ABSTRACT
Sequestration of aggregates into specialized deposition sites occurs in many species across all kingdoms of life ranging from bacteria to mammals and is commonly believed to have a cytoprotective function. Yeast cells possess at least 3 different spatially separated deposition sites, one of which is termed “Insoluble Protein Deposit (IPOD)” and harbors amyloid aggregates. We have recently discovered that recruitment of amyloid aggregates to the IPOD uses an actin cable based recruitment machinery that also involves vesicular transport.Citation1 Here we discuss how different proteins known to be involved in vesicular transport processes to the vacuole might act to guide amyloid aggregates to the IPOD. These factors include the Myosin V motor protein Myo2 involved in transporting vacuolar vesicles along actin cables, the transmembrane protein Atg9 involved in the recruitment of large precursor hydrolase complexes to the vacuole, the phosphatidylinositol/ phosphatidylcholine (PI/PC) transfer protein Sec 14 and the SNARE chaperone Sec 18. Furthermore, we present new data suggesting that the yeast dynamin homolog Vps1 is also crucial for faithful delivery of the amyloid model protein PrD-GFP to the IPOD. This is in agreement with a previously identified role for Vps1 in recruitment of heat-denatured aggregates to a perivacuolar deposition site.Citation2
YEAST PROTEIN QUALITY CONTROL COMPARTMENTS (PQC's)
Yeast cells possess at least 3 different spatially separated deposition sites for misfolded and aggregated proteins termed protein quality control compartments (PQC's). Those comprise the peri- or intranuclear quality control compartment JUNQ or INQ,Citation3-5 respectively, the cytosolic quality control-bodies (Q-bodies),Citation6 also known as peripheral aggregatesCitation7 or stress foci,Citation8 and the perivacuolar Insoluble Protein Deposit (IPOD).Citation3,9 The term IPOD was coined in 2008 by Judith Frydman and coworkers.Citation3 It was initially identified as a perivacuolar deposition site for terminally aggregated proteins. Identified substrates comprised constantly misfolding proteins such as a temperature-sensitive SUMO-conjugating enzyme ubc9ts and the heterologously expressed “Von Hippel-Lindau tumor suppressor VHL” that cannot fold to it's native state without it's co-factors elongin BC.Citation3 Those factors were not exclusively found at the IPOD, but also at a perinuclear deposition site for soluble misfolded proteins termed JUNQ (juxtanuclear quality control compartment). They accumulated mainly after inhibition of the proteasome.Citation3 Another class of substrates for the IPOD are amyloidogenic proteins.Citation3,9 In contrast to the constantly misfolding substrates, amyloidogenic proteins accumulate constantly at the IPOD even in non-stress conditions and are usually not found in other deposition sites such as JUNQ/INQ or Q-bodies. During isolation of the IPOD formed by the yeast prion domain of the [PSI+] prion determinant Sup35, several proteins that are known to be highly sensitive toward the oxidative modification of carbonylation were co-purified. Corresponding GFP fusions were found to aggregate and partially co-localize with the IPOD upon oxidative stress applied to the cells.Citation9 Besides these substrates, the IPOD was more recently also described to harbor inactive proteasomes.Citation10-12
What mechanisms are known to guide the diverse types of aggregates to the different PQC's? Previous searches for signals and factors that target substrates to the JUNQ/INQ or Q-bodies suggested polyubiquitination as a targeting signal.Citation3 However, it was found not to be essential for deposition of substrates at the JUNQ/INQ4. Another mechanism for proper targeting to the INQ/JUNQ and Q-bodies is the association of misfolded and aggregated proteins with specific targeting factors: the small heat shock protein Hsp42 is required for substrate targeting to Q-bodies.Citation6,7 while the heat shock inducible protein Btn2 and the Hsp40 chaperone Sis1 are involved in targeting to the JUNQ/INQ.Citation4,13,14 Furthermore, Hsp42 was also suggested to target inactive proteasome subunits to the IPOD.Citation10-12 Specific targeting factors for amyloid substrates to the IPOD were previously not known.
ROLE OF ACTIN IN HANDLING OF CELLULAR AGGREGATES
The existence of spatially separated deposition sites for different types of aggregates implies that the cell must be able to differentiate between different aggregates and target them to the designated deposition site. In our recent study,Citation1 we searched for factors that are involved in recruitment of amyloid aggregates to the IPOD. To identify those, we used an amyloid fiber-binding assay. We tagged the prion domain (PrD) of the [PSI+] prion determinant Sup35 with a biotin moiety, produced the protein recombinantly in E.coli and generated amyloid fibers in vitro. Those PrD amyloid fibers were immobilized to an avidin resin, incubated with [PSI+] yeast cell lysates and proteins bound to the fibers were identified by mass spectrometry.Citation1 Those studies revealed that proper recruitment of amyloid aggregates to the IPOD requires, among others, an actin cable-based transport machinery comprising tropomyosin and the actin motor protein Myo2 (compare also ). Transient depletion of these 2 proteins led to a targeting defect of amyloid aggregates to the single perivacuolar IPOD deposition site, which resulted in an accumulation of aggregates in multiple foci dispersed throughout the cytoplasm.Citation1
FIGURE 1. Model for an involvement of vesicular transport proteins in the recruitment of PrD-GFP aggregates and preApe1 to the IPOD and PAS respectively. For details, please see text.
