Abstract
The disproportional enlargement of the neocortex through evolution has been instrumental in the success of vertebrates, in particular mammals. The neocortex is a multilayered sheet of neurons generated from a simple proliferative neuroepithelium through a myriad of mechanisms with substantial evolutionary conservation. This developing neuroepithelium is populated by progenitors that can generate additional progenitors as well as post-mitotic neurons. Subtle alterations in the production of progenitors vs. differentiated cells during development can result in dramatic differences in neocortical size. This review article will examine how cadherin adhesion proteins, in particular α-catenin and N-cadherin, function in regulating the neural progenitor microenvironment, cell proliferation, and differentiation in cortical development.
Abbreviations
VZ | = | ventricular zone |
SNP | = | short neural precursor |
CBD | = | catenin binding domain |
JMD | = | juxtamembrane domain |
PI3K | = | phosphatidylinositol 3-kinase |
PTEN | = | phosphatase and tensin homolog |
GSK3β | = | glycogen synthase kinase 3β |
PCP | = | planar cell polarity |
SHH | = | sonic hedgehog |
Hh | = | Hedgehog |
CK1 | = | Casein kinase 1 |
APC | = | Adenomatous polyposis coli |
Introduction
The mammalian neocortex is a multilayered sheet of neurons generated from a proliferative neuroepithelium lining the lateral ventricles. This neuroepithelium, called the ventricular zone (VZ), is comprised of highly polarized neural progenitors that divide to generate additional progenitors and maturing neurons. Subtle alterations in the production of progenitors vs. differentiated cells during development can result in dramatic differences in neocortical size.Citation1,2 Recent studies have provided evidence that cadherin adhesion is important in maintaining the cytoarchitectural integrity of the VZ, and alterations in adhesion result in mis-regulation of cell signaling pathways regulating neural development.Citation3-6 The intact organization of the neuroepithelium appears essential for the normal balance of proliferation vs. differentiation, and widespread disruption of adhesion in the developing neuroepithlium leads to hyperactivation of signaling pathways that drive proliferation.Citation3-6 In contrast, reduction of adhesion in individual progenitors in an otherwise intact epithelium reduces canonical Wnt/β-catenin activity in the progenitors and leads to cell cycle exit and delamination from the VZ.Citation6-8 This review article will examine how cadherin cell-cell adhesion in the neural progenitor microenvironment regulates signaling pathways controlling proliferation and differentiation in cortical development.
Cortical neural progenitors are polarized neuroepithelial cells
The generation of neocortical neurons, or neurogenesis, in rodents begins shortly after the neural tube is closed and continues until just before birth.Citation9 Cortical projection neurons are generated from neural progenitors that develop from the pseudo-stratified neuroepithileum of the developing neocortex adjacent to the lateral ventricles termed the Ventricular Zone (VZ). Radial glial cells are highly polarized neural progenitors that possess a process attached to the apical (luminal) surface and a long process attached to the basal (cortical) surface that provides a physical structure to facilitate neuronal migration ().Citation10 Radial glial cells translocate their cell bodies to divide at the apical surface and can self-renew,Citation11-13 generate neurons,Citation14,15 as well as produce transient amplifying cells (termed basal or intermediate progenitors) that serve to produce later-generated neurons ().Citation16-18 There is another progenitor type that resides in the VZ of developing mouse brains, the short neural precursor (SNP). These apical progenitors also divide at the apical surface, can self-renew, and generate neurons ().Citation19 Unlike radial glia, they do not possess a long basal process and typically do not generate transient-amplifying basal progenitors.
Figure 1. Organization of the developing mouse neocortex. The two classes of neural precursor, radial glia and short neural precursors, both reside in the Ventricular Zone (VZ) with a process attached to the apical (luminal) surface adjacent to the developing lateral ventricle. The overt structural distinction between the 2 neural precursors is that radial glia possess a process attached to the basal (cortical) surface while short neural precursors do not. During mitosis the cell body migrates to the apical surface where it will divide to generate 2 daughter cells. In the case of radial glia, the daughter cells can be new radial glia cells, basal progenitors, or post-mitotic neurons. Basal progenitors are a transient type of progenitor that resides basal to the VZ in the Subventricular Zone (SVZ), and can also self-renew as well as generate post-mitotic neurons. Post-mitotic neurons migrate away from the VZ and/or SVZ along radial glia processes through the Intermediate Zone (IZ) to populate the developing Cortical Plate (CP) in an inside-out fashion, where the deepest cortical layers are generated first. The other apical precursor type, short neural precursors, can also self-renew or generate post-mitotic neurons, however they do not produce basal progenitors.
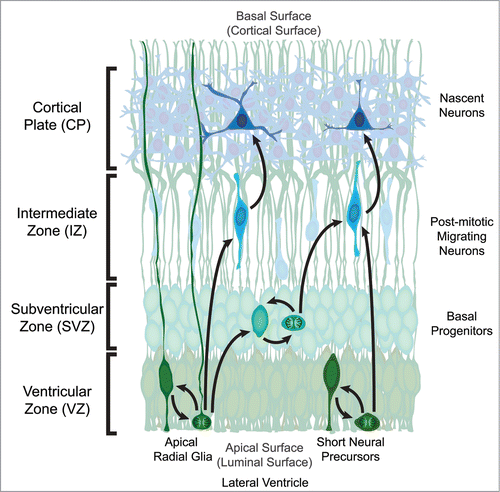
Through the course of neural development, a balance between self-sustaining proliferative divisions and neurogenic differentiation divisions must be appropriately maintained, and modest alterations in the fraction of daughter cells that exit or re-enter the cell cycle can result in dramatic changes in cortical size.Citation1,2 Dividing precursor cells in the VZ can yield daughter cells with the same fate (symmetric) or with differing fates (asymmetric).Citation20-22 The control of cell fate choices, cell proliferation and differentiation, is regulated by both cell-intrinsic and environmental influences on radial glial cells in the VZ.Citation23-25
Environmental influences have received less attention than cell intrinsic mechanisms, however recent evidence has demonstrated that cerebrospinal fluid can promote proliferation of radial glia.Citation26 Proliferative fate decisions are also regulated by a myriad of different intrinsic processes.Citation27,28 The orientation of cell division and asymmetric inheritance of cellular components can predict the behavior of daughter cells.Citation29-31 As in other tissues, in the neuroepithelium the daughter cell that inherits the older (mother) centrosome is preferentially retained in the VZ as a progenitor.Citation32 Inheritance of another component of the primary cilium, the apical ciliary membrane, has also been shown to help retain the recipient daughter cell in the VZ.Citation33
Asymmetric inheritance of adhesion machinery and polarity protein complexes can also influence cell fate decisions. Daughter cells with higher levels of Par3, a key component of the Par protein complex that regulates polarity, are preferentially retained as progenitors.Citation34 Cadherin adhesion also plays a critical role in establishing and maintaining apical-basal polarity in epithelia.Citation35,36 Evidence that adherens junctions could regulate asymmetric division in Drosophila neuroblastsCitation37 further supported the possibility that cadherin adhesion could impact cell behaviors in the developing cortical neuroepithelium. However, the critical role that cadherin adhesion served in maintaining epithelial architecture in cortical development complicated the interpretation of broad genetic loss-of-function methods with regard to the role of adhesion in neural cell fate determination.Citation4,5,38
