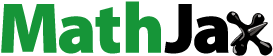
ABSTRACT
Bioassays of native cervid hosts have established the presence of infectious chronic wasting disease (CWD) prions in saliva, blood, urine, and feces of clinically diseased and pre-clinical infected deer. The intra-host trafficking of prions from the time of initial infection to shedding has been less well defined. We created a discrete-time compartmentalized model to simulate the misfolding catalysis, trafficking, and shedding of infectious prions throughout the organ systems of CWD-infected cervids. Using parameter values derived from experimental infections of North American deer (Odocoileus spp.), the exponential-based model predicts prion deposition over time with: 1) nervous tissues containing the highest deposition of prions at 20 months post-infection and 2) excreted fluids containing low levels of prions throughout infection with the highest numbers of prions predicted to be shed in saliva and feces (as high as 10 lethal doses (1.34 × 1029 prions) in 11–15 months). These findings are comparable to prion deposition described in literature as assayed by conventional and ultrasensitive amplification assays. The comparison of our model to published data suggests that highly sensitive assays (sPMCA, RT-QuIC, and bioassay) are appropriate for early prion detection in bodily fluids and secretions. The model provides a view of intra-host prion catalysis leading to pre-clinical shedding and provides a framework for continued development of antemortem diagnostic methods.
INTRODUCTION
Chronic wasting disease (CWD) is an invariably fatal, neurodegenerative disease affecting cervids (deer, elk, moose, and reindeer).Citation1-8 CWD has been confirmed in farmed and free-ranging cervid populations in 24 states, 2 Canadian provinces, South Korea, and Norway,Citation1,5-7,9-12 and is the only known transmissible spongiform encephalopathy (TSE) to affect both captive and wild cervid populations.Citation5,9-11,13
The disease is caused by the accumulation of abnormally folded prion protein (PrPRes) that has the capacity to convert additional normal cellular prion precursor protein (PrPC) to infectious prion protein (PrPD) by a templating mechanism not fully understood.Citation5-7,14-18 This conversion occurs at the protein level, without underlying genetic changes in the PrP precursor gene.Citation5 PrPC is expressed throughout the body, but is most abundant in nervous tissue.Citation16 The molecular biomarker for prion diseases (PrPRes) can be distinguished from PrPC by its partial resistances to proteolysis, tendency to form aggregated plaques, and insolubility in detergents.Citation6,14,16,19 Other prion diseases include kuru and Creutzfeldt-Jakob disease (CJD) in humans, bovine spongiform encephalopathy (BSE, mad cow disease) in cattle, and scrapie in sheep and goats.Citation6,13,16,18,20-21
In all prion diseases, deposits of PrPRes build up in the central nervous system and brain, developing a series of holes histologically resembling a sponge. This structure is termed spongiosis and results in the destruction of neural tissue and function.Citation7,16 CWD, like all TSEs, has a long preclinical stage of infection during which clinical signs of disease are not apparent (18 months to multiple decadesCitation6,17), which is followed by a shorter clinical phase of disease (2–6 months) when overt symptoms consistent with TSEs are present.Citation16,19 Clinical signs of CWD include weight and muscle loss, excessive salivation, and progressively worsening behavioral changes including altered stance, pacing, and hyper-excitability.Citation2,6 As no therapies or vaccines for CWD exist, death invariably follows.Citation5,16
Infectious prions have been detected by bioassay in urine,Citation11,22 saliva,Citation8,11-12 feces,Citation21,23 blood,Citation12 antler velvet,Citation24 and nasal secretionsCitation25 in preclinical and clinical deer and are shed into and contaminate the environment. Even a decaying carcass can act as a reservoir of infectious prions as PrPRes has been detected in skeletal and cardiac muscle, reproductive tissues, and fat.Citation2,6,23,26 CWD can be transmitted through direct contactCitation5 as well as through airborne routes (aerosolized fecal matter and soil-prion complexes).Citation3,5,6 In the environment, prions can remain infective for at least 2 years, potentially exposing and infecting a large number of deer indirectly.Citation5,6,9 As deer consume 8–30 grams (g) of soil per day, depending on the season,Citation5,23 environmental contamination may play an important role in CWD transmission. The relative importance of these various transmission routes is unclear, but evidence suggests that indirect environmental routes may be the major source for infection.Citation6 Studies and surveillance indicate that CWD has a low zoonotic potential, but since subclinical CWD-infected deer possess infectious prions, caution is still advisable when handling cervids.Citation6
The ecology of CWD at the population level, routes of infection and potential prion shedding mechanism are currently being studied.Citation3,9,11 What remains less well studied is the intra-host prion trafficking during the protracted incubation period.Citation11,16,22 The aim of our study was to establish a within-host model describing the patterns of prion trafficking and shedding within mule deer (Odocoileus hemionus). With the model, we simulated the timing of environmental shedding of infectious prions through saliva, urine, and feces relative to the onset of clinical symptoms. This understanding allows for the implementation of enhanced antemortem detection methods and management practices to better understand the epidemiology and spread of CWD.
RESULTS
Model prediction of intra-host prion spread
Using prion transition, replication, and initial invasion parameter values in Supplemental A, we simulated prion spread for 20 months post-infection to approximate a plausible incubation period for mule deer, which generally exhibit clinical signs 16.2 to 25.9 months after infection.Citation13 The nervous tissue, namely the brain, spinal cord, and olfactory bulb, contains the highest concentrations of prions at the end of 20 months. All other tissues exhibit prion concentrations at least one order of magnitude lower and are not visible on the same scale (See ).