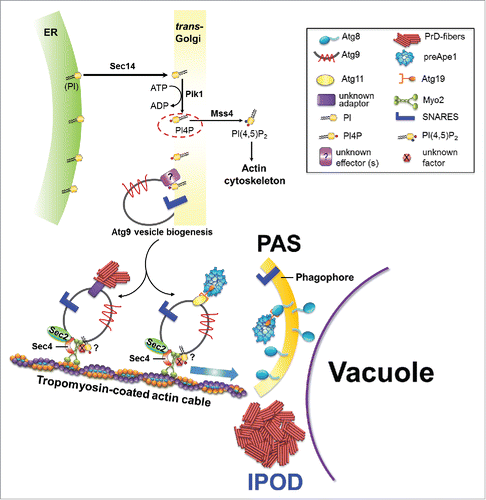
Actin had been implicated a role in the cellular handling of aggregates before. During the de novo induction of yeast prions, interactions of prion aggregates with actin were documented in vivo.Citation15 Later, it was observed that molecules of Sup35 can be linked with actin patches in the cell periphery via the stress-inducible protein Lsb2 that can associate with Sup35 aggregates on the one hand and the actin binding factor Las17 on the other hand.Citation16 Thus Lsb2 might collect and concentrate misfolded Sup35 molecules, resulting in locally high concentrations that facilitate conformational conversion to the prion state. It was suggested that aggregates captured near actin patches through this mechanism might be further directed to perivacuolar protein quality control compartments such as the IPOD. Since de novo formation of another yeast prion termed [RNQ+] was also facilitated by Lsb2, this proposed mechanism may be a general one for amyloidogenic proteins.Citation16 Additionally, actin was also implicated in targeting of other types of aggregates to respective deposition sites when it was observed that an intact actin cytoskeleton was crucial to target constantly misfolding VHL to the JUNQ/INQ and Q-bodies.Citation7 Another hint for a role of the actin cytoskeleton in aggregate handling came from studies investigating the molecular mechanism of asymmetric retention of protein aggregates in mother cells during cell division.Citation17,18 It was found that deletion of several components of the actin polarity machinery including the formin Bni1, actin mutants that cause reduced binding of actin cables to myosin and mutants of Myo2, resulted in a severe reduction of damage asymmetry.Citation19,20 Furthermore, aggregates that had formed in buds of budding mother cells were transported back into the mother cell. This process depended again on the formin Bni1 and the actin cable decorating protein tropomyosin. Both, retention of aggregates in mother cells as well as retrograde clearance of aggregates from the bud involved binding of aggregates associated with the protein disaggregase Hsp104, to actin. These data led to a model where actin cables provide a scaffold for tethering of Hsp104-containing aggregates. Retrograde actin flow away from the polarisome in the bud, initiated through Bni1 dependent actin nucleation, would then allow for net movement of aggregates toward the mother cell.Citation19,20 The role of Myo2 in this model was proposed to be an indirect one, because Myo2 moves along actin cables in the opposite direction as compared with aggregates. It was suggested to be involved in delivery of components to the polarisome, favoring an indirect effect of impairment of Myo2 function on aggregate asymmetry. In similar experiments, it was shown that aggregates of the amyloidogenic polyQ extension Huntingtin variant Htt103Q are also subject to asymmetric inheritance. In this case, the process is also dependent on the formin Bni1, suggesting that it might be retained by the same or a very similar mechanism.Citation20 More recently, higher resolution microscopy (3D-SIM) has confirmed an association of heat-denatured aggregates bound by Hsp104 and dispersed Htt103Q aggregates, with actin cables. The nature of these Htt103Q aggregates, however, remained unclear because these aggregates did not stain with the amyloid dye ThioflavinT,Citation21 opening the possibility that they were of amorphous nature rather than being amyloid like. Furthermore, both types of aggregates co-localized with Myo2 and the actin-organizing protein calmodulin, further substantiating a physical interaction of aggregates with the actin cytoskeleton network.Citation21
AMYLOID RECRUITMENT TO THE IPOD OVERLAPS WITH SUBSTRATE DELIVERY TO THE ADJACENT PHAGOPHORE ASSEMBLY SITE (PAS)
When we studied an involvement of actin cable-based recruitment of amyloids to the IPOD, we observed that the corresponding recruitment machinery strongly overlapped with that for precursor hydrolases on transit to the Phagophore Assembly Site (PAS).Citation1 The PAS is the site where the cell initiates the formation of autophagosomes during macroautophagy and CVT vesicles in the so-called cytoplasm-to-vacuole targeting pathway. A precursor form of the aminopeptidase1 (preApe1) is a major substrate of the CVT pathway. It forms homododecamers that assemble into higher order complexes in the cytoplasm. These complexes are recruited to the PAS where they are encapsulated into autophagosome-related double membrane vesicles termed CVT vesicles that subsequently fuse with the vacuolar membrane to release their content into the lumen for activation through cleavage of the propeptide.Citation22-25 Interestingly, the faithful recruitment of preApe1 complexes to the PAS involves actin cables.Citation26,27 According to a current model,Citation26 preApe1 complexes are recruited to the outside of small vesicles termed Atg9 vesicles via the adaptor Atg11 and the specific receptor Atg19 that can associate with Atg9 vesicles.Citation28,29 These Atg9 vesicles are generated through Golgi-related secretory and endosomal pathways.Citation30-32 They are stored in cytoplasmic reservoirs of 20–30 nm vesicles that can be activated for rapid delivery to the PAS if needed. At the PAS, Atg9 resides in a cluster of vesicles and tubules that can fuse to initiate autophagosome/CVT vesicle formation.Citation31 SNARE proteins are involved in formation and fusion of Atg9 containing vesicles and tubules.Citation33-35 When it was observed that the transmembrane protein Atg9 also failed to reach the PAS upon impairment of the actin cytoskeleton,Citation27 it was