ABSTRACT
One of the major medical challenges of the twenty-first century is the treatment of incurable and fatal neurodegenerative disorders caused by misfolded prion proteins. Since the discovery of these diseases a number of studies have been conducted to identify small molecules for their treatment, however to date no curative treatment is available. These studies can be highly expensive and time consuming, but more recent experimental approaches indicate a significant application for yeast prions in these studies. We therefore used yeast prions to optimize previous high-throughput methods for the cheaper, easier and more rapid screening of natural extracts. Through this approach we aimed to identify natural yeast-prion inhibitors that could be useful in the development of novel treatment strategies for neurodegenerative disorders. We screened 500 marine invertebrate extracts from temperate waters in Australia allowing the identification of yeast-prion inhibiting extracts. Through the bioassay-driven chemical investigation of an active Suberites sponge extract, a group of bromotyrosine derivatives were identified as potent yeast-prion inhibitors. This study outlines the importance of natural products and yeast prions as a first-stage screen for the identification of new chemically diverse and bioactive compounds.
Introduction
Prion diseases are fatal, neurodegenerative disorders caused by the smallest infectious particle detected to date, transmissible misfolded proteins. In humans these fatal diseases are most commonly associated with the pathogenic human prion protein (PrPSc). PrPSc is a misfolded protein isoform that incorporates a large percentage of beta sheet structure. This causes the formation of amyloid-like plaques which build up and cause cell damage [Citation1]. Prions diseases include Creutzfeldt-Jakob disease (CJD) in humans, as well as scrapie and bovine spongiform encephalopathy in animals [Citation2]. An outbreak of CJD in the United Kingdom (UK) in the 1990s led to significant causative and therapeutic investigation that aimed to discover risk factors and treatments for the disorder. The consumption of tainted meat was shown to be a source of infection and this led to widespread concern about the contraction of the disease [Citation3]. To date however, no permanent treatments or therapeutic options have been discovered for these fatal diseases [Citation4]. Furthermore as our understanding of prion mechanisms has expanded, it has become evident that other chronic neurodegenerative diseases such as Alzheimer’s, Parkinson’s and Motor Neurone Disease may also have prion-like mechanisms [Citation5,Citation6]. Many factors have contributed to the slow progress in the discovery of new therapeutics, including the nature of the infectious agent, the mechanism of prion-mediated toxicity, and late diagnosis [Citation7].
The screening of large chemical libraries is considered an essential strategy for the discovery of anti-prion therapeutics. To date however, natural products have not been included in any anti-prion screening strategy. Natural products are compounds produced by organisms through secondary metabolic processes and are used in defense, competition and communication [Citation8]. Natural products have been shown to be a rich source of drugs, drug leads and probes to understand cellular and subcellular processes. These compounds are typically identified from their natural sources using screening-based isolation [Citation9]. However, there are limited assays available for the identification of natural products with anti-prion activity.
The majority of screening assays that are available to discover anti-prion compounds are based on animal models and mammalian cell lines. These assays, however, not only involve costly equipment and processes but are also time consuming [Citation10]. As a consequence these expensive assays have usually been limited to the evaluation of pure compounds and synthetic drugs. In 1994 prion-like proteins were discovered in the yeast Saccharomyces cerevisiae and shown to possess similar amyloid-like structures and mechanisms to mammalian prions [Citation11]. Bach et al. (2003) demonstrated that prions in the yeast S. cerevisiae could be used for screening large libraries of compounds to identify novel prion inhibitors. They constructed two yeast strains for this purpose by adding a biomarker (ADE1 and 2 genes) that can be used to identify the presence or absence of prions [Citation12]. These two yeast prions, [PSI+] and [URE3] can be detected using these ADE1 and 2 biomarkers. These two yeast prions have now been widely used to elucidate prion mechanisms and have been shown to be a good model for mammalian prions. The application of yeast based anti-prion assays can considerably reduce the cost and time involved in screening compound libraries compared to mammalian live-animal and animal cell line based anti-prion assays [Citation13]. As a consequence, yeast-based anti-prion assays can facilitate and significantly enhance the screening of larger libraries of compounds for anti-prion activity.
The [PSI+] and [URE3] yeast strains are characterized by white colored colonies when the Sup35 and Ure2 proteins, respectively are in their prion form. When the Sup35 and Ure2 proteins are in their normal, non-infectious form ([psi−] and [ure-o]) it results in red colored colonies. The Sup35 protein is an important factor for reading stop codons during translation termination. The ade1-14 mutation introduces a premature stop codon in the ADE1 gene. In [PSI+] cells, where the Sup35p is in its infectious prion form it aggregates and does not perform its biological role in the reading of stop codons. As such during translation of the modified ade1-14 mRNA there is an increased read-through of the premature stop codon leading to the translation of full length Ade1p and white colonies [Citation14]. In [psi−] cells, where Sup35p is in its normal (non-prion) soluble form, translation is terminated at the premature stop codon causing the formation of the truncated and inactive Ade1p and the build-up of the red-colored compound poly(ribosylaminoimidazole) (PRAI) in these cells, derived from an adenine biosynthetic precursor [Citation15].
Ure2p is a transcriptional repressor important for the regulation of nitrogen metabolism [Citation11,Citation16]. The DAL5 gene important for metabolism of non-preferred nitrogen sources is not expressed under conditions of good nitrogen availability when the Ure2p is in its functional soluble conformation while the DAL5 gene is transcribed when the Ure2p is in its prion amyloid and inactive form [17,Citation18]. In the SB34 yeast strain the DAL5 gene has been replaced with the ADE2 gene (as the sole copy of ADE2) leading to the build-up of the same red-colored compound when the Ure2p is in its normal form and represses transcription of the ADE2 gene.
