD36 utilizes a lipid raft pathway that does not require caveolin-1. J Biol Chem 2003; 278(46): p:45931-6; PMID:12947091
- Van Vactor D, Wall DP, Johnson KG. Heparan sulfate proteoglycans and the emergence of neuronal connectivity. Curr Opin Neurobiol, 2006; 16(1): p:40-51; PMID:16417999
- Hassid BG, Nair MN, Ducruet AF, Otten ML, Komotar RJ, Pinsky DJ, Schmidt AM, Yan SF, Connolly ES Neuronal RAGE expression modulates severity of injury following transient focal cerebral ischemia. J Clin Neurosci 2009; 16(2): p:302-6; PMID:19071026
- Glezer I, Bittencourt JC, Rivest S, Neuronal expression of Cd36, Cd44, and Cd83 antigen transcripts maps to distinct and specific murine brain circuits. J Comp Neurol 2009; 517(6): p:906-24; PMID:19844997
- Palmer A, Klein R. Multiple roles of ephrins in morphogenesis, neuronal networking, and brain function. Genes Dev 2003; 17(12): p:1429-50; PMID:12815065
- Jurney WM, Gallo G, Letourneau PC, McLoon SC. Rac1-mediated endocytosis during ephrin-A2- and semaphorin 3A-induced growth cone collapse. J Neurosci 2002; 22(14): p:6019-28; PMID:12122063
- Tom VJ, Steinmetz MP, Miller JH, Doller CM, Silver J. Studies on the development and behavior of the dystrophic growth cone, the hallmark of regeneration failure, in an in vitro model of the glial scar and after spinal cord injury. J Neurosci 2004 24(29): p:6531-9
- Kabayama H, Takeuchi M, Taniguchi M, Tokushige N, Kozaki S, Mizutani A, Nakamura T, Mikoshiba K. Syntaxin 1B suppresses macropinocytosis and semaphorin 3A-induced growth cone collapse. J Neurosci 2011; 31(20): p:7357-64; PMID:21593320
- Kolpak AL, Jiang J, Guo D, Standley C, Bellve K, Fogarty K, Bao ZZ. Negative guidance factor-induced macropinocytosis in the growth cone plays a critical role in repulsive axon turning. J Neurosci 2009; 29(34): p:10488-98; PMID:19710302
- Zeineddine R, Yerbury JJ. The role of macropinocytosis in the propagation of protein aggregation associated with neurodegenerative diseases. Front Physiol 2015; 6: p:277; PMID:26528186
- Van Hoecke A, Schoonaert L, Lemmens R, Timmers M, Staats KA, Laird AS, Peeters E, Philips T, Goris A, Dubois B, et al. EPHA4 is a disease modifier of amyotrophic lateral sclerosis in animal models and in humans. Nat Med 2012; 18(9): p:1418-22; PMID:22922411