Cadherins and catenins mediate adhesion and signaling
There are 4 different classes of cadherins: classical types 1 and 2, protocadherins, desmosomal, and atypical.Citation39 Classical cadherins interact with cadherin molecules in other cells (trans-interactions) as well as those nearby in the same cell (cis-interactions).Citation40,41 Once initial contact is made between adjacent cells expressing cadherin, the cadherins cluster into puncta along the point of contact, then strengthen to form concentrated plaques of cadherin complexes.Citation42 The intracellular domain of classic cadherins consists of a juxtamembrane domain (JMD) and catenin binding domain (CBD). The JMD binds p120-cateninCitation43 while the CBD binds β-catenin.Citation44,45 Additionally, the cadherin intracellular domain also binds a number of other proteins that regulate normal housekeeping and trafficking functions such as endocytosis and degradation.Citation46
β-catenin contains 12 characteristic imperfect sequence armadillo repeatsCitation47 that form a triple α-helix.Citation48 This portion of β-catenin contains the cadherin binding site,Citation49,50 while the N-terminal portion contains the α-catenin binding site.Citation51,52 β-catenin also serves as the defining downstream mediator of the canonical Wnt signaling pathway.Citation53 When Wnt signaling is activated, β-catenin degradation by an APC-containing “destruction complex” is inhibited,Citation54-56 allowing for β-catenin to accumulate, translocate to the nucleus, and in conjunction with TCF/LEF proteins activate gene transcription.Citation50,57-59
α-catenin serves as the link between the cadherin/catenin complex and the actin cytoskeleton, as it can bind both β-cateninCitation44 and filamentous actin.Citation60 When sufficient physical tension is present within the cell, a strong bond is formed between F-actin and the ternary α-catenin/β-catenin/cadherin structure.Citation61 This linkage is not static, but rather dynamic as F-actin rapidly dissociates from the ternary complex when the tension is substantially reduced.Citation61 The ternary structure is incredibly stable, which provides further support for this 2-state catch bond model linking the cytoskeleton and the ternary complex.Citation62 Thus α-catenin serves as the dynamic link between cadherin adhesion and the cytoskeleton.
δ-catenin/p120-cateninCitation63 plays a role in maintaining synaptic contactsCitation64 as well as dendritic spines.Citation65 However, while present in neural precursorsCitation66 no specific function has been identified during neocortical development. No function of any kind has been described in neural tissue for γ-catenin/plakoglobin.Citation67 As such, the other members of the catenin family, γ- and δ-catenin, will not be discussed in detail in this review.
β-Catenin signaling regulates cortical progenitor proliferation and differentiation
As the downstream mediator of canonical Wnt signaling and an essential component of the cadherin adherens complex, β-catenin is positioned at the intersection of cell-cell adhesion and signaling. In the developing cerebral cortex, transgenic overexpression of a degradation-resistant β-catenin caused massive enlargement of the cortical progenitor poolCitation2 and expansion of cortical surface area.Citation68 Complementary studies provided evidence that the signaling function of β-catenin was critical in regulating cell proliferation in cortical development.Citation69 While these and many other studiesCitation70-78 pointed to the importance of β-catenin signaling in development, how adhesion and signaling might interact remained difficult to dissect experimentally.
Regulation of proliferation by cadherins in cortical development depends on context
As in other epithelia, cadherin adhesion in the developing nervous system regulates tissue integrity. Following closure of the neural tube N-cadherin is expressed in all portions of the tube except for the neural crest.Citation79,80 Injection of an N-cadherin function blocking antibody into the developing diencephalon of chick embryos caused a profound disruption in epithelial structure and the formation of neuroepithelial rosettes.Citation38 These findings were recapitulated in the developing cortex of mice where conditional deletion of N-cadherin resulted in disruption of the normal laminar organization of the cortex and a complete loss of apically concentrated adherens junction component expression.Citation3 Similarly, conditional deletion of β-catenin,Citation4 or αE-cateninCitation5,6 from neural precursors caused profound disruption of cortical layering and loss of the apically-located adherens junctions.
The striking architectural disorganization of the developing cortex following adherens junction disruption was accompanied by the mis-regulation of cell proliferation. When N-cadherin transport and localization were altered by conditional deletion of a subunit of the KIF3 molecular motor, KAP3 (kinesin-associated protein 3), hyperproliferation of neural progenitors and enlargement of the cortex resulted.Citation81 Similarly, conditional deletion of αE-catenin caused hyperproliferation of cortical progenitors and overgrowth of the cerebral cortex.Citation5 While Lien et al. observed upregulation of the hedgehog signaling pathway in the cortices of the conditional αE-catenin knockout mice, the specific mechanisms underlying the control of proliferation by cell adhesion remain poorly understood. The regulation of cell polarity and cell growth are intimately linked,Citation82 and the maintenance of distinct cellular domains by adherens junction adhesion likely plays a critical role in the proper balance of pro- and anti-proliferative signals.
In contrast to the hyperproliferation observed when adhesion and cell polarity was globally disrupted, focal inhibition of cadherin adhesion in an intact neuroepithelium appears to reduce, instead of increase, pro-proliferative signaling. In utero electroporation methods enabled the focal loss of adherens proteins while preserving the integrity of the VZ. Instead of the epithelial disorganization and hyperproliferation that resulted from widespread deletion of αE-catenin, focal deletion of αE-catenin resulted in premature cell-cycle exit, neuronal differentiation, and migration from the ventricular zone to the cortical plate.Citation6 The cells in which αE-catenin was reduced also showed reduction in β-catenin mediated Wnt signaling, and rescuing β-catenin signaling largely restores proliferation.Citation6 Similarly, cell-autonomous deletion of N-cadherin in neural precursors caused premature cell-cycle exit, neuronal differentiation, migration from the VZ, as well as reduced β-catenin mediated Wnt signaling.Citation7-8
Together these studies suggest that cadherin adhesion in cortical progenitors in a normal, intact VZ promotes neural progenitor self-renewal via activation of β-catenin signaling. The hyperproliferation observed in tissue-wide knockout of cadherin adhesion might therefore result indirectly from the loss of cell polarity and tissue organization. Pro-proliferative signals or their receptors may be released from their normal subcellular locations and regulation with loss of apical-basal polarity. Taken together, these findings suggest that the regulation of cell proliferation by cadherin adhesion is highly context-dependent in developing tissues and organs.