The previous yeast-based anti-prion screening method described by Bach et al. (2003) was based on a disk-diffusion methodology. The disk-diffusion method was carried out by placing sterile disks onto agar plates inoculated with either the [PSI+] or [URE3] yeast strains. Compounds were then pipetted onto the disks and allowed to diffuse into the solid media. Active compounds were detected by a color change halo in the yeast surrounding the disks [Citation19]. This disk-diffusion method has been used successfully to identify novel prion inhibitors [Citation12]. It has a number of benefits including its simple set up and its ability to identify prion inhibitors even when the compound shows toxicity. For example a gradient of concentration from the center of the disk can lead to sub lethal concentrations showing prion inhibitory activity and this can be observed in a single assay. However, the assay also suffers from some limitations, including low throughput and limited quantitative analysis of compounds. Furthermore, compounds with limited agar-permeability may not be detected using this methodology.
To address these limitations, we modified the screening methodology described above to develop an economical, high-throughput and rapid yeast-based liquid broth culture assay for the identification of anti-prion compounds. These modifications were developed so that larger libraries of compounds could be screened quickly. Importantly, this also made possible the efficient screening of natural product extracts that may contain complex mixtures of compounds with different physicochemical properties. The newly developed assays use the same genetically-modified [PSI+] or [URE3] yeast strains developed by Bach et al. (2003). To validate the developed method, a library of marine invertebrate extracts was screened and anti-prion natural products were isolated and identified.
Results
Optimization of the anti-prion assay for use with natural products and crude extracts
It was hypothesized that the use of a liquid-phase micro-culture assay for screening would address the limitations of the previous solid-phase disk diffusion assay. Therefore the solid-phase assay was converted into an optimized liquid-phase micro-culture assay for both yeast strains. This was achieved with three objectives. The first objective was to develop a high-throughput screen that would provide quantitative bioactivity data. The second objective was to adopt a liquid-phase that could be used successfully and reproducibly in a micro-well format to screen for compounds with anti-prion activity. The third objective was to validate the assay and create a baseline for low to high activity by using compounds previously identified with anti-prion activity. The latter allowed the choice of optimum concentrations of marine natural product extracts for use in the screen. Once the screen was optimized, a collection of 500 extracts derived from marine invertebrates was screened (). The optimized screen was successful in that it resulted in the identification of 3 new anti-prion compounds.
Figure 1. A schematic diagram giving an overview of the large-scale screen (from left to right). A liquid-phase micro-culture assay is performed on each sample in a library of marine extracts and the color change of the yeast cultures from white to red indicates anti-prion activity. The marine invertebrate containing the compounds with anti-prion activity, the sponge Suberea ianthelliformis (pictured), underwent chemical analysis to identify the anti-prion compounds.
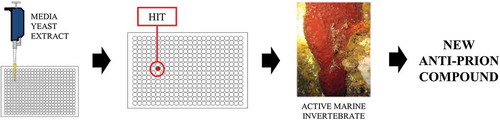
Use of PRAI as an indicator of prion curing
An important consideration when developing a high-throughput screen was the efficient detection and quantitation of curing. For this purpose the production of the colored PRAI was used. Using the reported solid-phase disk-diffusion assay method it is difficult to detect low levels of PRAI (e.g. in partially-cured colonies) and as a consequence compounds need to be tested at higher doses to detect anti-prion activity. Use of higher doses when screening risks compound toxicity that may confound results. Furthermore, compounds with low anti-prion activity could be missed.
The spectrophotometric properties of PRAI have been reported previously. Yeast cell lysates containing PRAI show a maximum fluorescence emission at 580 nm when excited at 488 nm [Citation20]. However, analysis of liquid cultures of intact yeast cells using these parameters was ineffective. This is most likely due to the background fluorescence of the growth medium. To overcome this issue of background fluorescence from the medium, fluorescence emission at 620 nm using excitation at 544 nm was used. This proved to be more effective as cultures of prion-infected cells showed minimal fluorescence with these excitation and emission wavelengths. Yeast cell lysates containing PRAI show UV-Vis absorption maxima at 490 nm and 540 nm [Citation15]. However, measurement of absorbance at 490 nm in liquid cultures was not possible due to background absorbance from the growth medium. Absorbance at 540 nm could be successfully used to detect the presence of PRAI since very low absorbance by prion-infected yeast cultures was detected at this wavelength.
Based on the spectrophotometric properties of PRAI, two different methods for the detection of PRAI in yeast cultures were developed. One method of detection used fluorescence (ex 544 nm/em 620 nm) and the other used absorbance (540 nm). To validate these methods, 24 replicates of 100% [PSI+] (infected) yeast cultures, 100% [psi−] (cured) yeast cultures and a mixture of these cultures (50% [PSI+]/50% [psi−]) were analyzed in 96- and 384-well plates (). A linear relationship between the color intensity based on the spectrophotometric measurement and percentage of [psi−] cells was obtained (R2 of 0.97 for fluorescence and 0.99 for absorbance).
Figure 2. A comparison of the accuracy and precision of the three methods of quantitating PRAI a. absorbance, b. fluorescence and c. CID. d. the scanned image of the micro-titer plate. The top four wells are 100% [psi−] cultures, the middle four wells are 50% [psi−]/50% [PSI+] cultures and the bottom four wells are 100% [PSI+] cultures (see the experimental section for [psi−] control cultures).
