ABSTRACT
Annexin A6 (AnxA6) belongs to a highly conserved protein family characterized by their calcium (Ca2+)-dependent binding to phospholipids. Over the years, immunohistochemistry, subcellular fractionations, and live cell microscopy established that AnxA6 is predominantly found at the plasma membrane and endosomal compartments. In these locations, AnxA6 acts as a multifunctional scaffold protein, recruiting signaling proteins, modulating cholesterol and membrane transport and influencing actin dynamics. These activities enable AnxA6 to contribute to the formation of multifactorial protein complexes and membrane domains relevant in signal transduction, cholesterol homeostasis and endo-/exocytic membrane transport. Hence, AnxA6 has been implicated in many biological processes, including cell proliferation, survival, differentiation, inflammation, but also membrane repair and viral infection. More recently, we and others identified roles for AnxA6 in cancer cell migration and invasion. This review will discuss how the multiple scaffold functions may enable AnxA6 to modulate migratory cell behavior in health and disease.
Introduction
Cell migration is fundamental for the organization and maintenance of multicellular organisms. During embryonic development, wound healing or immune responses that protect against pathogens, cells need to be equipped with multifaceted approaches to respond to biochemical signals from their local environment in order to migrate through tissues or across epithelial cell layers. On the other hand, de-regulated and aggressive migratory behavior of cancer cells is a critical feature driving metastatic events.Citation1-4
For cells to undergo migration, a very complex and coordinated set of protein-protein interactions, signaling and membrane trafficking events is required, which has been described in detail in excellent reviews by others.Citation1-4 In brief, the dynamics of cellular movement are initiated by the formation of focal adhesions at the leading edge. This facilitates attachment to the extracellular matrix (ECM) and links the cellular actin cytoskeleton to the ECM. At the leading edge, localized cytoskeletal rearrangements then generate traction forces that allow gradual forward movement. Consequently, for cells to continuously move forward, coordinated assembly and disassembly of adhesions at the cell's front and rear is critical. For cellular motion, heterodimeric integrin receptors composed of α and β subunits are central to focal adhesion structure and function, and their interactions with the ECM trigger both mechanical and chemical signals permitting cells to adhere and migrate. Hence, the transient nature and rapid turnover of focal adhesions requires integrins to be constantly internalized and recycled for redistribution at the leading edge.Citation1-4 This complex interplay of focal adhesion dynamics, integrin trafficking and cytoskeletal re-organisations is organized by a plethora of regulatory membrane transport proteins including Rabs, ADP ribosylation factors (ARFs) and soluble NSF attachment protein (SNAP) receptor (SNARE) proteins, and is coupled to structural elements that link to the actin cytoskeleton, such as vinculin, talin and α-actinin. Moreover, membrane trafficking and cytoskeletal re-arrangements have to be coordinated with the recruitment and spatiotemporal signaling of focal adhesion kinase (FAK), Src, Rho-family GTPases, growth factor receptors, and their numerous regulators and effectors such as protein kinase Cα (PKCα) and p120 GTPase activating protein (p120GAP).Citation1-4
Thus, in these specialized local areas at the leading edge, forward movement requires precise coordination in space and time to enable targeted delivery and endocytosis of vesicles, regulation of actin dynamics and signal complex formation. To facilitate dynamic assembly of specialized membrane domains, proteins that can temporarily stabilize protein–protein or protein-lipid interactions in specific cellular sites are essential. Scaffold, adaptor and targeting proteins fulfil this critical function, as their capacity to simultaneously interact with proteins and lipids is ideal to recruit signaling proteins and their regulators/effectors to specific membrane microdomains. These multiple features provide the platform for targeted vesicle transport from and to the plasma membrane, as well as localized actin re-arrangements, altogether enabling forward movement in a spatiotemporal manner. Over the years, an array of scaffold proteins relevant for cell migration, including paxillin, RACKs, IQGAPs, liprins and caveolin, have been identified.Citation5-8 This implicates that a diverse repertoire of scaffolds orchestrates coordinated dynamics during focal adhesion assembly. Moreover, in disease settings like cancer and wound healing, differential expression patterns of scaffold proteins are now also considered to be conducive or detrimental for the establishment of microenvironments that promote migratory behavior.Citation5-8
Annexins – a conserved family of proteins with diverse scaffolding functions
Annexins represent another protein family with various scaffolding functions important for cell motility. Like many other scaffolds, the modular domain organization of annexins supports protein–protein and protein–lipid interactions.Citation8-11 Each annexin contains a distinctive leader sequence at the N-terminus, followed by four, eight for AnxA6, conserved repeats that build the C-terminal annexin core, containing Ca2+ and phospholipid binding sites. Upon Ca2+ elevation, these features permit their rapid translocation from the cytosol to the plasma membrane or intracellular membranes.Citation8-11 In fact, the transient nature of Ca2+ spikes inside cells facilitates reversible membrane binding behavior of annexins, making them ideal to create short-lived platforms and protein complexes for the continuous and localized assembly and disassembly of specialized structures required for forward movement.