Cadherin adhesion and the neural progenitor niche
The local microenvironment, or niche, plays an important function in regulating the development of stem and progenitor cells. The physical attachment of stem/progenitor cells to the niche is important for maintaining stem cell identity and function.Citation83-86 The structural organization of the developing cortical VZ differs from the typical stem cell niche in that it lacks specific support cells, and is comprised exclusively of proliferating progenitor cells.Citation87
We proposed that cadherin adhesion in the cortical VZ functions to sustain a proliferative niche where neural precursors support and maintain themselves.Citation7 Cell-cell adhesion in the VZ niche likely provides them with optimized exposure to pro-proliferative Wnts expressed by progenitors in the VZ.Citation88 Exit and migration from the VZ would ensure that pro-proliferative Wnt signaling was reduced, and indeed, Wnt signaling activity is markedly reduced outside of the VZ.Citation69,77 However, the model that cadherin maintenance of β-catenin signaling occurs solely via physical retention of cells in the VZ is no doubt overly simplistic. We observed that electroporated progenitors still located in the VZ showed reduced β-catenin signaling after N-cadherin or αE-catenin reduction, indicating that reductions in β-catenin signaling preceded exit from the VZ.Citation6-8 Therefore, cadherin cell-cell adhesion appears to serve at least 2 related functions to promote Wnt/β-catenin signaling in cortical development: 1) to directly maintain β-catenin signaling in the VZ, and 2) to physically retain cells in the VZ to ensure proximity to pro-proliferative Wnt ligands.
What is the evidence that cadherin adhesion directly positively regulates Wnt/β-catenin signaling? A simple model, where cadherins set a threshold for β-catenin signaling, was suggested by numerous observations that forced overexpression of cadherins antagonized β-catenin transcriptional activation.Citation89-93 In this model, cadherin is able to titrate available β-catenin from its role in Wnt signaling.Citation89-93 Our observations that cadherin adhesion can also positively regulate Wnt/β-catenin signaling support increasing recent evidence that the relationship between cadherin adhesion and β-catenin signaling is context-dependent.Citation94 In the developing cortex, N-cadherin appears to function in Wnt signaling both through phosphorylation of LRP6 and activation of AKT signaling.Citation8 The assembly of functional Wnt signaling receptor complex also requires cadherin.Citation95-97 Moreover, a recent study suggests that only cadherin-associated β-catenin is Wnt-signaling competent, and functionally distinct from β-catenin that has never interacted with cadherin.Citation94 Cadherin also appears to maintain a pool of β-catenin that can be available for signal transduction, supporting studies suggesting that cadherin-bound β-catenin can translocate to the nucleus and activate transcription.Citation94,98,99
Distinct from its role in Wnt-activation of β-catenin, N-cadherin can also stimulate β-catenin transcriptional activation through AKT. N-cadherin adhesion triggers PI3K-mediated activation of AKT.Citation100-102 In turn, activated AKT signaling can stimulate β-catenin signaling. In intestinal stem cells the loss of a negative regulator of PI3K/AKT, PTEN, leads to β-catenin stabilization and upregulated β-catenin signaling.Citation103 AKT phosphorylates β-catenin at Serine residue 552, priming it for 14-3-3ζ binding and stabilization, which in turn allows β-catenin to translocate and accumulate in the nucleus.Citation104 AKT can also phosphorylate and inactivate GSK3β at Serine 9, which leads to stabilization and nuclear accumulation of β-catenin.Citation105 In cortical progenitors, AKT activation also results in stabilization and activation of β-catenin signaling by direct phosphorylation at Serine 552.Citation7-8
In addition to Wnt/β-catenin and PI3K/β-catenin signaling, appropriate regulation of other signaling pathways important in neural development depend on adherens junctions. A single cilium extends from the apical process of cortical progenitors.Citation106 Recent studies have supported a role for cilia in coordinating critical signaling pathways in neural development such as SHH.Citation107 Although the relationship of adherens junctions and the regulation of cilia-based signaling pathways remains poorly understood, recent evidence that the planar cell polarity (PCP) proteins implicated in ciliogenesis must be first positioned apicallyCitation107 suggest that adherens junctions, apical-basal polarity, and planar polarity are intimately linked. Indeed, the findings of upregulated hedgehog (Hh) signaling following αE-cateninCitation5 or N-cadherin deletionCitation108 suggest that intact epithelial architecture functions to restrain Hh signaling. The observations that Hh signaling maintains adherens junctions in zebrafish neural tube suggests a potential reciprocal inhibitory relationship between adherens junctions and SHH signaling.Citation108
The signaling machinery critical for polarity, adhesion, and proliferation cluster at the apical foot process of VZ precursors,Citation3,7,30,109,110 where the high density of these pathway components indicates the potential for cross-talk. A growing amount of data indicates that there are physical interactions between various components of these different cellular processes. APC, a component of the destruction complex that phosphorylates β-catenin to target it for degradation, is also known to localize to the cellular membrane as well as regulate polarity.Citation111,112 Findings in osteoblasts suggest that the destruction complex is not just located near the cellular membrane, but physically anchored to the adherens junction.Citation113 Recent reports have also demonstrated that the Wnt signaling receptor LRP5/6 binds to the adherens junction.Citation95 Casein Kinase 1 (CK1), which along with GSK3β targets β-catenin for destruction through phosphorylation, plays an essential role in Wnt receptor complex activation through the phosphorylation of LRP6, and the association of CK1 with LRP5/6 requires cadherin.Citation96 Furthermore, p120-catenin (δ-catenin) appears to control the assembly of the Wnt signaling complex through interactions with cadherin and CK1.Citation97
The high concentration of components critical for polarity, adhesion, and proliferation in the apical foot process may form an extra-nuclear hub to help foster the self-supportive neural stem/progenitor cell niche (). A reduction in adhesion could subsequently cause a cascade of changes in critical pathways (e.g. Wnt signaling, PI3K/Akt signaling, Hh signaling) with components proximal to or directly anchored to adherens junctions. It is also possible that the loss or disruption of fate-influencing polarity cues (e.g., PAR3, aPKC) could destabilize and ultimately remove adherens junctions.Citation109 The ability to influence multiple pathways in a restricted physical space may be important for coordinating the processes of delamination and neuronal differentiation following a reduction in adhesion.
Figure 2. Hypothetical signaling hub, or extra-nuclear hub, containing both adherens junction and Wnt signaling components at the apical surface. The high density of adherens junction and Wnt signaling components located in the apical end feet of neural precursors located in the VZ, coupled with emerging data suggests that these pathways may physically and functionally interact. In the absence of a Wnt signal, a destruction complex comprised of Axin and APC bind to β-catenin (β) and recruit the kinases GSK3β and CK1, which phosphorylate β-catenin thereby targeting it for ubiquitination and ultimately destruction. In the presence of a Wnt ligand, a Frizzled (Fzd) and LRP5/6 complex is formed and bound by Dishevelled (Dvl), leading to the phosphorylation of the LRP5/6 receptor by several kinases, including GSK3β and CK1. The phosphorylated LRP5/6 receptor recruits axin to the plasma membrane, leading to the decay of the destruction complex, and allowing stabilized β-catenin to be translocated to the nucleus. A growing number of studies suggest that several key components of Wnt signaling may physically interact with members of the adherens junction, in particular N-cadherin (N-cad). N-cadherin binds both p120-catenin (p120) at the JMD domain (juxtamembrane intracellular domain) and β-catenin at the CBD domain (catenin binding domain). β-catenin in turn binds to α-catenin (α), which is dynamically tethered to the actin cytoskeleton. PI3K, which through activation of AKT stabilizes β-catenin allowing it to participate in signaling, has also been shown to interact with N-cadherin. The research detailing additional potential interactions are detailed in the main text.