proposed that Atg9 vesicles loaded with preApe1 are associated with actin cables through an thitherto unknown factor and move either actively along actin cables or are pushed toward the PAS through nucleation and elongation of the actin cables they associate with.Citation26,36 We observed that the faithful recruitment of preApe1 as well as the PAS marker protein Atg8 to the PAS is also impaired after depletion of the actin cable motor Myo21. This would strongly support the hypothesis that Atg9 vesicles loaded with preApe1 are actively moving along actin cables to the PASCitation26 and identifies Myo2 as the missing factor between Atg9 vesicles and actin cables.Citation1 Such a Myo2 based movement of vesicles along actin cables is in full agreement with the confirmed role for Myo2 in moving vacuolar lobes on actin cables during vacuole inheritance.Citation37
AMYLOID AGGREGATES ARE LIKELY TRANSPORTED TO THE IPOD VIA Atg9 RELATED VESICLES
Depletion of components of the recruitment machinery for substrates to the PAS and IPOD, respectively, led to the co-accumulation of amyloid aggregates with preApe1, Atg8 and Atg9 in the same foci dispersed throughout the cytoplasm.Citation1 It was proposed that the precursor hydrolase complexes are attached to Atg9 vesicles that move along actin cables toward the PAS.Citation26 In such a scenario, cargoes that move along actin cables may simply get stuck at particular cellular sites when the integrity or functionality of the actin tracks is impaired or crucial factors are missing, similar to a traffic jam in front of a construction site. If amyloid aggregates were tethered directly to those actin cables, for example through direct interaction of Hsp104 with aggregates on the one hand and actin cables on the other hand, and moved indirectly through actin cable polymerization,Citation19 it would be curious why they accumulated at the same cellular sites as compared with actively moving Atg9 vesicles. Furthermore, in that case, it would be harder to explain why the depletion of the Myo2 motor protein led to a transport block of amyloid aggregates. Therefore, we hypothesized that amyloid aggregates might be associated with vesicles just like the large preApe1 complexes are. Due to the partial co-localization of PrD-GFP with Atg9, those vesicles might even represent Atg9 containing vesicles. We found several lines of evidence that this might indeed be the case. The first one came from our observation that Myo2 co-localized with PrD-GFP aggregates upon disruption of the recruitment machinery. As a second line of evidence, we observed that the depletion of different proteins involved in vesicular transport processes led to an identical phenotype as compared with Myo2 depletion, namely a block in recruitment of amyloid aggregates to the IPOD and accumulation of the aggregates in multiple dispersed foci. This clearly ties vesicular transport processes with the faithful recruitment of amyloid aggregates to the IPOD (). One of those vesicular transport proteins we identified was Sec 141. It is a phosphatidylinositol/ phosphatidylcholine (PI/PC) transfer proteinCitation38 that associates preferentially to Golgi membranes.Citation39 It transfers phosphatidylinositol (PI) lipid from the ER to the Golgi complex, where PI is phosphorylated to form phosphatidylinositol-4-phosphate (PI4P) by the PI4P kinase, Pik1.Citation38,40,41 It has been shown that the pool of PI4P lipids generated by Pik1 regulates the formation of Golgi-derived secretory vesicles.Citation41-43 Therefore, when Sec 14 or Pik1 function is abolished, the amount of PI4P is reduced and de novo formation of Golgi-derived vesicles is reduced.Citation40-42 Remarkably, Atg9 vesicles are also derived from the Golgi apparatus.Citation30 In line with this, it was shown that inactivation of Pik1 mutants at the nonpermissive temperature blocks the formation of Atg9 vesicles from the Golgi.Citation44 Since Sec 14 acts upstream of Pik1 in the formation of PI4P lipids,Citation40,41 its function is likely also crucial for releasing of Atg9 vesicles from the Golgi. Since we hypothesized that PrD-GFP may be recruited to Atg9 containing vesicles, this would plausibly explain why depletion of Sec 14 disturbs recruitment of PrD-GFP aggregates to the IPOD (). However, it should be noted that Sec 14 may also influence the integrity of actin cables: besides the role of PI4P lipids on the formation of Golgi-derived Atg9 transport vesicles,Citation44 PI4P also serves as a substrate for the PIP5-kinase Mss4 to generate PI(4,5)P2 lipids, which are required for the proper organization of the actin cytoskeleton.Citation40,45 In agreement with this, it was shown that impaired production of PI4P in pik1–101 mutant cells results in less PI(4,5)P2 generation, which causes disruption (depolarization) of actin cables.Citation43 Upon Sec 14 depletion, PI is not transported properly to Pik1. This may eventually also reduce the levels of PI(4,5)P2 lipids, causing an impairment of the actin cytoskeleton, which in turn could also contribute to impaired recruitment of PrD-GFP to the IPOD (). Furthermore, the PI4P lipids whose levels are reduced upon Sec 14 depletion, have an additional role in actin cable-based transport of vesicles. It was shown that the association of secretory vesicles with Myo2 for their transport along actin cables to the plasma membrane requires vesicle bound PI4P.Citation46 Since Atg9 vesicles also originate from the trans Golgi compartment,Citation30-32 it appears possible that PI4P is also important for binding of Myo2 to Atg9 containing vesicles that mediate recruitment of preApe1 and presumably also amyloid aggregates to the PAS and IPOD, respectively ().