![Figure 2. A comparison of the accuracy and precision of the three methods of quantitating PRAI a. absorbance, b. fluorescence and c. CID. d. the scanned image of the micro-titer plate. The top four wells are 100% [psi−] cultures, the middle four wells are 50% [psi−]/50% [PSI+] cultures and the bottom four wells are 100% [PSI+] cultures (see the experimental section for [psi−] control cultures).](/cms/asset/3d1d62d1-0014-4c8e-ab0d-52c6ed8b270e/kprn_a_1513315_f0002_oc.jpg)
For screens that must be performed in physical containment facilities, access to dedicated equipment for screening can substantially add to the total costs. A more economical alternative detection method that does not require expensive equipment is therefore advantageous. The use of a domestic flatbed scanner with computer-imaging densitometry (CID) provides a more economical alternative to the use of plate-based fluorescence and absorbance scanners. This approach has been used effectively in the past for assays that require the measurement of colored indicators on thin films [Citation21]. Using the same test described above, 96- and 384- well plates containing 100% [PSI+] yeast cultures, 100% [psi−] yeast cultures and mixture of the two cultures (see above) were scanned on the flatbed scanner (CanoScan LiDE 220). The images obtained were analyzed using ImageJ software. The image was converted to grayscale using the green color filter and the grayscale density of each well was measured (). As was the case with the spectrophotometric methods, a linear relationship between the grayscale density and percentage of [psi−] cells was observed (R2 of 0.96).
Development of a liquid-phase micro-culture screen format
Because the readout from the prion-curing assay is either colorimetric or spectrophotometric (see above) a high cell density at stationary phase in the miniaturized assay format is required to detect curing of the yeast prion (as assessed by the red color intensity). A suitable growth medium for this assay therefore needs to support a high rate of growth of the yeast while at the same time maximizing the difference in PRAI concentration between cured and prion-infected cultures for sensitive detection of bioactives. Two types of media were tested, SD (minimal) and YPD (rich) medium. A significantly higher cell density at stationary phase was obtained using the YPD medium (>40 million cells per mL) compared to the SD medium (<2 million cells per mL). This result led to the YPD medium being selected for further optimization of the assay. The cell density of cultures was quantified using optical density measurements at 600 nm (OD600).
Neither STRg6 nor SB34 yeast strains synthesize adenine when cured of their respective prions, [PSI+] and [URE3]. Because of their ade1 and ade2 mutations respectively, they instead produce an adenine precursor compound that forms the red-colored PRAI. The concentration of adenine in the medium directly influences the rate of growth and the intensity of red color of the yeast colonies. Too much adenine in the medium leads to no PRAI synthesis by the yeast because the adenine biosynthesis pathway is down-regulated; too low a concentration of adenine leads to poor growth of the cured yeast and issues with the PRAI detection. In YPD medium adenine is limited by the concentration of yeast extract, and in previously reported prion-curing assays it was noted that significant variability of results is associated with changes in the type and concentration of yeast extract [Citation19]. We found that in liquid-phase micro-cultures the use of two different types of yeast extract (Oxoid yeast extract and BD Difco Bacto yeast extract) did not significantly influence the results obtained. However, the concentration of yeast extract in the growth medium was found to have a significant effect on the red color intensity of prion-cured yeast cultures. The concentration of yeast extract in the growth medium that was found to produce the greatest cell density at stationary phase without visibly affecting the intensity of the color change upon prion curing (cured using Guanidine hydrochloride) was between 0.25–1% w/v. The intensity of the color developed by the yeast cells upon prion-curing in batches of YPD containing this range of yeast extract concentrations was examined. Prion-infected and cured STRg6 and SB34 yeast strains were grown in liquid-phase micro-cultures and the difference in color intensity was measured. The results indicated that the greatest difference in color intensity between the prion-cured and infected strains was observed when the growth media contained 0.5% w/v yeast extract ()).
Figure 3. The optimization of the concentration of yeast extract in YPD medium and the yeast cell density of inoculates. a. the color intensity of prion-cured and infected [PSI+] cultures with different concentrations of yeast extract in the YPD medium. b. color intensity of micro-titer plate wells after cultures reached stationary phase relative to cell density at inoculation. Color intensity in both graphs is shown relative to the [PSI+] and [psi−] control cultures in graph a (see experimental section) and was measured using absorbance at 540 nm.
![Figure 3. The optimization of the concentration of yeast extract in YPD medium and the yeast cell density of inoculates. a. the color intensity of prion-cured and infected [PSI+] cultures with different concentrations of yeast extract in the YPD medium. b. color intensity of micro-titer plate wells after cultures reached stationary phase relative to cell density at inoculation. Color intensity in both graphs is shown relative to the [PSI+] and [psi−] control cultures in graph a (see experimental section) and was measured using absorbance at 540 nm.](/cms/asset/2445b7f6-6364-4979-8d89-7f0fae0e65cf/kprn_a_1513315_f0003_b.gif)
Treatment of prion-infected yeast with anti-prion compounds during early stages of exponential growth phase and lower cell density at inoculation will increase the number of generations of yeast cells treated before the culture reaches stationary phase. This would result in more sensitive detection of prion-curing (based on color intensity) from compounds that not only directly cure prions but ones that indirectly cure prions through other cellular processes. However, inoculation of cultures using a greater number of cells as inoculum has the advantage of shorter incubation times to reach stationary phase. To determine the optimal initial cell density at inoculation, a series of experiments were performed in which cultures were inoculated at different cell densities (2x104, 1x105, 5x105, 1x106, 2.5x106, 5x106, 1x107, 2×107 cells/mL) and then cured of prions using GuHCl ()). The most intense red color developed was in cured yeast cultures that had been inoculated at an initial cell density of 1×105 cells/mL or less. The incubation period depended on the growth rate of the yeast strain but the required cell density (>40 million cells/mL) was typically achieved after 48 hours of incubation at 24°C. Stationary phase entry is obligatory for the accumulation of PRAI [Citation19]. Storage of the yeast cultures for 48 hours at 2 – 4°C facilitated rapid color development in cultures that had been cured of prions.
Validation of the screen using known anti-prion compounds
To validate the screen, the use of the optimized methods with previously identified anti-prion compounds is important. Validation of the assay is dependent on these compounds exhibiting anti-prion activity with a clear dose-response relationship. In addition, this experiment allows the development of a baseline of activity against which new anti-prion compounds can be compared.
