ABSTRACT
The Hsp70 chaperone machinery is a key component of the heat-shock response and a modulator of prion propagation in yeast. A major factor in optimizing Hsp70 function is the highly coordinated activities of the nucleotide-binding and substrate-binding domains of the protein. Hsp70 inter-domain communication occurs through a bidirectional allosteric interaction network between the two domains. Recent findings identified the β6/β7 region of the substrate-binding domain as playing a critical role in optimizing Hsp70 function in both the stress response and prion propagation and highlighted the allosteric interaction interface between the domains. Importantly, while functional changes in Hsp70 can result in phenotypic consequences for both the stress response and prion propagation, there can be significant differences in the levels of phenotypic impact that such changes illicit.
Introduction
The Hsp70 family of chaperones is evolutionarily conserved and widely distributed in the cytoplasm, mitochondrion, nucleus, endoplasmic reticulum and other cellular compartments in both prokaryotes and eukaryotes [Citation1]. Structurally, Hsp70 is comprised of two domains: an N-terminal nucleotide binding domain (NBD) and a C-terminal substrate-binding domain (SBD). The SBD is further subdivided into a β-sheet rich substrate-binding domain (SBDβ) with peptide-binding sites, an α-helical lid region (SBDα) located over the substrate-binding cavity and a disordered extreme C-terminal tail of around 30 residues [Citation2–Citation5]. Hsp70 structure is modified by ATP hydrolysis and substrate binding, and consequently mediates versatile functions, such as heat shock response, protein folding, refolding of misfolded proteins, protein translocation and signal transduction [Citation6–Citation10].
Prions are oligomerized and highly ordered aggregates of misfolded protein, which are the cause of neurodegenerative diseases classified as transmissible spongiform encephalopathies (TSEs). Prions have also been implicated in other neurodegenerative diseases such as Parkinson’s disease, Alzheimer’s disease and Huntington’s disease [Citation11]. In light of this complexity, it is perhaps not surprising that regulation of prion propagation has been linked to the heat-shock response as the cellular chaperone machinery contributes to maintaining protein homeostasis and protecting cells from the consequences of exposure to stress, such as protein denaturation [Citation12]. Hsp70 is a central component of the chaperone network playing a key role in mediating the propagation of prions and protection from cellular stress [Citation13].
Yeast has been widely used as a model organism for investigating Hsp70 in relation to prion propagation and heat-shock response [Citation14–Citation17]. A multitude of mutants in the yeast cytosolic Hsp70 Ssa1 have been isolated and characterized to varying degrees, which exhibit effects upon [PSI+] prion propagation or heat-shock response or both [Citation16–Citation18]. Additionally, over-expression of Ssa1 or mild heat shock can impair prion propagation [Citation19,Citation20].
Yeast has proved exceptionally useful for investigating the subtle differences that exist in the ability of highly homologous Hsp70s to propagate prions. Inherent differences exist among yeast and other fungal Hsp70 proteins in the ability to propagate [PSI+], with constitutively expressed Hsp70s being more adapted to this function compared to inducible Hsp70s [Citation21–Citation23]. Differences also exist in constitutively expressed yeast Hsp70s in the ability to propagate prions, as when Ssa1 is the sole cytosolic Hsp70 in the cell, the [URE3] prion is unable to propagate. However, the result is different when Ssa2 is the sole source of Hsp70, even though these proteins are 96% identical and have only 14 amino acid differences out of 641 [Citation24,Citation25]. It has been shown that mutating the A83 residue in Ssa1 to G83 as in Ssa2 or vice versa, allows the prion propagation properties of the proteins to switch [Citation26]. It is important to note that following genome sequencing, the yeast strain 74-D694, which is commonly used in prion propagation studies, possesses a naturally occurring A83G polymorphism in Ssa1, meaning that in prion propagation terms this strain could be viewed as possessing two copies of Ssa2 [Citation27].
Allosteric communication within the SBD of Hsp70 mediates [PSI+] propagation and heat-shock response
ATP hydrolysis is a key component of Hsp70 function and regulates the binding of peptide substrate. Control of Hsp70 ATPase is through a bidirectional allosteric intramolecular regulation between the NBD and the SBD [Citation2,Citation28–Citation31]. Previous genetic studies focusing on Ssa1 and Ssa2 have identified a large number of mutations within the NBD that could impair prion propagation [Citation16,Citation18], but none of these mutants had a significant effect upon ability to grow at higher temperatures (at least at 37°C); and suggested the possibility of decoupling Hsp70 functionality in heat shock and prion propagation. Although located in the SBD the relatively well-characterized L483W mutation has been shown to have an elevated level of ATPase activity, suggesting a possible defect in inter-domain communication as the root cause for phenotypic changes in this mutant [Citation32,Citation33], which could result in changes in substrate-binding activity also [Citation15,Citation16,Citation34].