Model predictions of prion shedding
The gold standards for detecting prions in tissues are western blot and immunohistochemistry (IHC).Citation14 We compared the predicted prion concentrations in tissues to the test sensitivity of western blot with a detection threshold of 4 ng/g (4 × 10−12 g/g).Citation19 As seen in , the model predicts that prions accumulate in the brain to this level by 12 months post-infection and should therefore be detectable by western blot. also contains model predictions of times to detection via western blot for other tissues commonly used for CWD-diagnostic tests. While prion infectivity has been found in excreta through bioassay, western blot has been unable to identify PrPRes in these samples.Citation10-12,23 The model predicts that excreted prions in saliva and feces may be detected by western blot by 20 months post-infection while urine is well below the detection threshold at this time (See ).
FIGURE 2. Predicted detection of prions in excreta (urine, saliva, feces, and a combined pool of all 3). The three panels show detection thresholds of (A) western blot (4 ng/g), (B) bioassay and RT-QuIC (1 fg/g), and (C) sPMCA (1.3 ag/g) against the predicted prion concentrations in excreta.
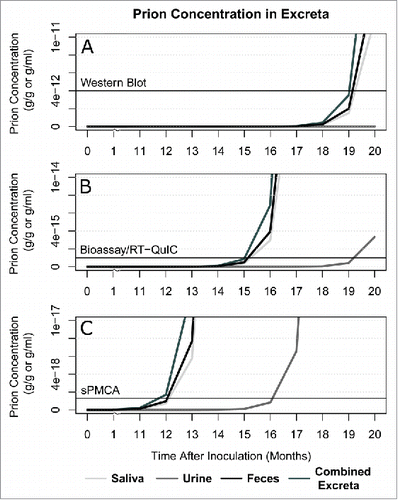
TABLE 1. Model predicts mean time (mpi)Footnote* to CWD detection by western blot in various tissues.
Recently, more sensitive methods for detecting prions in fluids have been developed.Citation4,14,18-20 These tests, however, may have higher rates of false positives. Serial protein misfolding cyclic amplification (sPMCA) relies on multiple rounds of incubation of PrPRes with excess PrPC and sonication to amplify the infectious prions present in a sample.Citation4,19 With 7 rounds of amplification, sPMCA has the capacity to detect as few as 1.3 ag/g (1.3 × 10−18 g/g).Citation19 Real-time quaking-induced conversion (RT-QuIC) relies on a similar method using recombinant PrPC substrate and shaking.Citation20,27 It has a detection threshold of about 1 fg/g (1 × 10−15 g/g),Citation20 roughly equivalent to the power of traditional bioassay.Citation18 illustrates predicted times at which both RT-QuIC (2B) and sPMCA (2C) should detect prions in urine, saliva, and feces.
To further explore the amount of prions shed into the environment during the incubation phase of CWD, we tracked the accumulation of prions in the environment from excreta, including saliva, urine, and feces. As seen in , the majority of prions shed into the environment occur through saliva followed closely by prions in feces. By 11 months post-infection, the number of prions shed into the environment through excreta constitutes one lethal intracerebral experimental dose (1 fgCitation18 or 1.34 × 10Citation28 prions assuming the molecular weight of PrPRes is 27 kDaCitation25). Within another month, the total number of prions shed into the environment by excreta since inoculation reaches 10 lethal doses (1.34 × 10Citation29 prions).
FIGURE 3. Predicted number of total accumulated prions in excreta (saliva, urine, feces, and a combined pool of all 3). Horizontal lines indicate the equivalent number of prions in one and 10 experimental intracerebral lethal doses (1.34 × 10Citation28,29 prions).
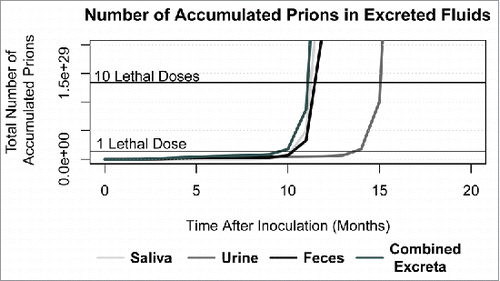
Sensitivity of model parameters
We tested sensitivity of response variables to parameter values used in the model. For the main response of interest—time (months) after infection until 10 lethal doses (1.34 × 10Citation29 prions) accumulated in the environment via excreted fluids—10 of the 78 model parameters changed the outcome of the model (see ). Sensitive transition parameters included the transition of prions to and from the brain and from the olfactory bulb to swallowed and orally excreted saliva. Sensitive replication parameters included replication of prions in the brain and rectal follicles. During initial uptake of prions, invasion of the lymph and blood were the most sensitive.
FIGURE 4. First order sensitivity of the 10 most sensitive parameters in the model. Parameters that have a range that do not contain zero and whose maximum sensitivity are greater than 1 × 10−3 are considered the most sensitive parameters. Parameters are grouped by the ‘class’ of model parameters to which they belong: transition, replication or initial invasion. Transition parameters are labeled , indicating movement of prions from tissue
to tissue
. Outliers, defined as data points outside of 1.5 interquartile ranges of the first and third quartiles, are indicated by points outside of the whiskers. Sal, saliva; ExSal, excreted saliva; OfB, olfactory bulb; Brn, brain; SpC, spinal cord; ANS, autonomic nervous system; Bld, blood; RF, rectal follicles; Lph, lymph.