A second protein whose depletion gave the same phenotype of impaired recruitment of amyloid aggregates to the IPOD as compared with Myo2 was the SNARE chaperone Sec 1847 that acts in disassembly of SNARE proteins and is crucial for different vesicular fusion and transport processes. Stunningly, Sec 18 and SNARE protein function is known to be required for autophagy and the CVT pathway.Citation33,35,48 Moreover, it was also found to be involved in establishing aggregate inheritance asymmetry during cell division.Citation21 As generation and trafficking of Atg9 vesicles to the PAS requires the action of several SNARE proteins,Citation33 it seems plausible that the effect of Sec 18 depletion is due to reduced trafficking of Atg9 vesicles to the PAS/IPOD.
Finally, some interesting additional findings support the hypothesis that Atg9 related vesicles are involved in recruitment of amyloid aggregates to the IPOD: Sec 4, a Rab family GTPase that is essential for autophagy and that is involved in anterograde transport of Atg9 vesicles to the PAS,Citation49 was found to bind to immobilized PrD fibers.Citation1 Since Sec 4 can associate with Myo2 during the transport of secretory vesicles along actin cables to the plasma membrane,Citation46,50 it may have a similar role in Atg9 containing vesicles (), which would explain why it is important for anterograde transport of Atg9 vesicles to the PAS. Furthermore, Ykt6, a v-SNARE protein that has been implicated in different vesicle trafficking pathways including the CVT pathway and Atg9 vesicle traffickingCitation33 also bound to immobilized PrD fibers. Thus we may have found these proteins to bind to immobilized PrD fibersCitation1 because they are components of the vesicles that recruit PrD-GFP and guide it to the IPOD (). In conclusion, many of these findings point to a direct recruitment of amyloid aggregates to Atg9 related vesicles. A more direct proof for this could come from direct visualization of amyloid aggregates with vesicles by EM in the future.
ASSOCIATION OF AGGREGATES WITH VESICULAR STRUCTURES
The concept proposing that vesicular transport is involved in the recruitment of aggregates to different aggregate deposition sites is rather new, but various previous studies exist that report an association of aggregates with membranes. Htt103 aggregates for example were shown to associate with endocytic membranes, thereby impairing endocytic processes.Citation51 Moreover, interaction of Htt103Q with endocytic membranes facilitated its aggregation.Citation52 Furthermore, cytosolic aggregates were found to be associated with the ER and mitochondria, probably constraining aggregate mobility and facilitating retention of aggregates in mother cells during cell division.Citation6,53
In a recent study, Hill et al investigated the mechanism of mother cell-biased segregation of aggregates.Citation2 The authors observed that Hsp104-bound cytosolic aggregates co-precipitated with several proteins involved in vesicular trafficking. More specifically, the 4 components of the vacuole inheritance machinery, Act1, Myo2, Vac17 and Vac8 as well as the yeast dynamin homolog Vps1 were identified to be involved in this process. Moreover, the authors observed that the deletion of Vac17 and Vps1 did not only impair retention of heat-denatured aggregates in the mother cells, but also disturbed the recruitment of these aggregates to a perivacuolar inclusion reminiscent of the IPOD. In line with this, the study also reported that Vac17 overexpression boosted fusion of small aggregates into larger perivacuolar assemblies. It was therefore proposed that aggregates bound by Hsp104 might interact with membrane vesicles, thereby hitchhiking on trafficking routes leading to the vacuole.Citation2 Interestingly, the deletion of Vac17 did not effect the recruitment of the amyloidogenic IPOD substrate Htt103Q.Citation2 This different dependency of heat-denatured and amyloidogenic aggregates on the Vac17 function for recruitment to IPOD like inclusions prompted us to ask whether the recruitment machineries for different types of aggregates differ. To approach