Recently, through an SBD-targeted mutagenesis approach, the F475S mutant was isolated [Citation17]. Unlike previous Hsp70-Ssa mutants, F475S exhibited defects in prion propagation and growth at 37°C. The locations of both F475S and L483W in the highly conserved β6/β7 region of the SBD highlight the importance of these β-strands in the fine-tuning of the domain in peptide-binding and Hsp70 functionality. The phenotype of F475S suggests that SBD allostery is a critical factor in both [PSI+] propagation and the heat-shock response.
Further clues to functional changes in F475S came from the isolation of three second-site suppressor mutations, A394V, P433S and V477I, that could each alleviate the temperature sensitive phenotype to varying degrees [Citation17]. Combining second site suppressors of L483W [A519T, E540K or P636S] with F475S resulted in restoration of prion propagation for P636S, but the cells remained temperature sensitive; and F475S was lethal when combined with A519T or E540K (unpublished data). Although P636S can restore prion propagation, most likely due to changes in TPR co-chaperone interactions (Jones and Masison 2003), overall these results suggest that the mechanism for prion impairment by F475S and L483W is not completely the same. Disruption of the hydrophobic core of the SBD by F475S induces instability and protein degradation, which is partially stabilized by each of the second-site suppressors [Citation17]. The structural instability of the F475S mutant compared to L483W, and the exacerbation of this instability when combined with second-site suppressors A519T and E540K, is the most likely reason for synthetic lethality of these mutants. Although F475S is inherently unstable compared to wild-type protein, the abundance of Hsp70 is not the primary cause for the phenotypic changes seen with [PSI+] propagation. Although A394V is the most efficient at stabilizing Ssa1, this does not result in increased protein abundance or improved prion propagation [Citation17]. Therefore, it is more likely that changes in SBD allosteric functionality are predominantly responsible for the phenotypic changes observed in F475S, particularly in relation to prion propagation.
Of the three second-site suppressors (A394V/F475S, P433S/F475S and V477I/F475S), A394V is of special interest. As reported, the F475S SBD is largely unfolded in vitro. The viability of F475S Ssa1 at 30°C indicates that this unfolding may be rescued by its partners in vivo. The rescue by V477I of the unfolding of F475S in vitro and in vivo may be attributed to its larger side chain, providing one more methyl group to the hydrophobic core, which blocks the pathway of water into the core where F475 is located (Figure 1(a)). The most significant rescue effect of A394V, which provides two additional methyl groups to the hydrophobic core, further supports this hypothesis. Unlike P433S/F475S and V477I/F475S, the A394V/F475S SBD has a similar SEC elution volume and other structural characteristics as wild-type protein [Citation17], indicating that differences of temperature sensitivity and prion propagation phenotypes between A394V/F475S and WT are mainly caused by the impairment of interdomain communication in A394V/F475S. Although A394V stabilizes the structure of F475S, the local structure loses flexibility to support interdomain communication. The rescue of P433S/F475S is somewhat more mysterious, as P433 is far from F475S. It is interesting that the corresponding residue to Ssa1 P433 is a serine in DnaK, and the local conformations at the site of P433 in DnaK and Ssa1 are similar (). Thus, the effect of the mutation P433S may not be caused by conformational change, but rather to an effect on remote allosteric communication in the SBD domain.
Figure 1. (a) Ssa1 SBD structure (PDB code 5Z8Q). (b), Local conformation at the site of P433 (Ssa1)/S434 (DnaK). Cyan, Ssa1 P433; yellow, DnaK S434.