DMSO was selected as the carrier solvent for extracts and purified compounds due to its ability to dissolve a wide range of natural products, its miscibility with the yeast growth media and its low toxicity for yeast. Toxicity testing indicated that both yeast strains tolerate up to 4% (w/v) DMSO ()).
Figure 4. Experimental results for the validation of the screen with known anti-prion compounds. a. the toxicity of DMSO for the primary STRg6 [PSI+] yeast cells. Inhibition was measured through colony counting and was relative to a 0% (inoculated with no DMSO) and 100% (no cell inoculation) growth inhibition. b. percentage curing by guanabenz as a function of the concentration of GuHCl. Each curve represents the addition of a different sub-effective concentration of GuHCl to the growth medium. The color intensity was measured using CID and was relative to controls ([PSI+] had addition of DMSO and [psi−] had addition of 2mM GuHCl).
![Figure 4. Experimental results for the validation of the screen with known anti-prion compounds. a. the toxicity of DMSO for the primary STRg6 [PSI+] yeast cells. Inhibition was measured through colony counting and was relative to a 0% (inoculated with no DMSO) and 100% (no cell inoculation) growth inhibition. b. percentage curing by guanabenz as a function of the concentration of GuHCl. Each curve represents the addition of a different sub-effective concentration of GuHCl to the growth medium. The color intensity was measured using CID and was relative to controls ([PSI+] had addition of DMSO and [psi−] had addition of 2mM GuHCl).](/cms/asset/28046b7e-044b-48fd-8aba-6a6cb4dbb0f9/kprn_a_1513315_f0004_b.gif)
Guanidine hydrochloride (GuHCl) is known to inactivate the prion chaperone Hsp104p, a heat shock protein that is essential for the propagation of most yeast prions [Citation17]. The EC50 for GuHCl was shown to be 879 µM using the [PSI+] liquid-phase culture assay. It has also been reported that the addition of GuHCl to assay media greatly enhances the detection of anti-prion activity of other compounds [Citation19]. To validate this observation under liquid culture assay conditions, guanabenz, a compound previously shown to cure the [PSI+] yeast prion [Citation22], was tested with increasing (sub-effective) concentrations of GuHCl. This showed that addition of GuHCl to the growth medium increased the sensitivity of the assay and the detection of the anti-prion activity of guanabenz ()). Addition of GuHCl to the assay media to 250 µM final concentration produced optimal results.
With the GuHCl concentration optimized, the assay was then used to test guanabenz and two additional known anti-prion compounds, quinacrine and chlorpromazine. The EC50s for guanabenz, quinacrine and chlorpromazine were 25 µM, 215 μM, and >400 μM, respectively. The results obtained here show a similar trend to that reported previously. Guanabenz was reported to possess potent anti-prion activity, while quinacrine and chlorpromazine were both reported to be weakly active in the disk-diffusion assay [Citation22].
The optimized assay was also validated using the [URE3]-infected yeast strain and the same approach described above. The EC50 of GuHCl for curing of the [URE3] prion was found to be 3.9 mM and similarly to what was observed for the [PSI+] curing assays, addition of GuHCl to the assay growth medium greatly increased the sensitivity of detection of anti-prion activity. The addition of 2 mM GuHCl to the assay medium produced the optimal results. Guanabenz, quinacrine and chlorpromazine were screened for their ability to cure the [URE3] prion (addition of 2 mM GuHCl). It was found that a higher concentration of active compounds was required to observe activity against the [URE3] prion (relative to the [PSI+] prion). For this reason, EC50 values were undefined (>400 μM) due to toxicity from the higher concentration of compounds. While the EC50 values could not be calculated, low level curing activity was observed for all the compounds tested. For screening the [PSI+] prion was used for quantitative assays to compare bioactivity of compounds and the [URE3] prion was used for qualitative confirmatory assays.
Screening of natural product extracts for anti-prion activity
The activity of the known anti-prion compounds in the liquid-phase micro-culture assay provided a benchmark for selection of the concentration at which to screen crude extracts. Our aim was to find pure compounds with EC50s less than 100 µM and since natural products are generally present in crude extracts at concentrations below 1% w/w the crude extracts were screened at a relatively high concentration (4 mg/mL).
Extracts of 500 marine invertebrates were screened in triplicate in the liquid-phase micro-culture assay using the optimized medium and GuHCl (250 µM). The percentage color intensity (relative to controls) for the crude extracts tested was found to have a high standard deviation (SD = 43%). This was due to a number of the extracts showing toxicity as determined by lower than expected OD600 readings (indicating lower yeast cell densities in these wells). Some of the wells with low cell densities also contained PRAI, indicating prion curing. This meant that a method to quantify prion-curing activity in wells with low yeast cell densities was needed. To determine if a linear relationship exists between yeast cell density and PRAI concentration a set of serial dilutions of [psi−] and [PSI+] cultures were analyzed to compare the yeast cell density (OD600) and the color intensity (PRAI). This indicated a linear relationship for both cultures (R2 of 0.99 for [psi−] and [PSI+]). This allowed the correction of the controls for high or low yeast cell densities in the micro-culture so that the corrected color intensity of assays could be calculated. The use of this correction resulted in the percent color intensity values of the assay results having a lower SD of 15.6%. The initial screen therefore identified 25 extracts with anti-prion activity >2SDs above the mean (corresponding to approx. >40% color intensity relative to the controls). However, this initial screen also identified 35 extracts that significantly inhibited the growth of the yeast (corresponding to >70% growth inhibition).