this question, we tested whether deletion of Vac8, Vac17 or Vps1 also affected the transport of the model amyloid substrate PrD-GFP to the IPOD. Thus we deleted each of these proteins in a strain expressing PrD-GFP under control of the Gal1 promoter. After 6 hours of induction of PrD-GFP expression with galactose, the protein was exclusively found at a perivacuolar IPOD site in the absence of Vac8 or Vac17, just like in a wild type control strain (). To our big surprise, however, the deletion of Vps1 did cause a severe recruitment defect for PrD-GFP to the IPOD (), just like it did for heat-denatured proteins in the study by Hill et al.Citation2 Thus our data on Vac8 and Vac17 deletion fully confirm the previous resultsCitation2 that these proteins do not influence the recruitment of amyloidogenic proteins to the IPOD to a measureable extend. A possible explanation for these results could be that both types of aggregates, heat-denatured proteins and amyloids, use different types of vesicles with different adaptors for their recruitment to IPOD-like inclusions, but both types of vesicles require the dynamin homolog Vps1. Additional future studies, however, have to unravel this issue in more molecular detail to confirm or disprove this hypothesis. Nonetheless, the key finding that recruitment of heat-denatured protein aggregates to a perivacuolar deposition site involves proteins mediating vesicular transportCitation2 fully supports our concept that vesicular transport is crucial for the sorting of amyloid aggregates to the perivacuolar deposition site IPOD.Citation1
FIGURE 2. Vps1, but not Vac8 and Vac17, is involved in the sorting of PrD-GFP aggregates to the IPOD. (A) PrD-GFP was integrated into the genome under the galactose-inducible promoter in a [PSI+] wild-type (wt) strain or a strain with a deletion of Vac8, Vac17 or Vps1. Cells were grown overnight in YPD and induced with galactose for 6 hours at an OD600 of 0.15. Cells were then fixed with 4 % paraformaldehyde (PFA) and analyzed by fluorescence microscopy (xcellence IX81Olympus). Images were acquired with a 100X /NA 1.45 oil immersion objective as Z-stacks with an optical section of 0.2 µm. An overlay of the acquired z-stacks (maximum intensity projection) was then processed with the ImageJ software. Scale bar, 2 µm. (B) Quantification of PrD-GFP foci in the wild-type strain and strains with the deletion of Vac8, Vac17 or Vps1 is shown. Frequencies of cells with one single focus or more than one foci are given in %; n = number of cells analyzed for quantification.
![FIGURE 2. Vps1, but not Vac8 and Vac17, is involved in the sorting of PrD-GFP aggregates to the IPOD. (A) PrD-GFP was integrated into the genome under the galactose-inducible promoter in a [PSI+] wild-type (wt) strain or a strain with a deletion of Vac8, Vac17 or Vps1. Cells were grown overnight in YPD and induced with galactose for 6 hours at an OD600 of 0.15. Cells were then fixed with 4 % paraformaldehyde (PFA) and analyzed by fluorescence microscopy (xcellence IX81Olympus). Images were acquired with a 100X /NA 1.45 oil immersion objective as Z-stacks with an optical section of 0.2 µm. An overlay of the acquired z-stacks (maximum intensity projection) was then processed with the ImageJ software. Scale bar, 2 µm. (B) Quantification of PrD-GFP foci in the wild-type strain and strains with the deletion of Vac8, Vac17 or Vps1 is shown. Frequencies of cells with one single focus or more than one foci are given in %; n = number of cells analyzed for quantification.](/cms/asset/831dc1f1-588c-4159-b7f8-78c1d33ddc45/kprn_a_1293226_f0002_c.gif)
DISCLOSURE OF POTENTIAL CONFLICTS OF INTEREST
No potential conflicts of interest were disclosed.
ACKNOWLEDGMENTS
We thank the Imaging Facility of the Center for Molecular Biology Heidelberg (ZMBH) headed by Dr. Holger Lorenz for assistance with microscopic analyses. We also thank Prof. Bernd Bukau and members of his lab and Prof. Peter P. Nawroth and members of his lab for helpful discussions during the project.
FUNDING
This study was supported by the Deutsche Forschungsgemeinschaft to Jens Tyedmers (TY93/1–1).