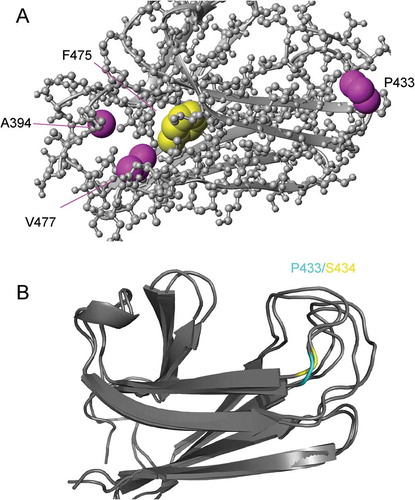
The importance of changes in remote allosteric communication in the β6/β7 region mutants is supported by NMR analysis of L483W. Chemical Shift Perturbations (CSPs) were identified in five regions for L483W compared to WT; (i) V393 in the linker; (ii) I417 in loop 23; (iii) D476, V477, D478, S479, N480, I482, L483, and N484 in β6, loop 67 and β7; (iv) N502, D503, and K504 in β8; and (v) I512, I533, K547, and I548 in SBDα [Citation17]. CSPs identified in region v are located in the α-helical lid and could directly influence the opening and/or closing of the lid. Additionally, CSP regions i-iv clearly formed an interface between the NBD and the SBD. Alanine-scanning mutagenesis across these five regions produced mutants with a variety of temperature-sensitive and prion propagation phenotypes that demonstrated that while the same functional changes in Hsp70 can influence both prion propagation and heat-shock roles of this chaperone, the degree of phenotypic influence can vary widely for specific mutants. Furthermore, these results may provide further insight into the residue to residue ‘conformational signaling pathway’ in the SBD from the substrate binding site to the NBD-SBD docking site.
Conclusion
Saccharomyces cerevisiae has proven to be an exceptionally valuable model system for investigating the role of the Hsp70 chaperone machinery in prion propagation and the heat-shock response. While it is clear that the same functional properties of Hsp70 are key players in both protein folding and prion maintenance, it is now apparent that the degree of functional change that occurs to the Hsp70 chaperone machinery can have disproportionate effects on protein folding activity and prion propagation. Therefore, while the functionality of Hsp70 is the same for protein folding and prion propagation, the phenotypic outcomes to changes of this functionality are different. The real possibility exists that if we fully understand the mechanistic relationship that exists between Hsp70 and the variety of functional roles that is carried out in the cell, the ability to moderate Hsp70 functionality would allow therapeutic targeting of this protein to alter specific phenotypic outcomes associated with a diseased state.
Disclosure statement
No potential conflict of interest was reported by the authors.
Additional information
Funding
References
- Boorstein WR, Ziegelhoffer T, Craig EA. Molecular evolution of the HSP70 multigene family. J Mol Evol. 1994;38:1–17.
- Kityk R, Kopp J, Sinning I, et al. Structure and dynamics of the ATP-bound open conformation of HSP70 chaperones. Mol Cell. 2012;48:863–874.
- Osipiuk J, Walsh MA, Freeman BC, et al. Structure of a new crystal form of human HSP70 ATPase domain. Acta Crystallogr D Biol Crystallogr. 1999;55:1105–1107.
- Stevens SY, Cai S, Pellecchia M, et al. The solution structure of the bacterial HSP70 chaperone protein domain DnaK(393-507) in complex with the peptide NRLLLTG. Protein Sci. 2003;12:2588–2596.
- Zhu X, Zhao X, Burkholder WF, et al. Structural analysis of substrate binding by the molecular chaperone DnaK. Science. 1996;272:1606–1614.
- Bettencourt BR, Feder ME, Cavicchi S. Experimental evolution of Hsp70 expression and thermotolerance in drosophila melanogaster. Evolution. 1999;53:484–492.
- Pratt WB, Toft DO. Regulation of signaling protein function and trafficking by the hsp90/hsp70-based chaperone machinery. Exp Biol Med (Maywood). 2003;228:111–133.
- Ryan MT, Pfanner N. Hsp70 proteins in protein translocation. Adv Protein Chem. 2001;59:223–242.
- Wruck F, Avellaneda MJ, Koers EJ, et al. Protein folding mediated by trigger factor and Hsp70: new insights from single-molecule approaches. J Mol Biol. 2018;430:438–449.
- Young JC, Barral JM, Ulrich Hartl F. More than folding: localized functions of cytosolic chaperones. Trends Biochem Sci. 2003;28:541–547.
- Knowles TP, Vendruscolo M, Dobson CM. The amyloid state and its association with protein misfolding diseases. Nat Rev Mol Cell Biol. 2014;15:384–396.
- Vabulas RM, Raychaudhuri S, Hayer-Hartl M, et al. Protein folding in the cytoplasm and the heat shock response. Cold Spring Harb Perspect Biol. 2010;2:a004390.
- Mayer MP, Bukau B. Hsp70 chaperones: cellular functions and molecular mechanism. Cell Mol Life Sci. 2005;62:670–684.