The 60 bioactive extracts (highly toxic or anti-prion) were rescreened at six doses (10, 4.0, 1.0, 0.4, 0.1, 0.04 mg/mL) to determine the potency of the extract (if active) or if the extract was a false positive. The regrowth of the cured cells on solid media is also important for identifying false positives. Any compounds that simply influence the expression of the adenine reporter gene would give negative results upon regrowth. Therefore, cured yeast cells from the active wells were regrown on solid media to determine if the colonies remained red (cured). This resulted in 13 extracts being confirmed as anti-prion as they cure the [PSI+] prion. Finally, these active extracts were tested for their ability to cure the [URE3] prion at a single concentration. The single concentration selected was dependent on the toxicity of the extracts (between 10 – 0.01 mg/mL). Of the 500 extracts screened, six were found to exhibit detectable anti-prion activity against both yeast prions (see supplementary file for full screening data).
Isolation of active compounds from active extracts
Among the extracts shown to possess prion-curing activity two were from the sponge species Suberea ianthelliformis collected from oceanic waters off Cook Is, NSW, Australia. A large quantity (20.15 g) of one of these samples (ACENV0134) was exhaustively extracted with methanol, yielding a deep red gum (0.80 g). The extract was separated using reverse-phase chromatography yielding a number of fractions. Subsequently, five pure compounds which had been found previously in Verongid sponges were isolated. Analysis of data obtained from extensive 1D and 2D nuclear magnetic resonance spectroscopy (NMR) experiments and mass spectrometry (MS) revealed that these compounds were aplysamine-1 (1), aplysamine-2 (2), purealidine-Q (3), 3,5-dibromoverongiaquinol (4) and 3,5-dibromoverongiaquinol dimethyl ketal (5) (see supplementary file for chemical data).
Three of the isolated compounds exhibited potent bioactivity against the [PSI+] prion (). Aplysamine-1 (1), aplysamine-2 (2), and purealidine-Q (3) showed EC50 values for curing of the [PSI+] prion (with 0.25 mM GuHCl) of 102.2 μM, 4.15 μM and 2.35 μM respectively. The three active compounds were found to cure the [URE3] prion thereby confirming the anti-prion activity of the compounds. Further evaluation showed that the activity of compounds 1–3 was synergistic with GuHCl. When the assay was conducted without GuHCl no prion inhibitory activity was observed from 1–3 up to a concentration of 0.5 mM (although 2 and 3 were toxic to the cells above 64 µM) and when the concentration of GuHCl was increased to 0.5 mM all three compounds showed 100% prion inhibitory activity at the lowest dose tested (0.5 µM) (see supplementary file). This result is in agreement with that previously reported for guanabenz, quinacrine and chlorpromazine [Citation12,Citation22], indicating that 1–3 most likely do not target Hsp104p and that they target another pathway that may be conserved between yeast and mammalian cells. The two other bromotyrosine derivatives 3,5-dibromoverongiaquinol (4) and 3,5-dibromoverongiaquinol dimethyl ketal (5) were found to be inactive against both the [PSI+] and [URE3] prions.
Figure 5. Chemical structures of the natural products identified in this study and respective screening results. a. Flatbed scanned image of the wells of the micro-titer plate. This displays the change in color of the STRg6 yeast cultures when grown in the presence of the three compounds (one replicate). b. Dose-response curves for the anti-prion activity against [PSI+] of the three active compounds. The dotted black line displays the anti-prion activity of guanabenz in the assay, indicating the baseline for potent anti-prion activity. The color intensity was relative to the controls (200 μM guanabenz for the positive) and was measured using CID.
![Figure 5. Chemical structures of the natural products identified in this study and respective screening results. a. Flatbed scanned image of the wells of the micro-titer plate. This displays the change in color of the STRg6 yeast cultures when grown in the presence of the three compounds (one replicate). b. Dose-response curves for the anti-prion activity against [PSI+] of the three active compounds. The dotted black line displays the anti-prion activity of guanabenz in the assay, indicating the baseline for potent anti-prion activity. The color intensity was relative to the controls (200 μM guanabenz for the positive) and was measured using CID.](/cms/asset/e6fc1244-517f-4b79-b0f6-910f14ffbf10/kprn_a_1513315_f0005_oc.jpg)
In addition to the observed anti-prion activity of aplysamine-2 (2) and purealidine-Q (3), both compounds inhibited the growth of yeast at higher concentrations (≥100 mg/L). However, aplysamine-1 (1), 3,5-dibromoverongiaquinol (4) and 3,5-dibromoverongiaquinol dimethyl ketal (5) did not significantly inhibit the growth of the yeast at the concentrations tested.
Discussion
The yeast-based anti-prion screen allows for the identification of bioactive natural products with lower risks and costs than equivalent screens using mammalian prions [Citation13]. Here we have shown that the use of a liquid-phase micro-culture assay has significant advantages when compared to solid-phase disk-diffusion methods employed in previous yeast-based anti-prion screens. While the previous disk-diffusion methodology has many benefits, it is labor-intensive, provides only qualitative data and is dependent for its success on the efficient diffusion of compounds through agar. The limitations of this methodology limit the ability to detect low-level curing and to compare the potency of bioactive compounds [Citation19]. The use of liquid-phase micro-cultures in micro-titer plates significantly reduces the labor involved and by providing quantitative data allows the comparison of the bioactivity of compounds. These advantages allow the optimized screening strategy developed in this study to be used to screen compounds from diverse sources, including biota extracts containing complex mixtures of natural products. This is a significant advance because natural products present a significantly greater chemical diversity than synthetic drug and compound libraries and are therefore a potentially richer source of bioactive molecules [Citation23]. Furthermore, the quantitative nature of the optimized screen enables its use in structure-activity relationship and drug-design studies.
The use of an optimized yeast-based anti-prion assay with increased throughput and quantitation that is compatible with the screening of natural extracts led to the identification of a series of marine natural products with anti-prion activity. All active compounds identified in the extract of the sponge S. ianthelliformis contained an ethylaminodibromophenyl (EADP) moiety and were highly potent. In previous studies, guanabenz was shown to be the most active compound [Citation12,Citation22]. In our assay guanabenz also exhibited the most potent activity of the previously identified anti-prion compounds. However, we now have found that aplysamine-2 (2) and purealidine-Q (3) are significantly more potent than guanabenz.