REFERENCES
- Kumar R, Nawroth PP, Tyedmers J. Prion aggregates are recruited to the insoluble protein deposit (IPOD) via myosin 2-based vesicular transport. PLoS Genet 2016; 12:e1006324; PMID:27689885; http://dx.doi.org/10.1371/journal.pgen.1006324
- Hill SM, Hao X, Gronvall J, Spikings-Nordby S, Widlund PO, Amen T, Jörhov A, Josefson R, Kaganovich D, Liu B, et al. Asymmetric inheritance of aggregated proteins and age reset in yeast are regulated by Vac17-dependent vacuolar functions. Cell Rep 2016; 16:826-38; PMID:27373154
- Kaganovich D, Kopito R, Frydman J. Misfolded proteins partition between two distinct quality control compartments. Nature 2008; 454:1088-95; PMID:18756251; http://dx.doi.org/10.1038/nature07195
- Miller SB, Ho CT, Winkler J, Khokhrina M, Neuner A, Mohamed MY, Guilbride DL, Richter K, Lisby M, Schiebel E, et al. Compartment-specific aggregases direct distinct nuclear and cytoplasmic aggregate deposition. EMBO J 2015; 34:778-97; PMID:25672362; http://dx.doi.org/10.15252/embj.201489524
- Gallina I, Colding C, Henriksen P, Beli P, Nakamura K, Offman J, Mathiasen DP, Silva S, Hoffmann E, Groth A, et al. Cmr1/WDR76 defines a nuclear genotoxic stress body linking genome integrity and protein quality control. Nat Commun 2015; 6:6533; PMID:25817432; http://dx.doi.org/10.1038/ncomms7533
- Escusa-Toret S, Vonk WI, Frydman J. Spatial sequestration of misfolded proteins by a dynamic chaperone pathway enhances cellular fitness during stress. Nat Cell Biol 2013; 15:1231-43; PMID:24036477; http://dx.doi.org/10.1038/ncb2838
- Specht S, Miller SB, Mogk A, Bukau B. Hsp42 is required for sequestration of protein aggregates into deposition sites in Saccharomyces cerevisiae. J Cell Biol 2011; 195:617-29; PMID:22065637; http://dx.doi.org/10.1083/jcb.201106037
- Spokoini R, Moldavski O, Nahmias Y, England JL, Schuldiner M, Kaganovich D. Confinement to organelle-associated inclusion structures mediates asymmetric inheritance of aggregated protein in budding yeast. Cell Rep 2012; 2:738-47; PMID:23022486; http://dx.doi.org/10.1016/j.celrep.2012.08.024
- Tyedmers J, Treusch S, Dong J, McCaffery JM, Bevis B, Lindquist S. Prion induction involves an ancient system for the sequestration of aggregated proteins and heritable changes in prion fragmentation. Proc Natl Acad Sci U S A 2010; 107:8633-8; PMID:20421488; http://dx.doi.org/10.1073/pnas.1003895107
- Peters LZ, Karmon O, David-Kadoch G, Hazan R, Yu T, Glickman MH, Ben-Aroya S. The protein quality control machinery regulates its misassembled proteasome subunits. PLoS Genet 2015; 11:e1005178; PMID:25919710; http://dx.doi.org/10.1371/journal.pgen.1005178
- Peters LZ, Karmon O, Miodownik S, Ben-Aroya S. Proteasome storage granules are transiently associated with the insoluble protein deposit in Saccharomyces cerevisiae. J Cell Sci 2016; 129:1190-7; PMID:26826189; http://dx.doi.org/10.1242/jcs.179648
- Marshall RS, McLoughlin F, Vierstra RD. Autophagic turnover of inactive 26s proteasomes in yeast is directed by the ubiquitin receptor Cue5 and the Hsp42 chaperone. Cell Rep 2016; 16:1717-32; PMID:27477278; http://dx.doi.org/10.1016/j.celrep.2016.07.015
- Malinovska L, Kroschwald S, Munder MC, Richter D, Alberti S. Molecular chaperones and stress-inducible protein-sorting factors coordinate the spatiotemporal distribution of protein aggregates. Mol Biol Cell 2012; 23:3041-56; PMID:22718905; http://dx.doi.org/10.1091/mbc.E12-03-0194
- Park SH, Kukushkin Y, Gupta R, Chen T, Konagai A, Hipp MS, Hayer-Hartl M, Hartl FU. PolyQ proteins interfere with nuclear degradation of cytosolic proteins by sequestering the Sis1p chaperone. Cell 2013; 154:134-45; PMID:23791384; http://dx.doi.org/10.1016/j.cell.2013.06.003
- Ganusova EE, Ozolins LN, Bhagat S, Newnam GP, Wegrzyn RD, Sherman MY, Chernoff YO. Modulation of prion formation, aggregation, and toxicity by the actin cytoskeleton in yeast. Mol Cell Biol 2006; 26:617-29; PMID:16382152; http://dx.doi.org/10.1128/MCB.26.2.617-629.2006
- Chernova TA, Romanyuk AV, Karpova TS, Shanks JR, Ali M, Moffatt N, Howie RL, O'Dell A, McNally JG, Liebman SW, et al. Prion induction by the short-lived, stress-induced protein Lsb2 is regulated by ubiquitination and association with the actin cytoskeleton. Mol Cell 2011; 43:242-52; PMID:21777813; http://dx.doi.org/10.1016/j.molcel.2011.07.001