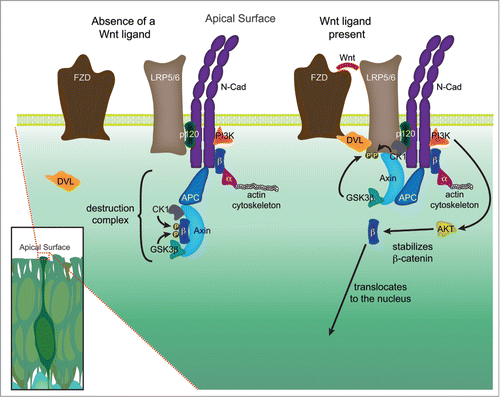
Summary
Recent experiments examining the roles of αE-catenin and N-cadherin have demonstrated that proper adhesive function is important in maintaining neural precursors within the proliferative ventricular zone of the developing neocortex, as well as maintaining β-catenin mediated Wnt signaling. Furthermore, adherens junctions help maintain a self supportive proliferative neural stem cell niche. The apical concentration of adhesive and signaling molecules may provide an extra-nuclear hub utilized to sustain the proliferative neuroepithelium during development. Future studies investigating the roles of these apically concentrated signaling molecules play in maintaining a proliferative microdomain or niche, should be fruitful and elucidating. Moreover, understanding which pathways intersect and how/when they intersect will be useful not only to the field of developmental neurobiology but many others (e.g. cancer) where alterations in cell-cell adhesion impact cell behaviors.
Disclosure of Potential Conflicts of Interest
No potential conflicts of interest were disclosed.
Acknowledgments
We would like to thank Jianing Zhang, Kyucheol Cho, and Daichi Kawaguchi for providing helpful commentary and critiques of this manuscript during its preparation.
References
- Rakic P. A small step for the cell, a giant leap for mankind: a hypothesis of neocortical expansion during evolution. Trends Neurosci 1995; 18:383-8; PMID:7482803; http://dx.doi.org/10.1016/0166-2236(95)93934-P
- Chenn A, Walsh CA. Regulation of cerebral cortical size by control of cell cycle exit in neural precursors. Science 2002; 297:365-9; PMID:12130776; http://dx.doi.org/10.1126/science.1074192
- Kadowaki M, Nakamura S, Machon O, Krauss S, Radice GL, Takeichi M. N-cadherin mediates cortical organization in the mouse brain. Dev Biol 2007; 304:22-33; PMID:17222817; http://dx.doi.org/10.1016/j.ydbio.2006.12.014
- Junghans D, Hack I, Frotscher M, Taylor V, Kemler R. Beta-catenin-mediated cell-adhesion is vital for embryonic forebrain development. Dev Dyn 2005; 233:528-39; PMID:15844200; http://dx.doi.org/10.1002/dvdy.20365
- Lien WH, Klezovitch O, Fernandez TE, Delrow J, Vasioukhin V. alphaE-catenin controls cerebral cortical size by regulating the hedgehog signaling pathway. Science 2006; 311:1609-12; PMID:16543460; http://dx.doi.org/10.1126/science.1121449
- Stocker AM, Chenn A. Focal reduction of alphaE-catenin causes premature differentiation and reduction of beta-catenin signaling during cortical development. Dev Biol 2009; 328:66-77; PMID:19389371; http://dx.doi.org/10.1016/j.ydbio.2009.01.010
- Zhang J, Woodhead GJ, Swaminathan SK, Noles SR, McQuinn ER, Pisarek AJ, Stocker AM, Mutch CA, Funatsu N, Chenn A. Cortical neural precursors inhibit their own differentiation via N-cadherin maintenance of beta-catenin signaling. Dev Cell 2010; 18:472-9; PMID:20230753; http://dx.doi.org/10.1016/j.devcel.2009.12.025
- Zhang J, Shemezis JR, McQuinn ER, Wang J, Sverdlov M, Chenn A. AKT activation by N-cadherin regulates beta-catenin signaling and neuronal differentiation during cortical development. Neural Dev 2013; 8:7; PMID:23618343; http://dx.doi.org/10.1186/1749-8104-8-7
- Bayer SA, Altman J. Neocortical development. New York: Raven Press, 1991.
- Rakic P. Mode of cell migration to the superficial layers of fetal monkey neocortex. J Comp Neurol 1972; 145:61-83; PMID:4624784
- Halliday AL, Cepko CL. Generation and migration of cells in the developing striatum. Neuron 1992; 9:15-26; PMID:1632968; http://dx.doi.org/10.1016/0896-6273(92)90216-Z
- Misson JP, Edwards MA, Yamamoto M, Caviness VS, Jr. Mitotic cycling of radial glial cells of the fetal murine cerebral wall: a combined autoradiographic and immunohistochemical study. Brain Res 1988; 466:183-90; PMID:3359310; http://dx.doi.org/10.1016/0165-3806(88)90043-0
- Gray GE, Sanes JR. Lineage of radial glia in the chicken optic tectum. Development 1992; 114:271-83; PMID:1576964
- Miyata T, Kawaguchi A, Okano H, Ogawa M. Asymmetric inheritance of radial glial fibers by cortical neurons. Neuron 2001; 31:727-41; PMID:11567613; http://dx.doi.org/10.1016/S0896-6273(01)00420-2
- Noctor SC, Flint AC, Weissman TA, Dammerman RS, Kriegstein AR. Neurons derived from radial glial cells establish radial units in neocortex. Nature 2001; 409:714-20; PMID:11217860; http://dx.doi.org/10.1038/35055553
- Haubensak W, Attardo A, Denk W, Huttner WB. Neurons arise in the basal neuroepithelium of the early mammalian telencephalon: a major site of neurogenesis. Proc Natl Acad Sci U S A 2004; 101:3196-201; PMID:14963232; http://dx.doi.org/10.1073/pnas.0308600100
- Miyata T, Kawaguchi A, Saito K, Kawano M, Muto T, Ogawa M. Asymmetric production of surface-dividing and non-surface-dividing cortical progenitor cells. Development 2004; 131:3133-45; PMID:15175243; http://dx.doi.org/10.1242/dev.01173
- Noctor SC, Martinez-Cerdeno V, Ivic L, Kriegstein AR. Cortical neurons arise in symmetric and asymmetric division zones and migrate through specific phases. Nat Neurosci 2004; 7:136-44; PMID:14703572; http://dx.doi.org/10.1038/nn1172
- Stancik EK, Navarro-Quiroga I, Sellke R, Haydar TF. Heterogeneity in ventricular zone neural precursors contributes to neuronal fate diversity in the postnatal neocortex. J Neurosci 2010; 30:7028-36; PMID:20484645; http://dx.doi.org/10.1523/JNEUROSCI.6131-09.2010