Aplysamine-1 (1) and aplysamine-2 (2) were first isolated in 1989 from the Verongidae sponge, Aplysina sp [Citation24]. Purealidine-Q (3) was first isolated in 1994 from an Okinawan sponge, Psammaplysilla purea, from the same family [Citation25]. The EADP structure has been found in a number of compounds including the moloka’iamines [Citation26], ceratinamines [Citation27], purpurealidines [Citation28] and purealidines [Citation29], that have been isolated from the Verongidae sponges. This compound class has been found to possess a wide range of bioactivities, including anti-fouling [Citation30], anti-microbial [Citation31], acetylcholinesterase (AChE) inhibition [Citation32], apolipoprotein E (ApoE) modulating activity [Citation33], anti-HIV [Citation34] and inhibition of the histamine subtype 3 (H3) receptor [Citation35]. It is interesting to note that the H3 receptor, ApoE and AChE have all been identified as potential targets for the treatment of various neurodegenerative diseases [Citation36,Citation37].
The inability of 3,5-dibromoverongiaquinol (4) and 3,5-dibromoverongiaquinol dimethyl ketal (5) to cure yeast of prions provides the first indication of the structural features of these compounds that may be important for anti-prion activity. The alkyl amine chain is present in all three active compounds, but is absent in the inactive compounds. This structural feature may therefore be necessary for anti-prion activity. Aplysamine-2 and purealidine-Q have lipophilic moieties amide-linked to the EAPD moiety instead of the amine. The presence of these lipophilic groups correlates with an order of magnitude increase in potency. The increased logP of 2 and 3 may make them more cell-permeable, resulting in more potent anti-prion activity compared to 1 since the compounds need to pass through the cell membrane of the yeast cells to inhibit or cure the prions. Increased membrane-permeability may also explain why 2 and 3 exhibit increased toxicity compared to 1. A number of anti-prion compounds identified in previous in vitro cell-free assays have not been developed further because they show poor blood brain barrier permeability and this was primarily due to both their logP value and large molecular size [Citation38]. Therefore, since the EADP-compounds described here must cross the yeast cell membrane to exhibit anti-prion activity they may be useful leads for the future development of anti-prion drugs. A large number of related EADP-containing compounds have been reported from marine invertebrates and it would be fruitful to screen these related EADP-containing compounds for anti-prion activity. The EADP structure class may serve as a useful lead for the future development and design of novel and improved anti-prion therapeutics.
Additionally, the fact that the compounds identified here are probably not active against the yeast prion chaperone Hsp104p targeted by GuHCl suggests that they may have other targets potentially including target proteins and pathways that are conserved in mammals. Future work should be directed towards the investigation of the activity of the compounds against mammalian prions and characterization of their mode of action. Subsequently, the activity of these compounds in models of various other neurodegenerative diseases that have recently been attributed to amyloids could be explored. Finally, we will use the optimized screen to investigate larger libraries of natural extracts from additional sources.
In conclusion the results of this study support the view that natural products, and in particular those derived from invertebrates, are a rich but until now untapped and therefore potentially important source of anti-prion compounds. Recent research findings have implicated prions (or at least prion-like mechanisms) in a number of chronic neurodegenerative disorders with high and increasing incidence including Alzheimer’s disease [Citation39] and Multiple systems atrophy [Citation40]. Therefore, compounds such as those identified in this study (or compounds based on them) that would be predicted to cross the blood-brain barrier and deliver anti-prion activity to the brain are of potentially great significance.
Experimental methods
General biological procedures
Analytical grade compounds, guanidine hydrochloride (GuHCl), guanabenz, quinacrine and chlorpromazine were purchased from Sigma (Castle Hill, NSW). A BMG Labtech FLUO star omega microplate fluorimeter was used to measure fluorescence and absorbance in micro-well plates and a Canon CanoScan LiDE 220 flatbed color scanner was used for image capture. The color images were analyzed using ImageJ 1.51j8 computer software (freely available from http://imagej.nih.gov/ij/download.html). S. cerevisiae was grown in ½YPD growth media prepared by dissolving D-glucose (2%), BD (North Ryde, NSW) yeast extract (0.5%, variations in some experiments) and Oxoid bacteriological peptone (2%) in sterile milli-Q filtered water. Solid media were prepared with the same ingredients with the addition of BD Bacto agar 2% (w/v). The growth media were sterilized by autoclaving at 121°C for 20 minutes. Stock solutions (10 mM) of guanabenz, quinacrine and chlorpromazine were prepared in DMSO. A stock solution of GuHCl (300 mM) was prepared in sterile milli-Q filtered water.
Yeast prion strains
Both the [PSI+] and [URE3] prions of S. cerevisiae were screened [Citation12]. The significant differences in the structure and function of these yeast-prions provided an ideal platform for an examination of compounds active against molecular mechanisms common to diverse prions. Compounds active against only one strain may inhibit more specific pathways used by a particular prion, whereas, in previous studies compounds that were reported to cure both the [PSI+] and [URE3] prions were also found to be active against mammalian prions [Citation13]. The STRg6 strain contains the [PSI+] prion (MATa ade1-14 trp1-289 his3Δ200 ura3-52 leu2-3,112 erg6::TRP1 [PSI+]). The SB34 strain contains the [URE3] prion (MATa ade2-1 trp1-1 leu2-3,112 his3-11,15 ura2::HIS3 dal5::ADE2 [URE3]). The genotypes and modifications of the STRg6 and SB34 strains are explained in detail in Bach et al. (2006) [Citation19]. For each strain, the phenotypes associated with the presence of the normal form of the proteins Sup35p and Ure2p ([psi−] for STRg6 and [ure-o] for SB34) were established. This was done by the treatment of prion-infected strains with GuHCl during serial subculturing (each time picking red colonies on solid media for the next subculture).