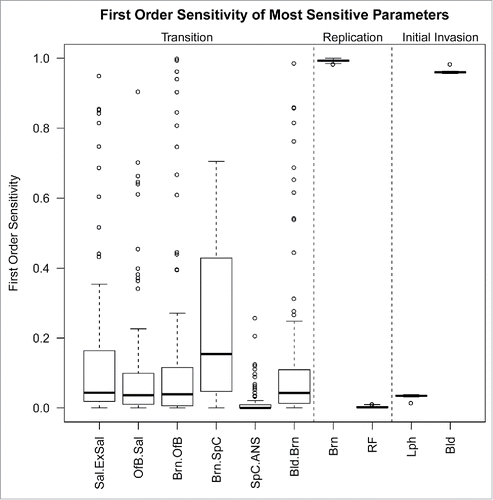
Using the range of parameter values shown in Supplemental A, the range of post-infection times required to reach environmental accumulation of 10 lethal doses (1.34 × 10Citation29 prions) ranged from 10–18 months (data not shown).
DISCUSSION
Our aim was to develop a model describing intra-host prion trafficking and shedding of prions, an area lacking in CWD literature. Using literature-derived values and assumptions pertaining to the temporal and spatial distribution of PrPC and PrPRes, the organ systems implicated in disease progression and detection of prions in excreta, the model describes the complete system and pattern of intra-host trafficking from infection to shedding. The model predicts that the brain, olfactory bulb, and spinal cord contain the highest deposition of prions by the end of a 20 month incubation period and that throughout this period, prions are shed through saliva, urine, and feces. During the majority of the incubation period, the model predicts that prions cannot be detected via western blot in the excreta. Predicted prion concentrations in saliva, however, are detectable by sPMCA and RT-QuIC as early as 11 and 15 months post-infection, respectively.
The sensitivity analysis helps to validate our model. The sensitivity of the model to replication in the brain suggests that this is a location where a large amount of PrPC to PrPD conversion occurs in vivo. This corresponds to known high concentrations of conversion-ready PrPC in the central nervous system (CNS).Citation16 The high model sensitivity to replication in the brain indicates that levels of shed prions depends strongly upon prion replication in the brain, even though these CNS prions must then pass through other organ systems to be shed. Physiologically, replication in the brain results in the development of plaques and spongiosis of neural tissue, resulting in clinical disease. This model suggests that large accumulation of shed prions occurs late in infection, likely localizing the environmental reservoir and facilitating CWD horizontal transmission. This corresponds to observed spatial clustering of CWD cases.Citation6,28 Transition parameters with high sensitivity included the movement of prions from the brain to excreted saliva, and the movement of prions through the CNS. This implicates salivary shedding as the most important source of environmental prions. It also predicts that the majority of infectious prions originate in the CNS. It has been shown that feces and saliva contain similar infectivity, and that infectivity is greater than that of urine.Citation8,14 Similarly, the rate of initial prion invasion into the blood and lymph also influences the environmental pool of prions, and illustrates that the earlier prions enter the circulatory system, the earlier shedding will occur; potentially because the blood widely disseminates prions throughout bodily systems. Prions can be detected in the blood within minutes of inoculation and remain throughout the infection,Citation27 illustrating the importance of blood in prion dissemination.
Corroborating earlier studies,Citation7,11 this model assumes exponential deposition of prions in tissues over time and predicts that the brain, olfactory bulb, and spinal cord contain the highest accumulation at the end of 20 months. As previously illustrated in experimental CWD studies, western blot detects prions in the obex of the brainstem by 16–22 months post-infectionCitation29 although another study did not detect prions 19 months post-infection.Citation10 As seen in , our model predicts the brain will test CWD-positive by western blot by 12 months post-infection. This suggests that the predicted rate of prion accumulation is faster than experimentally reported. Further, the model predicts that western blot will test CWD-positive in tonsils by 19 months post-infection and in rectal mucosa lymphoid tissue by 18 months post-infection (see ). Western blot CWD-positivity has been reported in tonsils 6 months post-infection and 6–12 months post-infection in rectal mucosa lymphoid tissue.Citation3 It appears that the model either over-estimates prion spread into and replication within the neural tissues, or under-estimates prion dissemination from these tissues. Alternatively, but not exclusively, the model may under-estimate prion transition to and replication within lymphatic compartments or over-estimate the loss of prions from these compartments. This highlights the need for further experimental observation of these rates.
The predicted prion concentration within the nervous system is biologically impossible. As seen in , the final PrPRes concentration in the brain is about 0.1 mg/g while the spinal cord and olfactory bulb have PrPRes concentrations between 10 µg/g and 20 µg/g. This is not biologically possible and thus, the model prediction may result from a lack of evidence describing these in vivo transition parameters. Moreover, an as-of-yet unknown and unincorporated variable or mechanism may prevent the model from producing completely realistic predictions. Additionally, replication parameters remain the same over the course of the simulation. It has been shown that as the disease progresses, the pool of available cellular prions decrease as PrPD converts PrPC,Citation17 thus reducing prion replication rate within organs. In nature, we would hypothesize that both the transition and replication parameters for tissues would vary over the course of infection.