- Chenn A, McConnell SK. Cleavage orientation and the asymmetric inheritance of Notch1 immunoreactivity in mammalian neurogenesis. Cell 1995; 82:631-41; PMID:7664342; http://dx.doi.org/10.1016/0092-8674(95)90035-7
- Haydar TF, Ang E, Jr., Rakic P. Mitotic spindle rotation and mode of cell division in the developing telencephalon. Proc Natl Acad Sci U S A 2003; 100:2890-5; PMID:12589023; http://dx.doi.org/10.1073/pnas.0437969100
- Kosodo Y, Roper K, Haubensak W, Marzesco AM, Corbeil D, Huttner WB. Asymmetric distribution of the apical plasma membrane during neurogenic divisions of mammalian neuroepithelial cells. EMBO J 2004; 23:2314-24; PMID:15141162; http://dx.doi.org/10.1038/sj.emboj.7600223
- Leone DP, Srinivasan K, Chen B, Alcamo E, McConnell SK. The determination of projection neuron identity in the developing cerebral cortex. Curr Opin Neurobiol 2008; 18:28-35; PMID:18508260; http://dx.doi.org/10.1016/j.conb.2008.05.006
- Knoblich JA. Mechanisms of asymmetric stem cell division. Cell 2008; 132:583-97; PMID:18295577
- Hardwick LJ, Philpott A. Nervous decision-making: to divide or differentiate. Trends Genet 2014; 30:254-61; PMID:24791612; http://dx.doi.org/10.1016/j.tig.2014.04.001
- Lehtinen MK, Zappaterra MW, Chen X, Yang YJ, Hill AD, Lun M, Maynard T, Gonzalez D, Kim S, Ye P, et al. The cerebrospinal fluid provides a proliferative niche for neural progenitor cells. Neuron 2011; 69:893-905; PMID:21382550; http://dx.doi.org/10.1016/j.neuron.2011.01.023
- Vorhagen S, Niessen CM. Mammalian aPKC/Par polarity complex mediated regulation of epithelial division orientation and cell fate. Exp Cell Res 2014; 328:296-302; PMID:25128813; http://dx.doi.org/10.1016/j.yexcr.2014.08.008
- Januschke J, Nathke I. Stem cell decisions: a twist of fate or a niche market? Semin Cell Dev Biol 2014; 34:116-23; PMID:24613913; http://dx.doi.org/10.1016/j.semcdb.2014.02.014
- Doe CQ, Fuerstenberg S, Peng CY. Neural stem cells: from fly to vertebrates. J Neurobiol 1998; 36:111-27; PMID:9712299; http://dx.doi.org/10.1002/(SICI)1097-4695(199808)36:2%3c111::AID-NEU2%3e3.0.CO;2-4
- Chenn A, Braisted JE, McConnell SK, O'Leary DD. Development of the cerebral cortex: mechanisms controlling cell fate, laminar and areal patterning, and axonal connectivity. In: Cowan WM, Zipursky SL, Jessell TM, eds. Molecular and Cellular Approaches to Neural Development. New York: Oxford University Press, 1997:440-73.
- Tsunekawa Y, Kikkawa T, Osumi N. Asymmetric inheritance of Cyclin D2 maintains proliferative neural stem/progenitor cells: a critical event in brain development and evolution. Dev Growth Differ 2014; 56:349-57; PMID:24835888; http://dx.doi.org/10.1111/dgd.12135
- Wang X, Tsai JW, Imai JH, Lian WN, Vallee RB, Shi SH. Asymmetric centrosome inheritance maintains neural progenitors in the neocortex. Nature 2009; 461:947-55; PMID:19829375; http://dx.doi.org/10.1038/nature08435
- Paridaen JT, Wilsch-Brauninger M, Huttner WB. Asymmetric inheritance of centrosome-associated primary cilium membrane directs ciliogenesis after cell division. Cell 2013; 155:333-44; PMID:24120134; http://dx.doi.org/10.1016/j.cell.2013.08.060
- Bultje RS, Castaneda-Castellanos DR, Jan LY, Jan YN, Kriegstein AR, Shi SH. Mammalian Par3 regulates progenitor cell asymmetric division via notch signaling in the developing neocortex. Neuron 2009; 63:189-202; PMID:19640478; http://dx.doi.org/10.1016/j.neuron.2009.07.004
- Nelson WJ. Adaptation of core mechanisms to generate cell polarity. Nature 2003; 422:766-74; PMID:12700771
- Halbleib JM, Nelson WJ. Cadherins in development: cell adhesion, sorting, and tissue morphogenesis. Genes Dev 2006; 20:3199-214; PMID:17158740; http://dx.doi.org/10.1101/gad.1486806
- Lu B, Roegiers F, Jan LY, Jan YN. Adherens junctions inhibit asymmetric division in the Drosophila epithelium. Nature 2001; 409:522-5; PMID:11206549; http://dx.doi.org/10.1038/35054077
- Ganzler-Odenthal SI, Redies C. Blocking N-cadherin function disrupts the epithelial structure of differentiating neural tissue in the embryonic chicken brain. J Neurosci 1998; 18:5415-25; PMID:9651223
- Harmon RM, Desai BV, Green KJ. Regulatory roles of the cadherin superfamily. F1000 Biol Rep 2009; 1:13; PMID:20948673
- Boggon TJ, Murray J, Chappuis-Flament S, Wong E, Gumbiner BM, Shapiro L. C-cadherin ectodomain structure and implications for cell adhesion mechanisms. Science 2002; 296:1308-13; PMID:11964443; http://dx.doi.org/10.1126/science.1071559
- Tomschy A, Fauser C, Landwehr R, Engel J. Homophilic adhesion of E-cadherin occurs by a co-operative two-step interaction of N-terminal domains. EMBO J 1996; 15:3507-14; PMID:8670853
- Adams CL, Chen YT, Smith SJ, Nelson WJ. Mechanisms of epithelial cell-cell adhesion and cell compaction revealed by high-resolution tracking of E-cadherin-green fluorescent protein. J Cell Biol 1998; 142:1105-19; PMID:9722621; http://dx.doi.org/10.1083/jcb.142.4.1105
- Yap AS, Niessen CM, Gumbiner BM. The juxtamembrane region of the cadherin cytoplasmic tail supports lateral clustering, adhesive strengthening, and interaction with p120ctn. J Cell Biol 1998; 141:779-89; PMID:9566976; http://dx.doi.org/10.1083/jcb.141.3.779
- Aberle H, Butz S, Stappert J, Weissig H, Kemler R, Hoschuetzky H. Assembly of the cadherin-catenin complex in vitro with recombinant proteins. J Cell Sci 1994; 107(Pt 12):3655-63; PMID:7706414
- Ozawa M, Ringwald M, Kemler R. Uvomorulin-catenin complex formation is regulated by a specific domain in the cytoplasmic region of the cell adhesion molecule. Proc Natl Acad Sci U S A 1990; 87:4246-50; PMID:2349235; http://dx.doi.org/10.1073/pnas.87.11.4246