Extract and compound screening
Marine invertebrates were collected from the northern NSW coast and freeze-dried for storage. The crude extracts for initial screening were obtained by exhaustively extracting each invertebrate (0.5 g) with methanol (MeOH). Each extract was then redissolved in DMSO (100 mg/mL). Black wall 384-well plates were used for the primary screens and this allowed 308 separate assays to be performed per plate. Assays in 384-well plates were carried out in a total volume of 50 μL containing growth medium with 0.25 μM GuHCl addition (46 μL), a culture of one of the S. cerevisiae yeast strains (2 μL) and DMSO containing a marine invertebrate extract (2 μL). Dilution gradients and other confirmatory assays were performed in 96-well plates allowing 60 separate assays to be performed per plate. Assays in 96-well plates were carried out with a total volume of 250 μL containing growth medium with 0.25 μM GuHCl addition (240 μL), culture of one of the S. cerevisiae yeast strains (5 μL) and DMSO containing a marine invertebrate extract (5 μL). To evaluate the synergistic effects of compounds with GuHCl the assay above was performed with differing concentrations of GuHCl (0 – 1 mM). Plates were incubated at 24°C for 48 hours and then transferred to 4°C for 48 hour before analysis. The outer wells of the microtiter plates were filled with sterile water to minimise evaporation of the assay media.
General chemical procedures
NMR spectra were recorded at 25°C on a Bruker Avance III 500 MHz spectrometer (BBFO Smartprobe, 5 mm 31P-109Ag) with solvent peak referenced to δH 2.49 and δC 39.5 in DMSO-d6. ESI-TOF data was recorded on an Agilent 6530-accurate mass Q-TOF LC/MS mass spectrometer. Alltech Davisil 30–70 μm 60 Å C18 silica was used to adsorb the sponge extract prior to HPLC separation. A Merck Hitachi L7100 pump and L7455 PDA detector connected to a Thermo Betasil C18 150 × 21.2 mm 5 μm 100 Å HPLC column were used for HPLC separations. All solvents used for chromatography and MS were HPLC grade and the H2O was Millipore Milli-Q PF filtered.
Extraction and isolation
The bioactive freeze-dried sample of the sponge Suberites ianthelliformis (20.15 g) was exhaustively extracted with MeOH to yield a crude extract (0.7973 g). The MeOH extract was adsorbed onto the C18 silica gel at a 1:1 ratio (0.8 g) and packed into the HPLC refillable cartridge (10 mm x 20 mm). The refillable cartridge was then connected in series with a Betasil C18 bonded silica HPLC column (21 mm x 150 mm). The columns were eluted with a gradient from 100% H2O containing 0.1% TFA to 100% MeOH containing 0.1% TFA over 60 min at a flow rate of 9 mL/min. The column was further eluted with 100% MeOH containing 0.1% TFA for 10 min. A total of 70 fractions were collected at one min intervals. Aliquots (10% from each fraction) from seven sequential fractions were combined yielding a total of ten fractions for anti-prion testing. The bioactivity of these combined fractions was evaluated after redissolving them in DMSO and the un-combined fractions were analyzed by 1H NMR. Based on 1D and 2D NMR analysis, pure and known compounds aplysamine-1 (1) (9.6 mg), aplysamine-2 (2) (8.0 mg), purealidine-Q (3) (5.8 mg), 3,5-dibromoverongiaquinol (4) (5.5 mg) and 3,5-dibromoverongiaquinol dimethyl ketal (5) (6.2 mg) were identified. These compounds were assayed (as above) to determine effective concentration at which 50% of the yeast cells are red coloured (EC50).
Supplemental Material
Download MS Word (2.4 MB)Acknowledgments
We thank Marc Blondel, Cecile Voisset and Flavie Soubigou from CNRS in Roscoff, France for providing the yeast strains used in the assay. We thank Joshua Hayton for extraction of the marine invertebrate library. LKJ was supported by an Australian Government Research Training Program Scholarship.
Disclosure statement
No potential conflict of interest was reported by the authors.
Supplementary material
Supplemental data for this article can be accessed here.
References
- Prusiner SB. Prions. Proc Natl Acad Sci USA. 1998;95:13363–13383.
- Prusiner SB. Molecular biology of prion diseases. Science. 1991;252:1515–1522.
- Collinge J, Sidle KC, Meads J, et al. Molecular analysis of prion strain variation and the aetiology of ‘new variant’ CJD. Nature. 1996;383:685–690.
- Seelig DM, Goodman PA, Skinner PJ. Potential approaches for heterologous prion protein treatment of prion diseases. Prion. 2016;10:18–24.
- Scheckel C, Aguzzi A. Prions, prionoids and protein misfolding disorders. Nat Rev Genet. 2018;19:405–418.
- Antony H, Wiegmans AP, Wei MQ, et al. Potential roles for prions and protein-only inheritance in cancer. Cancer Metastasis Rev. 2012;31:1–19.
- Imberdis T, Heeres JT, Yueh H, et al. Identification of anti-prion compounds using a novel cellular assay. J Biol Chem. 2016;291:26164–26176.
- Maplestone RA, Stone MJ, Williams DH. The evolutionary role of secondary metabolites – a review. Gene. 1992;115:151–157.
- Newman DJ, Cragg GM. Natural products as sources of new drugs from 1981 to 2014. J Nat Prod. 2016;79:629–661.
- Solassol J, Crozet C, Lehmann S. Prion propagation in cultured cells. Br Med Bull. 2003;66:87–97.
- Wickner RB. [URE3] as an altered Ure2 protein: evidence for a prion analog in Saccharomyces cerevisiae. Science. 1994;264:566–569.