- Hottiger T, De Virgilio C, Bell W, et al. 70-kilodalton heat-shock proteins of the SSA subfamily negatively modulate heat-shock-induced accumulation of trehalose and promote recovery from heat stress in the yeast, Saccharomyces cerevisiae. Eur J Biochem. 1992;210:125–132.
- Jones G, Song Y, Chung S, et al. Propagation of Saccharomyces cerevisiae [PSI+] prion is impaired by factors that regulate Hsp70 substrate binding. Mol Cell Biol. 2004;24:3928–3937.
- Jones GW, Masison DC. Saccharomyces cerevisiae Hsp70 mutations affect [PSI+] prion propagation and cell growth differently and implicate Hsp40 and tetratricopeptide repeat cochaperones in impairment of [PSI+]. Genetics. 2003;163:495–506.
- Xu L, Gong W, Cusack SA, et al. The beta6/beta7 region of the Hsp70 substrate-binding domain mediates heat-shock response and prion propagation. Cell Mol Life Sci. 2017;75(8):1445–1459.
- Loovers HM, Guinan E, Jones GW. Importance of the Hsp70 ATPase domain in yeast prion propagation. Genetics. 2007;175:621–630.
- Newnam GP, Birchmore JL, Chernoff YO. Destabilization and recovery of a yeast prion after mild heat shock. J Mol Biol. 2011;408:432–448.
- Newnam GP, Wegrzyn RD, Lindquist SL, et al. Antagonistic interactions between yeast chaperones Hsp104 and Hsp70 in prion curing. Mol Cell Biol. 1999;19:1325–1333.
- Allen KD, Wegrzyn RD, Chernova TA, et al. Hsp70 chaperones as modulators of prion life cycle: novel effects of Ssa and Ssb on the Saccharomyces cerevisiae prion [PSI+]. Genetics. 2005;169:1227–1242.
- Sharma D, Martineau CN, Le Dall MT, et al. Function of SSA subfamily of Hsp70 within and across species varies widely in complementing Saccharomyces cerevisiae cell growth and prion propagation. PLoS One. 2009;4:e6644.
- Sharma D, Masison DC. Functionally redundant isoforms of a yeast Hsp70 chaperone subfamily have different antiprion effects. Genetics. 2008;179:1301–1311.
- Roberts BT, Moriyama H, Wickner RB. [URE3] prion propagation is abolished by a mutation of the primary cytosolic Hsp70 of budding yeast. Yeast. 2004;21:107–117.
- Schwimmer C, Masison DC. Antagonistic interactions between yeast [PSI(+)] and [URE3] prions and curing of [URE3] by Hsp70 protein chaperone Ssa1p but not by Ssa2p. Mol Cell Biol. 2002;22:3590–3598.
- Sharma D, Masison DC. Single methyl group determines prion propagation and protein degradation activities of yeast heat shock protein (Hsp)-70 chaperones Ssa1p and Ssa2p. Proc Natl Acad Sci U S A. 2011;108:13665–13670.
- Fitzpatrick DA, O’Brien J, Moran C, et al. Assessment of inactivating stop codon mutations in forty Saccharomyces cerevisiae strains: implications for [PSI] prion- mediated phenotypes. PLoS One. 2011;6:e28684.
- Kityk R, Vogel M, Schlecht R, et al. Pathways of allosteric regulation in Hsp70 chaperones. Nat Commun. 2015;6:8308.
- Mayer MP, Kityk R. Insights into the molecular mechanism of allostery in Hsp70s. Front Mol Biosci. 2015;2:58.
- Zhuravleva A, Gierasch LM. Substrate-binding domain conformational dynamics mediate Hsp70 allostery. Proc Natl Acad Sci U S A. 2015;112:E2865–E2873.
- Zuiderweg ER, Bertelsen EB, Rousaki A, et al. Allostery in the Hsp70 chaperone proteins. Top Curr Chem. 2013;328:99–153.
- Jung G, Jones G, Wegrzyn RD, et al. A role for cytosolic hsp70 in yeast [PSI(+)] prion propagation and [PSI(+)] as a cellular stress. Genetics. 2000;156:559–570.
- Needham PG, Masison DC. Prion-impairing mutations in Hsp70 chaperone Ssa1: effects on ATPase and chaperone activities. Arch Biochem Biophys. 2008;478:167–174.
- Xu L, Hasin N, Shen M, et al. Using steered molecular dynamics to predict and assess Hsp70 substrate-binding domain mutants that alter prion propagation. PLoS Comput Biol. 2013;9:e1002896.