- Bryant DM, Stow JL. The ins and outs of E-cadherin trafficking. Trends Cell Biol 2004; 14:427-34; PMID:15308209; http://dx.doi.org/10.1016/j.tcb.2004.07.007
- Riggleman B, Wieschaus E, Schedl P. Molecular analysis of the armadillo locus: uniformly distributed transcripts and a protein with novel internal repeats are associated with a Drosophila segment polarity gene. Genes Dev 1989; 3:96-113; PMID:2707602; http://dx.doi.org/10.1101/gad.3.1.96
- Huber AH, Nelson WJ, Weis WI. Three-dimensional structure of the armadillo repeat region of beta-catenin. Cell 1997; 90:871-82; PMID:9298899; http://dx.doi.org/10.1016/S0092-8674(00)80352-9
- Hulsken J, Birchmeier W, Behrens J. E-cadherin and APC compete for the interaction with beta-catenin and the cytoskeleton. J Cell Biol 1994; 127:2061-9; PMID:7806582; http://dx.doi.org/10.1083/jcb.127.6.2061
- Orsulic S, Peifer M. An in vivo structure-function study of armadillo, the beta-catenin homologue, reveals both separate and overlapping regions of the protein required for cell adhesion and for wingless signaling. J Cell Biol 1996; 134:1283-300; PMID:8794868; http://dx.doi.org/10.1083/jcb.134.5.1283
- Aberle H, Schwartz H, Hoschuetzky H, Kemler R. Single amino acid substitutions in proteins of the armadillo gene family abolish their binding to alpha-catenin. J Biol Chem 1996; 271:1520-6; PMID:8576147; http://dx.doi.org/10.1074/jbc.271.3.1520
- Pai LM, Kirkpatrick C, Blanton J, Oda H, Takeichi M, Peifer M. Drosophila alpha-catenin and E-cadherin bind to distinct regions of Drosophila Armadillo. J Biol Chem 1996; 271:32411-20; PMID:8943306; http://dx.doi.org/10.1074/jbc.271.50.32411
- McCrea PD, Brieher WM, Gumbiner BM. Induction of a secondary body axis in Xenopus by antibodies to beta-catenin. J Cell Biol 1993; 123:477-84; PMID:8408227; http://dx.doi.org/10.1083/jcb.123.2.477
- Munemitsu S, Albert I, Souza B, Rubinfeld B, Polakis P. Regulation of intracellular beta-catenin levels by the adenomatous polyposis coli (APC) tumor-suppressor protein. Proc Natl Acad Sci U S A 1995; 92:3046-50; PMID:7708772; http://dx.doi.org/10.1073/pnas.92.7.3046
- Korinek V, Barker N, Morin PJ, van Wichen D, de Weger R, Kinzler KW, Vogelstein B, Clevers H. Constitutive transcriptional activation by a beta-catenin-Tcf complex in APC-/- colon carcinoma. Science 1997; 275:1784-7; PMID:9065401; http://dx.doi.org/10.1126/science.275.5307.1784
- Morin PJ, Sparks AB, Korinek V, Barker N, Clevers H, Vogelstein B, Kinzler KW. Activation of beta-catenin-Tcf signaling in colon cancer by mutations in beta-catenin or APC. Science 1997; 275:1787-90; PMID:9065402; http://dx.doi.org/10.1126/science.275.5307.1787
- Behrens J, von Kries JP, Kuhl M, Bruhn L, Wedlich D, Grosschedl R, Birchmeier W. Functional interaction of beta-catenin with the transcription factor LEF-1. Nature 1996; 382:638-42; PMID:8757136; http://dx.doi.org/10.1038/382638a0
- van de Wetering M, Cavallo R, Dooijes D, van Beest M, van Es J, Loureiro J, Ypma A, Hursh D, Jones T, Bejsovec A, et al. Armadillo coactivates transcription driven by the product of the Drosophila segment polarity gene dTCF. Cell 1997; 88:789-99; PMID:9118222; http://dx.doi.org/10.1016/S0092-8674(00)81925-X
- Hsu SC, Galceran J, Grosschedl R. Modulation of transcriptional regulation by LEF-1 in response to Wnt-1 signaling and association with beta-catenin. Mol Cell Biol 1998; 18:4807-18; PMID:9671490
- Rimm DL, Koslov ER, Kebriaei P, Cianci CD, Morrow JS. Alpha 1(E)-catenin is an actin-binding and -bundling protein mediating the attachment of F-actin to the membrane adhesion complex. Proc Natl Acad Sci U S A 1995; 92:8813-7; PMID:7568023
- Buckley CD, Tan J, Anderson KL, Hanein D, Volkmann N, Weis WI, Nelson WJ, Dunn AR. Cell adhesion. The minimal cadherin-catenin complex binds to actin filaments under force. Science 2014; 346:1254211; PMID:25359979; http://dx.doi.org/10.1126/science.1254211
- Pokutta S, Choi HJ, Ahlsen G, Hansen SD, Weis WI. Structural and thermodynamic characterization of cadherin. beta-catenin.alpha-catenin complex formation. J Biol Chem 2014; 289:13589-601; PMID:24692547; http://dx.doi.org/10.1074/jbc.M114.554709
- Reynolds AB, Roesel DJ, Kanner SB, Parsons JT. Transformation-specific tyrosine phosphorylation of a novel cellular protein in chicken cells expressing oncogenic variants of the avian cellular src gene. Mol Cell Biol 1989; 9:629-38; PMID:2469003
- Matter C, Pribadi M, Liu X, Trachtenberg JT. Delta-catenin is required for the maintenance of neural structure and function in mature cortex in vivo. Neuron 2009; 64:320-7; PMID:19914181; http://dx.doi.org/10.1016/j.neuron.2009.09.026
- Brigidi GS, Sun Y, Beccano-Kelly D, Pitman K, Mobasser M, Borgland SL, Milnerwood AJ, Bamji SX. Palmitoylation of delta-catenin by DHHC5 mediates activity-induced synapse plasticity. Nat Neurosci 2014; 17:522-32; PMID:24562000; http://dx.doi.org/10.1038/nn.3657
- Chenn A, Zhang YA, Chang BT, McConnell SK. Intrinsic polarity of mammalian neuroepithelial cells. Mol Cell Neurosci 1998; 11:183-93; PMID:9675050; http://dx.doi.org/10.1006/mcne.1998.0680
- McCrea PD, Turck CW, Gumbiner B. A homolog of the armadillo protein in Drosophila (plakoglobin) associated with E-cadherin. Science 1991; 254:1359-61; PMID:1962194; http://dx.doi.org/10.1126/science.1962194
- Chenn A, Walsh CA. Increased neuronal production, enlarged forebrains and cytoarchitectural distortions in beta-catenin overexpressing transgenic mice. Cereb Cortex 2003; 13:599-606; PMID:12764034; http://dx.doi.org/10.1093/cercor/13.6.599