While the manner in which prions transition between compartments is unknown, we assumed the most general case, namely that prions traffic between tissue compartments. However, since the in vivo parameter values are unknown, it was difficult to determine if this scenario of prion movement and misfolding catalysis reflects the actual prion transitions in vivo. Further research is needed to clarify this step.
As illustrated in , western blot analysis does not have the required sensitivity to detect prions in fluids until late in CWD incubation and is thus not an effective diagnostic tool. RT-QuIC, bioassay, and sPMCA have detection thresholds 3 to 6 orders of magnitude lower than western blotCitation19 (see and C). Thus, these techniques are better tools for early detection of TSEs.Citation13,18-20,29 Bioassay has detected prions in saliva at 6–18 months post-infection,Citation8,12 and in feces at 9 months post-infection.Citation23 Prions have been detected in urine by RT-QuIC as early as 13–16 months post-infection.Citation13 Our model predicts that saliva and feces should test positive by bioassay and RT-QuIC at 15 months while urine is positive at 19 months (see ). Thus, our model predicts lower prion concentrations in urine and feces than experimentally observed while predicting saliva concentrations at the lower end of observed concentrations.
Given that the model predicts lower prion concentrations in excreted fluids than observed, represents a conservative view of pre-symptomatic shedding. We predict that 10–14 months is required for one lethal dose of prions (1.34 × 10Citation28 prions) to be shed via saliva, feces, or urine. On the other hand, a 10-fold increase in number of prions in excreta occurs within one to 2 months after the accumulation of one lethal dose. It is easy to see that the number of excreted prions could equal those found in the terminal brain very quickly.Citation8 The model predicts a large amount of shedding in the later part of infection with the bulk of shed prions deriving from saliva and feces. This highlights a potential route of direct transmission through social encounters and grooming where infectious saliva, urine or feces may transfer between individuals. Because females tend to congregate in herds more frequently than males,Citation30 direct contact with saliva and feces shed by CWD-positive individuals may have a larger impact on transmission dynamics in females than in males. However, males may inhale more environmental CWD particles because they engage in more behaviors that generate dust.Citation5 Additionally, the flehmen response in males may efficiently expose them to large quantities of infectious urine, saliva and feces during the rut.Citation14 Indirect environmental contamination should be a route of transmission for both sexes.
Intra-host prion trafficking rates and the manner in which these transitions occur needs further exploration. However, this model's novel description of intra-host trafficking reinforces the importance of pre-clinical shedding and implicates saliva and feces as the primary sources of prions for transmission, which occurs mainly in later stages of CWD incubation. This means that direct contact with excreta through grooming and social interactions may be important mechanism of transmission. Even with the model's conservative prediction of shedding through saliva, urine, and feces, the number of prions excreted pre-clinically by a single infected animal can reach 10 lethal doses, quickly producing a large environmental prion pool. The model also indicates that while western blot is useful in validating the presence of prions once clinical disease is obvious, a more sensitive test is needed to identify early prion infection. RT-QuIC, sPMCA, and bioassay are more appropriate in detecting low levels of prions in excreta in the early stages of CWD. Overall, the model provides insight into intra-host CWD disease dynamics and may provide assistance in the development of antemortem detection methodologies and strategies for the management of CWD in cervid populations.
MATERIALS AND METHODS
Biological structure of the model
The model parameters mirror an infected female mule deer in terms of organ size and transition parameters gleaned from published experimental infections. Where information on mule deer was not available, data from white-tailed deer were substituted. Mule deer and white-tailed deer are phylogenetic sister species,Citation31-32 are very similar physically,Citation31 and thus acquire CWD similarly. The model was coded and analyzed using version 3.2.2 of the R programming package (R Core Team (2015). R: A language and environment for statistical computing. R Foundation for Statistical Computing, Vienna, Austria. URL https://www.R-project.org/.).
The current understanding of intra-host CWD trafficking suggests that PrPD spreads from the peripheral infection site (generally lymphoid tissues) to the central nervous system via neural and lymphatic pathways where amplification occurs.Citation15,33,34 Infectious prions then spread back to peripheral tissues.Citation10-11,25 After initial infection, prion deposits grow throughout the cervid bodily systems as PrPC is misfolded into PrPD. We limit possible routes of prion transmission to physically interacting or adjacent organs as outlined in . Our work aims to clarify the relative importance of these many potential pathways.
FIGURE 5. Current hypothesized spread of PrPD in mule deer. Prions can transition between organs and fluids as CWD progresses. A single-headed arrow indicates that prions only transition in that direction while a double-headed arrow indicates that prion transition occurs in both directions between those tissues.