- Aguilaniu H, Gustafsson L, Rigoulet M, Nystrom T. Asymmetric inheritance of oxidatively damaged proteins during cytokinesis. Science 2003; 299:1751-3; PMID:12610228; http://dx.doi.org/10.1126/science.1080418
- Erjavec N, Larsson L, Grantham J, Nystrom T. Accelerated aging and failure to segregate damaged proteins in Sir2 mutants can be suppressed by overproducing the protein aggregation-remodeling factor Hsp104p. Genes Dev 2007; 21:2410-21; http://dx.doi.org/10.1101/gad.439307
- Liu B, Larsson L, Caballero A, Hao X, Oling D, Grantham J, Nyström T. The polarisome is required for segregation and retrograde transport of protein aggregates. Cell 2010; 140:257-67; PMID:20141839; http://dx.doi.org/10.1016/j.cell.2009.12.031
- Liu B, Larsson L, Franssens V, Hao X, Hill SM, Andersson V, Höglund D, Song J, Yang X, Öling D, et al. Segregation of protein aggregates involves actin and the polarity machinery. Cell 2011; 147:959-61; PMID:22118450; http://dx.doi.org/10.1016/j.cell.2011.11.018
- Song J, Yang Q, Yang J, Larsson L, Hao X, Zhu X, Malmgren-Hill S, Cvijovic M, Fernandez-Rodriguez J, Grantham J, et al. Essential genetic interactors of SIR2 required for spatial sequestration and asymmetrical inheritance of protein aggregates. PLoS Genet 2014; 10:e1004539; PMID:25079602; http://dx.doi.org/10.1371/journal.pgen.1004539
- Kim J, Scott SV, Oda MN, Klionsky DJ. Transport of a large oligomeric protein by the cytoplasm to vacuole protein targeting pathway. J Cell Biol 1997; 137:609-18; PMID:9151668; http://dx.doi.org/10.1083/jcb.137.3.609
- Klionsky DJ, Cueva R, Yaver DS. Aminopeptidase I of Saccharomyces cerevisiae is localized to the vacuole independent of the secretory pathway. J Cell Biol 1992; 119:287-99; PMID:1400574; http://dx.doi.org/10.1083/jcb.119.2.287
- Lynch-Day MA, Klionsky DJ. The Cvt pathway as a model for selective autophagy. FEBS Lett 2010; 584:1359-66; PMID:20146925; http://dx.doi.org/10.1016/j.febslet.2010.02.013
- Suzuki K. Selective autophagy in budding yeast. Cell Death Differ 2013; 20:43-8; PMID:22705847; http://dx.doi.org/10.1038/cdd.2012.73
- Monastyrska I, Rieter E, Klionsky DJ, Reggiori F. Multiple roles of the cytoskeleton in autophagy. Biol Rev Camb Philos Soc 2009; 84:431-48; PMID:19659885; http://dx.doi.org/10.1111/j.1469-185X.2009.00082.x
- Reggiori F, Monastyrska I, Shintani T, Klionsky DJ. The actin cytoskeleton is required for selective types of autophagy, but not nonspecific autophagy, in the yeast Saccharomyces cerevisiae. Mol Biol Cell 2005; 16:5843-56; PMID:16221887; http://dx.doi.org/10.1091/mbc.E05-07-0629
- Kakuta S, Yamamoto H, Negishi L, Kondo-Kakuta C, Hayashi N, Ohsumi Y. Atg9 vesicles recruit vesicle-tethering proteins Trs85 and Ypt1 to the autophagosome formation site. J Biol Chem 2012; 287:44261-9; PMID:23129774; http://dx.doi.org/10.1074/jbc.M112.411454
- He C, Song H, Yorimitsu T, Monastyrska I, Yen WL, Legakis JE, Klionsky DJ. Recruitment of Atg9 to the preautophagosomal structure by Atg11 is essential for selective autophagy in budding yeast. J Cell Biol 2006; 175:925-35; PMID:17178909; http://dx.doi.org/10.1083/jcb.200606084
- Yamamoto H, Kakuta S, Watanabe TM, Kitamura A, Sekito T, Kondo-Kakuta C, Ichikawa R, Kinjo M, Ohsumi Y. Atg9 vesicles are an important membrane source during early steps of autophagosome formation. J Cell Biol 2012; 198:219-33; PMID:22826123; http://dx.doi.org/10.1083/jcb.201202061
- Mari M, Griffith J, Rieter E, Krishnappa L, Klionsky DJ, Reggiori F. An Atg9-containing compartment that functions in the early steps of autophagosome biogenesis. J Cell Biol 2010; 190:1005-22; PMID:20855505; http://dx.doi.org/10.1083/jcb.200912089
- Ohashi Y, Munro S. Membrane delivery to the yeast autophagosome from the Golgi-endosomal system. Mol Biol Cell 2010; 21:3998-4008; PMID:20861302; http://dx.doi.org/10.1091/mbc.E10-05-0457
- Nair U, Jotwani A, Geng J, Gammoh N, Richerson D, Yen WL, Griffith J, Nag S, Wang K, Moss T, et al. SNARE proteins are required for macroautophagy. Cell 2011; 146:290-302; PMID:21784249; http://dx.doi.org/10.1016/j.cell.`2011.06.022
- Nair U, Klionsky DJ. Autophagosome biogenesis requires SNAREs. Autophagy 2011; 7:1570-2; PMID:22024744; http://dx.doi.org/10.4161/auto.7.12.18001
- Moreau K, Ravikumar B, Renna M, Puri C, Rubinsztein DC. Autophagosome precursor maturation requires homotypic fusion. Cell 2011; 146:303-17; PMID:21784250; http://dx.doi.org/10.1016/j.cell.2011.06.023