- Woodhead GJ, Mutch CA, Olson EC, Chenn A. Cell-autonomous beta-catenin signaling regulates cortical precursor proliferation. J Neurosci 2006; 26:12620-30; PMID:17135424; http://dx.doi.org/10.1523/JNEUROSCI.3180-06.2006
- Backman M, Machon O, Mygland L, van den Bout CJ, Zhong W, Taketo MM, Krauss S. Effects of canonical Wnt signaling on dorso-ventral specification of the mouse telencephalon. Dev Biol 2005; 279:155-68; PMID:15708565; http://dx.doi.org/10.1016/j.ydbio.2004.12.010
- Machon O, van den Bout CJ, Backman M, Kemler R, Krauss S. Role of beta-catenin in the developing cortical and hippocampal neuroepithelium. Neuroscience 2003; 122:129-43; PMID:14596855; http://dx.doi.org/10.1016/S0306-4522(03)00519-0
- Wrobel CN, Mutch CA, Swaminathan S, Taketo MM, Chenn A. Persistent expression of stabilized beta-catenin delays maturation of radial glial cells into intermediate progenitors. Dev Biol 2007; 309:285-97; PMID:17706960; http://dx.doi.org/10.1016/j.ydbio.2007.07.013
- Zechner D, Fujita Y, Hulsken J, Muller T, Walther I, Taketo MM, Crenshaw EB, 3rd, Birchmeier W, Birchmeier C. beta-Catenin signals regulate cell growth and the balance between progenitor cell expansion and differentiation in the nervous system. Dev Biol 2003; 258:406-18; PMID:12798297; http://dx.doi.org/10.1016/S0012-1606(03)00123-4
- Mutch CA, Schulte JD, Olson E, Chenn A. Beta-catenin signaling negatively regulates intermediate progenitor population numbers in the developing cortex. PLoS One 2010; 5:e12376; PMID:20811503; http://dx.doi.org/10.1371/journal.pone.0012376
- Gulacsi AA, Anderson SA. Beta-catenin-mediated Wnt signaling regulates neurogenesis in the ventral telencephalon. Nat Neurosci 2008; 11:1383-91; PMID:18997789; http://dx.doi.org/10.1038/nn.2226
- Zhou CJ, Borello U, Rubenstein JL, Pleasure SJ. Neuronal production and precursor proliferation defects in the neocortex of mice with loss of function in the canonical Wnt signaling pathway. Neuroscience 2006; 142:1119-31; PMID:16920270; http://dx.doi.org/10.1016/j.neuroscience.2006.07.007
- Mutch CA, Funatsu N, Monuki ES, Chenn A. Beta-catenin signaling levels in progenitors influence the laminar cell fates of projection neurons. J Neurosci 2009; 29:13710-9; PMID:19864583; http://dx.doi.org/10.1523/JNEUROSCI.3022-09.2009
- Hirabayashi Y, Itoh Y, Tabata H, Nakajima K, Akiyama T, Masuyama N, Gotoh Y. The Wnt/beta-catenin pathway directs neuronal differentiation of cortical neural precursor cells. Development 2004; 131:2791-801; PMID:15142975; http://dx.doi.org/10.1242/dev.01165
- Bronner-Fraser M, Wolf JJ, Murray BA. Effects of antibodies against N-cadherin and N-CAM on the cranial neural crest and neural tube. Dev Biol 1992; 153:291-301; PMID:1397686; http://dx.doi.org/10.1016/0012-1606(92)90114-V
- Nakagawa S, Takeichi M. Neural crest cell-cell adhesion controlled by sequential and subpopulation-specific expression of novel cadherins. Development 1995; 121:1321-32; PMID:7540531
- Teng J, Rai T, Tanaka Y, Takei Y, Nakata T, Hirasawa M, Kulkarni AB, Hirokawa N. The KIF3 motor transports N-cadherin and organizes the developing neuroepithelium. Nat Cell Biol 2005; 7:474-82; PMID:15834408; http://dx.doi.org/10.1038/ncb1249
- Bilder D, Li M, Perrimon N. Cooperative Regulation of Cell Polarity and Growth by Drosophila Tumor Suppressors. Science 2000; 289:113-6; PMID:10884224; http://dx.doi.org/10.1126/science.289.5476.113
- Maeda K, Takemura M, Umemori M, Adachi-Yamada T. E-cadherin prolongs the moment for interaction between intestinal stem cell and its progenitor cell to ensure Notch signaling in adult Drosophila midgut. Genes Cells 2008; 13:1219-27; PMID:19021776; http://dx.doi.org/10.1111/j.1365-2443.2008.01239.x
- Ohlstein B, Spradling A. Multipotent Drosophila intestinal stem cells specify daughter cell fates by differential notch signaling. Science 2007; 315:988-92; PMID:17303754; http://dx.doi.org/10.1126/science.1136606
- Song X, Zhu CH, Doan C, Xie T. Germline stem cells anchored by adherens junctions in the Drosophila ovary niches. Science 2002; 296:1855-7; PMID:12052957; http://dx.doi.org/10.1126/science.1069871
- Jin Z, Kirilly D, Weng C, Kawase E, Song X, Smith S, Schwartz J, Xie T. Differentiation-defective stem cells outcompete normal stem cells for niche occupancy in the Drosophila ovary. Cell Stem Cell 2008; 2:39-49; PMID:18371420; http://dx.doi.org/10.1016/j.stem.2007.10.021
- Takahashi T, Nowakowski RS, Caviness VS, Jr. Cell cycle parameters and patterns of nuclear movement in the neocortical proliferative zone of the fetal mouse. J Neurosci 1993; 13:820-33; PMID:8426239
- Viti J, Gulacsi A, Lillien L. Wnt regulation of progenitor maturation in the cortex depends on Shh or fibroblast growth factor 2. J Neurosci 2003; 23:5919-27; PMID:12843296
- Eger A, Stockinger A, Schaffhauser B, Beug H, Foisner R. Epithelial mesenchymal transition by c-Fos estrogen receptor activation involves nuclear translocation of beta-catenin and upregulation of beta-catenin/lymphoid enhancer binding factor-1 transcriptional activity. J Cell Biol 2000; 148:173-88; PMID:10629227; http://dx.doi.org/10.1083/jcb.148.1.173
- Orsulic S, Huber O, Aberle H, Arnold S, Kemler R. E-cadherin binding prevents beta-catenin nuclear localization and beta-catenin/LEF-1-mediated transactivation. J Cell Sci 1999; 112(Pt 8):1237-45; PMID:10085258
- Sanson B, White P, Vincent JP. Uncoupling cadherin-based adhesion from wingless signalling in Drosophila. Nature 1996; 383:627-30; PMID:8857539; http://dx.doi.org/10.1038/383627a0