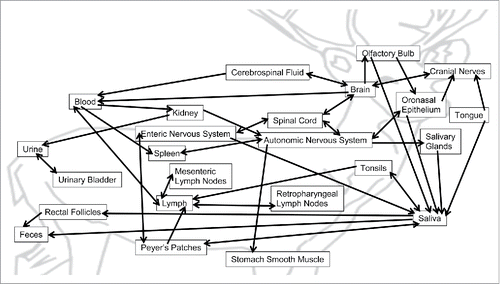
Our model assumes oral inoculation, as is most common for in vivo laboratory CWD investigations. Ingested material passes through the oronasal cavities and then progresses through the gastrointestinal (GI) tract. In order to gain entry to the body, prions must cross the mucosal epithelium into bodily fluids or become deposited in the oronasal epithelium. Prions cross the epithelium and are moved to lymph by paracellular transport.Citation2,15 Prions also pass into blood within minutes of oral exposureCitation27 and can be taken up by lymphoid tissue (tonsils and Peyer's patches) that continually sample the GI environment for foreign material.Citation5,16,21,33 Both routes deposit PrPD into the lymph, passing through regional lymph nodes before draining back into the circulatory system. In circulation, prion-infected blood passes through the spleen, depositing prions.Citation2 Prions enter the nervous system from the sympathetically innervated spleenCitation7 or Peyer's patch-associated enteric nerves.Citation16 Autonomic and enteric nerves are thought to allow PrPD to spread to and amplify within the spinal cord before invading the brain.Citation2,7 Neuroinvasion can also occur directly through cranial nerves in the tongue and oronasal epithelium, but this process appears to be slower than the lymphatic route.Citation8,33 After amplification in the brain, prions spread back through the autonomic and enteric nervous systems to infect peripheral tissues like skeletal muscle, kidneys, and salivary glands.Citation2 Within the olfactory bulb of the brain, prions pass through olfactory receptor neurons, which amplify PrPD to the oronasal epithelium.Citation5,25 The brain can also receive prions from the blood at the circumventricular organs which lack the blood-brain barrier.Citation4 As the disease progresses, tissues throughout the brain deteriorate and prions can leak through the ependyma into the cerebrospinal fluid. Shedding prions into saliva occurs from tissues associated with the GI tract, like the tongue, salivary glands, lymphoid tissues, and enteric and olfactory neurons.Citation21,25,34 Deer swallow the majority of their saliva, leading to potential increased prion deposition into tissues of the GI tract.Citation2,8 The presence of prions in urine may arise from the filtrate passing through the kidneys as well as infectious prions shed from the kidneys and urinary bladder. Urine titers of PrPRes are very similar to those of the blood, indicating that the majority of PrPRes present derives from the blood.Citation22
In order for prion deposition to occur across bodily organs and fluids, prions must transition between organs and fluids that interact with each other; prions cannot directly “jump” to non-adjacent tissues. In the instances when prion accumulation occurs in physically disparate tissues, bodily fluids like blood or lymph must be responsible for the prion transport. Prion transition between organs may occur by 2 methods: by directly moving into the adjacent organ or by catalyzing the conversion of PrPC to PrPD at the cellular border between organs without the prion catalyst actually exiting the original host tissue. It is unclear which method occurs in natural CWD infection, which is reflected in the use of ambiguous terms such as “prion spread”Citation7 and “physical interactions.”Citation16 Other experiments support the idea that PrPD must come in direct contact with PrPC in order to convert it,Citation15 thus implying that prions must physically move between tissues. To provide the most general model of prion transitions, our model assumes that prions always physically move between tissues, or undergo “border crossing,” for all transitions. Other possible transition schemes might include that prions only “border cross” in and out of fluids (shedding into and deposition by fluids) or only “border cross” into fluids (shedding into fluids) while at all other interfaces, prions simply catalyze conversion of PrPC to PrPD in adjacent tissues without leaving the host tissue. All three possible transition schemes are included in the R code in Supplemental C, but in this report we present and discuss only the most general transition scheme where prions always “border cross” between all tissues.
The amount of prion deposition in each organ depends on the pre-existing amount of prion precursor available in the organ to be converted. The brain and nervous tissue contain the highest concentrations of cellular prion precursorCitation16 so these would have the largest deposition of prions. All other tissues have at least 10x lower concentration of cellular prion precursor than the brain while fluids contain none.
Mathematical structure of model
We modeled the spread of prions during infection as a discrete time, compartmentalized model. The growth within and transition between compartments at each monthly time step (t) is given by:(1)
(1)
At each time step, vector pt, where pt is of length 27, represents the number of prions in each of 27 tissue compartments. We assume that prion transitions between compartments occur before prion replication. Assuming the oral inoculation route, initial prion distribution at is determined by the portion of infectious inoculum taken up by tissues along the GI tract (see Supplemental A for values). To correspond with experimental infections, all modeled infections begin with one lethal intracerebral dose of PrPD (1 fgCitation18 or 1.34 × 10Citation28 PrPD proteins, assuming the molecular weight of PrPRes is 27 kDaCitation25).Citation22 We assumed that initial tissue uptake of prions is temporally instantaneous relative to the model's monthly time scale; no unassimilated inoculum remains after the first time step.
Transition parameters, which illustrate the “border crossing” of prions between tissue compartments, are stored in matrix A (see Supplemental B). For example, see below a row of the transition matrix A () which corresponds to prion transitions out of the autonomic nervous system (ANS). Tissues into which prions transition from the ANS are listed above the associated transition parameters.
Transition parameters for organ-to-organ and fluid-to-organ interactions take into account the proximity of tissues, relative size of organs, and level of PrPC. Tissues that are not physically in contact, like the ANS and cerebrospinal fluid (CSF), have no direct “border crossing” between them so the corresponding transition parameter between the ANS and CSF () equals zero. Also, the relative size and amount of surface area in contact between adjacent tissue compartments inform the degree to which prion “border crossings” are possible. As publications do not report surface areas or overlaps between compartments, estimates were based on relative organ masses as well as physiological co-location and function of organs that would facilitate overlapping. For example, the oronasal epithelium, a large tissue compartment, would share a larger total amount of surface area with the ANS than the salivary glands, a small compartment, because of the larger number of innervations the ANS has with the oronasal epithelium (See for organ masses). Thus, the transition parameter for prions “border crossing” from the ANS into the oronasal epithelium (
) is larger than that of the ANS into the salivary glands (
).