- Monastyrska I, He C, Geng J, Hoppe AD, Li Z, Klionsky DJ. Arp2 links autophagic machinery with the actin cytoskeleton. Mol Biol Cell 2008; 19:1962-75; PMID:18287533; http://dx.doi.org/10.1091/mbc.E07-09-0892
- Hammer JA, 3rd, Sellers JR. Walking to work: roles for class V myosins as cargo transporters. Nat Rev Mol Cell Biol 2011; 13:13-26; PMID:22146746; http://dx.doi.org/10.1038/ncb2135
- Bankaitis VA, Aitken JR, Cleves AE, Dowhan W. An essential role for a phospholipid transfer protein in yeast Golgi function. Nature 1990; 347:561-2; PMID:2215682; http://dx.doi.org/10.1038/347561a0
- Cleves AE, McGee TP, Whitters EA, Champion KM, Aitken JR, Dowhan W, Goebl M, Bankaitis VA. Mutations in the CDP-choline pathway for phospholipid biosynthesis bypass the requirement for an essential phospholipid transfer protein. Cell 1991; 64:789-800; PMID:1997207; http://dx.doi.org/10.1016/0092-8674(91)90508-V
- Grabon A, Khan D, Bankaitis VA. Phosphatidylinositol transfer proteins and instructive regulation of lipid kinase biology. Biochim Biophys Acta 2015; 1851:724-35; PMID:25592381; http://dx.doi.org/10.1016/j.bbalip.2014.12.011
- Hama H, Schnieders EA, Thorner J, Takemoto JY, DeWald DB. Direct involvement of phosphatidylinositol 4-phosphate in secretion in the yeast Saccharomyces cerevisiae. J Biol Chem 1999; 274:34294-300; PMID:10567405; http://dx.doi.org/10.1074/jbc.274.48.34294
- Fang M, Kearns BG, Gedvilaite A, Kagiwada S, Kearns M, Fung MK, Bankaitis VA. Kes1p shares homology with human oxysterol binding protein and participates in a novel regulatory pathway for yeast Golgi-derived transport vesicle biogenesis. EMBO J 1996; 15:6447-59; PMID:8978672
- Walch-Solimena C, Novick P. The yeast phosphatidylinositol-4-OH kinase pik1 regulates secretion at the Golgi. Nat Cell Biol 1999; 1:523-5; PMID:10587649; http://dx.doi.org/10.1038/70319
- Wang K, Yang Z, Liu X, Mao K, Nair U, Klionsky DJ. Phosphatidylinositol 4-kinases are required for autophagic membrane trafficking. J Biol Chem 2012; 287:37964-72; PMID:22977244; http://dx.doi.org/10.1074/jbc.M112.371591
- Desrivieres S, Cooke FT, Parker PJ, Hall MN. MSS4, a phosphatidylinositol-4-phosphate 5-kinase required for organization of the actin cytoskeleton in Saccharomyces cerevisiae. J Biol Chem 1998; 273:15787-93; PMID:9624178; http://dx.doi.org/10.1074/jbc.273.25.15787
- Santiago-Tirado FH, Legesse-Miller A, Schott D, Bretscher A. PI4P and Rab inputs collaborate in myosin-V-dependent transport of secretory compartments in yeast. Dev Cell 2011; 20:47-59; PMID:21238924; http://dx.doi.org/10.1016/j.devcel.2010.11.006
- Mayer A, Wickner W, Haas A. Sec 18p (NSF)-driven release of Sec 17p (alpha-SNAP) can precede docking and fusion of yeast vacuoles. Cell 1996; 85:83-94; PMID:8620540; http://dx.doi.org/10.1016/S0092-8674(00)81084-3
- Ishihara N, Hamasaki M, Yokota S, Suzuki K, Kamada Y, Kihara A, Yoshimori T, Noda T, Ohsumi Y. Autophagosome requires specific early Sec proteins for its formation and NSF/SNARE for vacuolar fusion. Mol Biol Cell 2001; 12:3690-702; PMID:11694599; http://dx.doi.org/10.1091/mbc.12.11.3690
- Geng J, Nair U, Yasumura-Yorimitsu K, Klionsky DJ. Post-Golgi Sec proteins are required for autophagy in Saccharomyces cerevisiae. Mol Biol Cell 2010; 21:2257-69; PMID:20444978; http://dx.doi.org/10.1091/mbc.E09-11-0969
- Jin Y, Sultana A, Gandhi P, Franklin E, Hamamoto S, Khan AR, Munson M, Schekman R, Weisman LS. Myosin V transports secretory vesicles via a Rab GTPase cascade and interaction with the exocyst complex. Dev Cell 2011; 21:1156-70; PMID:22172676; http://dx.doi.org/10.1016/j.devcel.2011.10.009
- Meriin AB, Zhang X, Miliaras NB, Kazantsev A, Chernoff YO, McCaffery JM, Wendland B, Sherman MY. Aggregation of expanded polyglutamine domain in yeast leads to defects in endocytosis. Mol Cell Biol 2003; 23:7554-65; PMID:14560003; http://dx.doi.org/10.1128/MCB.23.21.7554-7565.2003
- Meriin AB, Zhang X, Alexandrov IM, Salnikova AB, Ter-Avanesian MD, Chernoff YO, Sherman MY. Endocytosis machinery is involved in aggregation of proteins with expanded polyglutamine domains. FASEB J 2007; 21:1915-25; PMID:17341688; http://dx.doi.org/10.1096/fj.06-6878com
- Zhou C, Slaughter BD, Unruh JR, Guo F, Yu Z, Mickey K, Narkar A, Ross RT, McClain M, Li R. Organelle-based aggregation and retention of damaged proteins in asymmetrically dividing cells. Cell 2014; 159:530-42; PMID:25417105; http://dx.doi.org/10.1016/j.cell.2014.09.026