- Fagotto F, Funayama N, Gluck U, Gumbiner BM. Binding to cadherins antagonizes the signaling activity of beta-catenin during axis formation in Xenopus. J Cell Biol 1996; 132:1105-14; PMID:8601588; http://dx.doi.org/10.1083/jcb.132.6.1105
- Heasman J, Crawford A, Goldstone K, Garner-Hamrick P, Gumbiner B, McCrea P, Kintner C, Noro CY, Wylie C. Overexpression of cadherins and underexpression of beta-catenin inhibit dorsal mesoderm induction in early Xenopus embryos. Cell 1994; 79:791-803; PMID:7528101; http://dx.doi.org/10.1016/0092-8674(94)90069-8
- Howard S, Deroo T, Fujita Y, Itasaki N. A positive role of cadherin in Wnt/beta-catenin signalling during epithelial-mesenchymal transition. PLoS One 2011; 6:e23899; PMID:21909376
- Hay E, Buczkowski T, Marty C, Da Nascimento S, Sonnet P, Marie PJ. Peptide-based mediated disruption of N-cadherin-LRP5/6 interaction promotes Wnt signalling and bone formation. J Bone Miner Res 2012; PMID:22576936
- Del Valle-Perez B, Arques O, Vinyoles M, de Herreros AG, Dunach M. Coordinated action of CK1 isoforms in canonical Wnt signaling. Mol Cell Biol 2011; 31:2877-88; PMID:21606194; http://dx.doi.org/10.1128/MCB.01466-10
- Casagolda D, Del Valle-Perez B, Valls G, Lugilde E, Vinyoles M, Casado-Vela J, Solanas G, Batlle E, Reynolds AB, Casal JI, et al. A p120-catenin-CK1epsilon complex regulates Wnt signaling. J Cell Sci 2010; 123:2621-31; PMID:20940130; http://dx.doi.org/10.1242/jcs.067512
- Kam Y, Quaranta V. Cadherin-bound beta-catenin feeds into the Wnt pathway upon adherens junctions dissociation: evidence for an intersection between beta-catenin pools. PLoS One 2009; 4:e4580; PMID:19238201; http://dx.doi.org/10.1371/journal.pone.0004580
- Monga SP, Mars WM, Pediaditakis P, Bell A, Mule K, Bowen WC, Wang X, Zarnegar R, Michalopoulos GK. Hepatocyte growth factor induces Wnt-independent nuclear translocation of beta-catenin after Met-beta-catenin dissociation in hepatocytes. Cancer Res 2002; 62:2064-71; PMID:11929826
- Tran NL, Adams DG, Vaillancourt RR, Heimark RL. Signal transduction from N-cadherin increases Bcl-2. Regulation of the phosphatidylinositol 3-kinase/Akt pathway by homophilic adhesion and actin cytoskeletal organization. J Biol Chem 2002; 277:32905-14; PMID:12095980; http://dx.doi.org/10.1074/jbc.M200300200
- Li G, Satyamoorthy K, Herlyn M. N-cadherin-mediated intercellular interactions promote survival and migration of melanoma cells. Cancer Res 2001; 61:3819-25; PMID:11325858
- Gartner A, Fornasiero EF, Munck S, Vennekens K, Seuntjens E, Huttner WB, Valtorta F, Dotti CG. N-cadherin specifies first asymmetry in developing neurons. EMBO J 2012; 31:1893-903; PMID:22354041; http://dx.doi.org/10.1038/emboj.2012.41
- He XC, Yin T, Grindley JC, Tian Q, Sato T, Tao WA, Dirisina R, Porter-Westpfahl KS, Hembree M, Johnson T, et al. PTEN-deficient intestinal stem cells initiate intestinal polyposis. Nat Genet 2007; 39:189-98; PMID:17237784; http://dx.doi.org/10.1038/ng1928
- Tian Q, Feetham MC, Tao WA, He XC, Li L, Aebersold R, Hood L. Proteomic analysis identifies that 14-3-3zeta interacts with beta-catenin and facilitates its activation by Akt. Proc Natl Acad Sci U S A 2004; 101:15370-5; PMID:15492215
- Sharma M, Chuang WW, Sun Z. Phosphatidylinositol 3-kinase/Akt stimulates androgen pathway through GSK3beta inhibition and nuclear beta-catenin accumulation. J Biol Chem 2002; 277:30935-41; PMID:12063252; http://dx.doi.org/10.1074/jbc.M201919200
- Hinds JW, Ruffett TL. Cell proliferation in the neural tube: an electron microscopic and golgi analysis in the mouse cerebral vesicle. Z Zellforsch Mikrosk Anat 1971; 115:226-64; PMID:4102323; http://dx.doi.org/10.1007/BF00391127
- Wallingford JB, Mitchell B. Strange as it may seem: the many links between Wnt signaling, planar cell polarity, and cilia. Genes Dev 2011; 25:201-13; PMID:21289065; http://dx.doi.org/10.1101/gad.2008011
- Chalasani K, Brewster RM. N-cadherin-mediated cell adhesion restricts cell proliferation in the dorsal neural tube. Mol Biol Cell 2011; 22:1505-15; PMID:21389116; http://dx.doi.org/10.1091/mbc.E10-08-0675
- Marthiens V, ffrench-Constant C. Adherens junction domains are split by asymmetric division of embryonic neural stem cells. EMBO Rep 2009; 10:515-20; PMID:19373255; http://dx.doi.org/10.1038/embor.2009.36
- Rasin MR, Gazula VR, Breunig JJ, Kwan KY, Johnson MB, Liu-Chen S, Li HS, Jan LY, Jan YN, Rakic P, et al. Numb and Numbl are required for maintenance of cadherin-based adhesion and polarity of neural progenitors. Nat Neurosci 2007; 10:819-27; PMID:17589506; http://dx.doi.org/10.1038/nn1924
- Ivaniutsin U, Chen Y, Mason JO, Price DJ, Pratt T. Adenomatous polyposis coli is required for early events in the normal growth and differentiation of the developing cerebral cortex. Neural Dev 2009; 4:3; PMID:19149881; http://dx.doi.org/10.1186/1749-8104-4-3
- Yokota Y, Kim WY, Chen Y, Wang X, Stanco A, Komuro Y, Snider W, Anton ES. The adenomatous polyposis coli protein is an essential regulator of radial glial polarity and construction of the cerebral cortex. Neuron 2009; 61:42-56; PMID:19146812; http://dx.doi.org/10.1016/j.neuron.2008.10.053
- Hay E, Laplantine E, Geoffroy V, Frain M, Kohler T, Muller R, Marie PJ. N-cadherin interacts with axin and LRP5 to negatively regulate Wnt/beta-catenin signaling, osteoblast function, and bone formation. Mol Cell Biol 2009; 29:953-64; PMID:19075000; http://dx.doi.org/10.1128/MCB.00349-08