Transition parameters for organ-to-fluid interactions encompass cellular turnover rates, as well as prion concentrations in the organs. During normal cellular turnover, apoptosis is thought to release PrPD and PrPC into the surrounding bodily fluids of blood, lymph, saliva, urine, and feces.Citation9 Since nervous tissue contains at least 10x more prion precursor than other tissues, transition parameters for nervous tissue to fluid “border crossing” is larger than that of prions entering fluids from non-nervous tissue. For example, the mean value for transition from the olfactory bulb neurons to saliva is 0.5 while the mean value for Peyer's patches to saliva is 0.05 (see Supplemental B).
TABLE 2. Organ mass and volume of fluids present monthly in mule deer.
Growth terms along the diagonal of A incorporate both the growth within and the movement between compartments of prions. The amount of PrPC present dictates the degree to which prions can catalyze prion precursor misfolding and thus replicate in that tissue. A high level of prion precursor provides a large substrate pool for prion catalysis, resulting in large increases in prion number and a comparatively larger replication term than a compartment with a lower level of prion precursor. For example, the urinary bladder, which has 10-times less prion precursor than the brain, has a replication term of 2.0 while the kidneys, which have 20 to 100-times less prion precursor than the brain, have a replication term of 1.1. See Supplemental A for the complete list of parameter values. As fluids do not contain endogenous PrPC other than that shed from surrounding tissues, the replication term for fluids is 1; prions can only enter these tissues by “border crossing,” not by within-tissue conversion of PrPC. Because our model assumes that prions physically leave tissues during the transition phase, there are fewer prions left in the compartment during the replication phase and the growth term in the diagonal of A is only a portion of the replication term for that compartment. For example, if compartment has replication term,
but 20% of the prions leave compartment x each month, the diagonal of A for compartment
is:
.
While the model uses prion counts for the calculation of spread of prions throughout tissues, we also calculate concentrations within tissue compartments to allow for comparison to published values, specifically when determining predicted time to detection by methods of known detection thresholds. For this conversion, prion molecular weight was assumed to be 27 kDa.Citation25 contains a list of the mass or volume of each organ and fluid. For fluids that are continually excreted, the total volume produced per month is used.
Parameter values used for calculating the spread of prions throughout the mule deer are listed in (mean parameter values).
Sensitivity analysis
We evaluated first order sensitivity of parameters using ‘Distributed Evaluation of Local Sensitivity Analysis’ (function ‘delsa’) in the sensitivity package (https://cran.r-project.org/web/packages/sensitivity/sensitivity.pdf) of the program R. The function scaled sensitivity for each parameter by the variance in the response variable attributed to that parameter divided by the total variance in the response variable for all inputted parameters. We declared the response variable to be the number of months post-infection required to accumulate 10 lethal doses (1.34 × 10Citation29 prions) of shed prions in the environment. Parameters belonged in 3 ‘classes’—transition, replication, and initial invasion—in order to simplify analysis and provide validation for each part of the model. We analyzed sensitivity on each parameter class individually. See parameter means and ranges used for sensitivity analysis in Supplemental A.
ABBREVIATIONS
ANS | = | autonomic nervous system |
Bld | = | blood |
Brn | = | brain |
CN | = | cranial nerves |
CNS | = | central nervous system |
CSF | = | cerebrospinal fluid |
CWD | = | chronic wasting disease |
ENS | = | enteric nervous system |
Epi | = | oronasal epithelium |
ExFcs | = | excreted feces |
ExSal | = | excreted saliva |
ExUrn | = | excreted urine |
Fcs | = | feces |
GI | = | gastrointestinal |
IHC | = | immunohistochemistry |
Kd | = | kidney |
Lph | = | lymph |
MLN | = | mesenteric lymph nodes |
OfB | = | olfactory bulb |
PP | = | Peyer's patches |
PrPC | = | cellular prion protein |
PrPD | = | disease forming aberrant prion |
PrPRes | = | protein kinase resistant prion protein of unknown infectivity detected by assays |
RF | = | rectal follicles |
RPLN | = | retropharyngeal lymph nodes |
RT-QuIC | = | real-time quaking-induced conversion |
Sal | = | saliva |
SGd | = | salivary glands |
SpC | = | spinal cord |
Spl | = | spleen |
sPMCA | = | serial protein misfolding cyclic amplification |
SSM | = | stomach smooth muscle |
Tng | = | tongue |
TSE | = | transmissible spongiform encephalopathy |
Tsl | = | tonsils |
UB | = | urinary bladder |
Urn | = | urine |
DISCLOSURE OF POTENTIAL OF CONFLICTS OF INTEREST
No potential of conflicts of interest were disclosed.
Supplemental_Material.zip
Download Zip (107.3 KB)ACKNOWLEDGMENTS
We thank the many prion researchers at Colorado State University who have contributed their generous time and efforts in the development of many of the ideas presented here.
Funding
NLG was supported by the National Science Foundation (EF-00914489).