- Bach S, Talarek N, Andrieu T, et al. Isolation of drugs active against mammalian prions using a yeast-based screening assay. Nat Biotechnol. 2003;21:1075–1081.
- Tribouillard D, Bach S, Gug F, et al. Using budding yeast to screen for anti-prion drugs. Biotechnol J. 2006;1:58–67.
- Wickner RB, Masison DC, Edskes HK. [PSI+] and [URE3] as yeast prions. Yeast. 1995;11:1671–1685.
- Smirnov M, Smirnov V, Budowsky E, et al. Red pigment of adenine-deficient yeast Saccharomyces cerevisiae. Biochem Biophys Res Commun. 1967;27:299–304.
- Shewmaker F, Mull L, Nakayashiki T, et al. Ure2p function is enhanced by its prion domain in Saccharomyces cerevisiae. Genetics. 2007;176:1557–1565.
- Liebman SW, Chernoff YO. Prions in yeast. Genetics. 2012;191:1041–1072.
- Coschigano PW, Magasanik B. The URE2 gene product of Saccharomyces cerevisiae plays an important role in the cellular response to the nitrogen source and has homology to glutathione s-transferases. Mol Cell Biol. 1991;11:822–832.
- Bach S, Tribouillard D, Talarek N, et al. A yeast-based assay to isolate drugs active against mammalian prions. Methods. 2006;39:72–77.
- Bruschi CV, Chuba PJ. Nonselective enrichment for yeast adenine mutants by flow cytometry. Cytometry. 1988;9:60–67.
- Teasdale PR, Hayward S, Davison W. In situ, high-resolution measurement of dissolved sulfide using diffusive gradients in thin films with computer-imaging densitometry. Anal Chem. 1999;71:2186–2191.
- Tribouillard-Tanvier D, Beringue V, Desban N, et al. Antihypertensive drug Guanabenz is active in vivo against both yeast and mammalian prions. PLoS One. 2008;3:e1981.
- Feher M, Schmidt JM. Property distributions: differences between drugs, natural products, and molecules from combinatorial chemistry. J Chem Inf Comput Sci. 2003;43:218–227.
- Xynas R, Capon R. Two new bromotyrosine-derived metabolites from an Australian marine sponge, Aplysina sp. Aust J Chem. 1989;42:1427–1433.
- Kobayashi J, Honma K, Sasaki T, et al. Purealidins J-R, new bromotyrosine alkaloids from the Okinawan marine sponge Psammaplysilla purea. Chem Pharm Bull. 1995;43:403–407.
- Hamann MT, Scheuer PJ, Kelly-Borges M. Biogenetically diverse, bioactive constituents of a sponge, order Verongida: bromotyramines and sesquiterpene-shikimate derived metabolites. J Org Chem. 1993;58:6565–6569.
- Tsukamoto S, Kato H, Hirota H, et al. Ceratinamine: an unprecedented antifouling cyanoformamide from the marine sponge Pseudoceratina purpurea. J Org Chem. 1996;61:2936–2937.
- Tilvi S, Rodrigues C, Naik CG, et al. New bromotyrosine alkaloids from the marine sponge Psammaplysilla purpurea. Tetrahedron. 2004;60:10207–10215.
- Ishibashi M, Tsuda M, Ohizumi Y, et al. Purealidin A, a new cytotoxic bromotyrosine-derived alkaloid from the Okinawan marine sponge Psammaplysilla purea. Experientia. 1991;47:299–300.
- Ortlepp S, Sjoegren M, Dahlstroem M, et al. Antifouling activity of bromotyrosine-derived sponge metabolites and synthetic analogues. Mar Biotechnol. 2007;9:776–785.
- Gotsbacher MP, Karuso P. New antimicrobial bromotyrosine analogues from the sponge Pseudoceratina purpurea and its predator Tylodina corticalis. Mar Drugs. 2015;13:1389–1409.
- Olatunji OJ, Ogundajo AL, Oladosu IA, et al. Non-competitive inhibition of acetylcholinesterase by bromotyrosine alkaloids. Nat Prod Commun. 2014;9:1559–1561.
- Tian L-W, Feng Y, Shimizu Y, et al. Aplysinellamides A–C, bromotyrosine-derived metabolites from an Australian Aplysinella sp. Marine Sponge J Nat Prod. 2014;77:1210–1214.
- Schoenfeld RC, Lumb J-P, Fantini J, et al. Total synthesis of mololipids: a new series of anti-HIV Moloka’iamine derivatives. Bioorg Med Chem Lett. 2000;10:2679–2681.
- Brogan JT, Stoops SL, Crews BC, et al. Total synthesis of (+)-7-bromotrypargine and unnatural analogues: biological evaluation uncovers activity at CNS targets of therapeutic relevance. ACS Chem Neurosci. 2011;2:633–639.
- Morisset S, Rouleau A, Ligneau X, et al. High constitutive activity of native H3 receptors regulates histamine neurons in brain. Nature. 2000;408:860–864.
- Torrent J, Vilchez-Acosta A, Muñoz-Torrero D, et al. Interaction of prion protein with acetylcholinesterase: potential pathobiological implications in prion diseases. Acta Neuropathol Commun. 2015;3:18.
- Doh-Ura K, Ishikawa K, Murakami-Kubo I, et al. Treatment of transmissible spongiform encephalopathy by intraventricular drug infusion in animal models. J Virol. 2004;78:4999–5006.
- Vishnevskaya AB, Kushnirov VV, Ter-Avanesyan MD. Neurodegenerative amyloidoses: yeast model. Mol Biol. 2007;41:308–315.
- Prusiner SB, Woerman AL, Mordes DA, et al. Evidence for α-synuclein prions causing multiple system atrophy in humans with parkinsonism. Proc Natl Acad Sci USA. 2015;112:E5308–E5317.