REFERENCES
- Becker R. Deadly animal prion disease appears in Europe. Nature 2016; http://dx.doi.org/10.1038/nature.2016.19759
- Fox K, Jewell J, Williams E, Miller M. Patterns of PrPCWD accumulation during the course of chronic wasting disease infection in orally inoculated mule deer (Odocoileus hemionus). J Gen Virol 2006; 87:3451-61; PMID:17030882; http://dx.doi.org/10.1099/vir.0.81999-0
- Denkers N, Hayes-Klug J, Anderson K, Seeling D, Haley N, Dahmes S, Osborn D, Miller K, Warren R, Mathiason C, et al. Aerosol transmission of chronic wasting disease in white-tailed deer. J Virol 2013; 87:1890-2; PMID:23175370; http://dx.doi.org/10.1128/JVI.02852-12
- Nichols T, Spraker T, Gidlewski T, Powers J, Telling G, VerCauteren K, Zabel M. Detection of prion protein in the cerebrospinal fluid of elk (Cervus Canadensis nelson) with chronic wasting disease using prion misfolding cyclic amplification. J Vet Diagn Invest 2012; 24:746-9; PMID:22621952; http://dx.doi.org/10.1177/1040638712448060
- Nichols T, Spraker T, Rigg T, Meyerett-Reid C, Hoover C, Michel B, Bian J, Hoover E, Gidlewski T, Balachandran A, et al. Intranasal inoculation of white-tailed deer (Odocoileus viginianus) with lyophilized chronic wasting disease prion particulate complexed with montmorillonite clay. PLoS One 2013; 8:e62455; PMID:23671598; http://dx.doi.org/10.1371/journal.pone.0062455
- Saunders S, Bartelt-Hunt S, Bartz J. Occurrence, transmission, and zoonotic potential of chronic wasting disease. Emerg Infect Dis 2012; 18:369-76; PMID:22377159; http://dx.doi.org/10.3201/eid1803.110685
- Seeling D, Mason G, Telling G, Hoover E. Chronic wasting disease prion trafficking via the autonomic nervous system. Am J Pathol 2011; 179:1319-28; PMID:21777560; http://dx.doi.org/10.1016/j.ajpath.2011.05.057
- Tamgüney G, Richt J, Hamir A, Greenlee J, Miller M, Wolfe L, Sirochman T, Young A, Glidden D, Johnson N, et al. Salivary prions in sheep and deer. Prion; 6:52-61; PMID:22453179; http://dx.doi.org/10.4161/pri.6.1.16984
- Haley N, Mathiason C, Carver S, Zabel M, Telling G, Hoover E. Detection of chronic wasting disease prions in salivary, urinary, and intestinal tissues of deer: potential mechanisms of prion shedding and transmission. J Virol 2011; 85:6309-18; PMID:21525361; http://dx.doi.org/10.1128/JVI.00425-11
- Haley N, Mathiason C, Zabel M, Telling G, Hoover E. Detection of sub-clinical CWD infection in conventional test-negative deer long after oral exposure to urine and feces from CWD+ deer. PLoS One 2009; 4:e7990; PMID:19956732; http://dx.doi.org/10.1371/journal.pone.0007990
- Haley N, Seeling D, Zabel M, Telling G, Hoover E. Detection of CWD prions in urine and saliva of deer by transgenic mouse bioassay. PLoS One 2009; 4:e4848; PMID:19293928; http://dx.doi.org/10.1371/journal.pone.0004848
- Mathiason C, Hays S, Powers J, Hayes-Klug J, Langenberg J, Dahmes S, Osborn D, Miller K, Warren R, Mason G, et al. Infectious prions in pre-clinical deer and transmission of chronic wasting disease solely by environmental exposure. PLoS One 2009; 4:e5916; PMID:19529769; http://dx.doi.org/10.1371/journal.pone.0005916
- John R, Schätzl H, Glich S. Early detection of chronic wasting disease prions in urine of pre-symptomatic deer by real-time quaking-induced conversion assay. Prion 2013; 7:253-8; PMID:23764839; http://dx.doi.org/10.4161/pri.24430
- Henderson D, Davenport K, Haley N, Denkers N, Mathiason C, Hoover E. Quantitative assessment of prion infectivity in tissues and bodily fluids by real-time quaking-induced conversion. J Gen Virol 2015; 96:210-9; PMID:25304654; http://dx.doi.org/10.1099/vir.0.069906-0
- Kincaid E, Hudson K, Richey M, Bartz J. Rapid transepithelial transport of prions following inhalation. J Virol 2012; 86:12731-40; PMID:22973025; http://dx.doi.org/10.1128/JVI.01930-12
- Kujala P, Raymond C, Romeijn M, Godsave S, van Kasteren S, Wille H, Prusiner S, Mabbott N, Peters P. Prion uptake in the gut: identification of the first uptake and replication sites. PLoS Pathog 2011; 7:e1002449; PMID:22216002; http://dx.doi.org/10.1371/journal.ppat.1002449
- Mays C, Kim C, Haldiman T, van der Merwe J, Lau A, Yang J, Grams J, Di Bari M, Nonno R, Telling G, et al. Prion disease tempo determined by host-dependent substrate reduction. J Clin Invest 2014; 124:847-58; PMID:24430187; http://dx.doi.org/10.1172/JCI72241
- Wilham J, Orrú C, Bessen R, Atarashi R, Sano K, Race B, Meade-White K, Taubner L, Timmes A, Caugheu B. Rapid end-point quantitation of prion seeding activity with sensitivity comparable to bioassays. PLoS Pathog 2010; 6:e1001217; PMID:21152012; http://dx.doi.org/10.1371/journal.ppat.1001217
- Saa P, Castillas J, Soto C. Ultra-efficient replication of infectious prions by automated protein misfolding cyclic amplification. J Biol Chem 2006; 281:35245-52; PMID:16982620; http://dx.doi.org/10.1074/jbc.M603964200
- Atarashi R, Sano K, Satoh K, Nishida N. Real-time quaking-induced conversion a highly sensitive assay for prion detection. Prion 2011; 5:150-3; PMID:21778820; http://dx.doi.org/10.4161/pri.5.3.16893
- Safar J, Lessard P, Tamgüney G, Freyman Y, Deering C, Letessier F, DeArmond S, Prusiner S. Transmission and detection of prions in feces. J Infect Dis 2008; 198:81-9; PMID:18505383; http://dx.doi.org/10.1086/588193
- Gregori L, Kovacs G, Alexeeva I, Budka H, Rowher R. Excretion of transmissible spongiform encephalopathy infectivity in urine. Emerg Infect Dis 2008; 14:1406-12; PMID:18760007; http://dx.doi.org/10.3201/eid1409.080259
- Tamgüney G, Miller M, Wolfe L, Sirochman T, Glidden D, Palmer C, Lemus A, DeArmond S, Prusiner S. Asymptomatic deer excrete infectious prions in faeces. Nature 2009; 461:529-32; PMID:19741608; http://dx.doi.org/10.1038/nature08289
- Angers R, Seward T, Napier D, Green M, Hoover E, Spraker T, O'Rourke K, Balachandran A, Telling G. Chronic wasting disease prions in elk antler velvet. Emerg Infect Dis 2009; 15:696-703; PMID:19402954; http://dx.doi.org/10.3201/eid1505.081458
- Bessen R, Shearin H, Martinka S, Boharski R, Lowe D, Wilham J, Caughey B, Wiley J. Prion shedding from the olfactory neurons into nasal secretions. PLoS Pathog 2010; 6:e1000837; PMID:20419120; http://dx.doi.org/10.1371/journal.ppat.1000837
- Angers R, Browning S, Seward T, Sigurdson C, Miller M, Hoover E, Telling G. Prions in skeletal muscles of deer with chronic wasting disease. Science 2006; 311:1117; PMID:16439622; http://dx.doi.org/10.1126/science.1122864
- Elder A, Henderson D, Nalls A, Hoover E, Kincaid A, Bartz J, Mathiason C. Immediate and ongoing detection of prions in the blood of hamsters and deer following oral, nasal, and blood inoculations. J Virol 2015; 89:7421-4; PMID:25926635; http://dx.doi.org/10.1128/JVI.00760-15
- Robinson S, Samuel M, Rolley R, Shelton P. Using landscape epidemiology models to understand the distribution of chronic wasting disease in the Midwestern USA. Landscape Ecol 2013; 28:1923-35; http://dx.doi.org/10.1007/s10980-013-9919-4
- Elder A, Henderson D, Nalls A, Wilham J, Caughey B, Hoover E, Kincaid A, Bartz J, Mathiason C. In vitro detection of prionemia in TSE-infected cervids and hamsters. PLoS One 2013; 8:e80203; PMID:24224043; http://dx.doi.org/10.1371/journal.pone.0080203
- Bender L, Myers W, Gould W. Comparison of helicopter and ground surveys for North American elk Cervus elaphus and mule deer Odocoileus hemionus population composition. Wildlife Biol 2003; 9:199-205.
- Gilbert C, Ropiquet A, Hassanin A. Mitochondrial and nuclear phylogenies of Cervidae (Mammalia, Ruminantia): systematics, morphology, and biogeography. Mol Phylogenet Evol 2006; 40:101-17; PMID:16584894; http://dx.doi.org/10.1016/j.ympev.2006.02.017
- Polziehn R, Strobeck C. Phylogeny of wapiti, red deer, sika deer, and other North American cervids as determined from mitochondrial DNA. Mol Phylogenet Evol 1998; 10:249-58; PMID:9878235; http://dx.doi.org/10.1006/mpev.1998.0527
- Bessen R, Martinka S, Kelly J, Gonzalez D. Role of lymphoreticular system in prion neuroinvasion from the oral and nasal mucosa. J Virol 2009; 83:6435-45; PMID:19369351; http://dx.doi.org/10.1128/JVI.00018-09
- DeJoia C, Moreaux B, O'Connell K, Bessen R. Prion infection of oral and nasal mucosa. J Virol 2006; 80:4546-56; PMID:16611915; http://dx.doi.org/10.1128/JVI.80.9.4546-4556.2006
- Ranslow A, Richter J, Neuberger T, Van Valkenburgh B, Rumple C, Quigley A, Pang B, Krane M, Craven B. Reconstruction and morphometric analysis of the nasal airway of white-tailed deer (Odocoileus viginianus) and implications regarding respiratory and olfactory airflow. Anat Rec 2014; 297:2138-47; PMID:25312370; http://dx.doi.org/10.1002/ar.23037
- Meadows S, Hakonson T. Contribution of tissues to body mass in elk. J Wildlife Manage 1982;46:838-41; http://dx.doi.org/10.2307/3808588
- Chitwood M, DePerno C, Flowers J, Kennedy-Stoskopf S. Physiological condition of female white-tailed deer in nutrient–deficient habitat type. Southeast Nat 2013; 12:307-16; http://dx.doi.org/10.1656/058.012.0206
- Groves and Rebec, Introduction to Biological Psychology, 3rd edition, Dubuque: Wm.C. Brown Publ., 1988.