Most annexins are widely expressed, often at high levels, in multiple locations and with various interaction partners. Despite these intricate characteristics, annexins are now recognized to alter the localization and function of signaling proteins, to create specific membrane microenvironments, and trigger cytoskeletal re-arrangements.Citation8-13 For more than 20 years, several annexins have been known to influence the adhesive and migratory behavior of cells. For instance, early studies implicated AnxA1 to control the adhesion and migration of leucocytes, such as neutrophils and granulocytes, during the inflammatory response induced by glucocorticoids.Citation14,15 Soon thereafter, AnxA2 was reported to affect the migratory behavior of endothelial cells and neoangiogenesis.Citation16,17 Similarly, recombinant AnxA5 was initially shown to inhibit lung carcinoma cell migration and T cell adhesion.Citation18,19 Strikingly, all these annexins contribute to cell adhesion and migration through different mechanisms. This includes prominent extracellular functions that do not require Ca2+-dependent membrane association. In fact, in vivo studies from AnxA1, A2 and A5-deficient mouse models strongly support these extracellular activities to significantly affect adhesive and migratory cell behavior, which has been reviewed in detail elsewhere.Citation20-23
For each of the abovementioned annexins (AnxA1, A2, A5), abundant and rapidly expanding literature related to cell adhesion and migration exists, while little is known about the possible roles for AnxA6 in this context. However, recent findings from our laboratory and others provide exciting insights how AnxA6 may influence the ability of cells to migrate and adhere. In the following we will summarize and discuss the multiple functions that enable AnxA6 to modulate cell migration and adhesion, with possible implications for metastatic events in cancer.
Extracellular roles of AnxA6 in cell migration
AnxA6 represents the largest member of the annexin family and is highly expressed in most tissues.Citation8-11,24,25 AnxA6 is predominantly located at the plasma membrane and the endosomal compartment, where it interacts with multiple proteins and lipids to organize membrane domains, signaling complexes, transient membrane-actin interactions as well as intracellular cholesterol homeostasis.Citation8,9,24-28 All of these interactions and regulatory functions appear relevant for cell proliferation, survival, differentiation, inflammation, membrane repair and viral infection, which has been reviewed in detail.Citation8-11,23-25
More recently, we and others identified some of these intracellular AnxA6 functions to contribute significantly to the migratory and adhesive behavior of fibroblasts and several cancer cell linesCitation29,30 Yet, the first reports of AnxA6 affecting cell adhesion and migration came from studies implicating extracellular AnxA6 activities. When characterizing the molecular properties of metastatic cells, AnxA6 and other annexins were identified on the cell surface of metastatic RAW117 lymphoma cells.Citation31 Anti-AnxA6 antibodies partially blocked Ca2+ -dependent adhesion of these cells to endothelial layers, indicating that AnxA6 was a cell surface component that could possibly function as an adhesion molecule on migrating cancer cells.Citation31 Soon thereafter, purified AnxA6 as well as other annexins were reported to bind glycosaminoglycans, such as chondroitin sulfate, heparin and heparin sulfate,Citation32,33 which are ECM based molecules believed to contribute to adhesion of migrating cells. Moreover, ectopic expression of AnxA6 in A431 cells, an epithelial adenocarcinoma cell line which lacks endogenous AnxA6,Citation26,34 conferred the ability to attach to chondroitin sulfate chains.Citation33 Flow cytometry analysis indicated that a certain pool of AnxA6 proteins could indeed be exposed on the external cell surface of A431 cells.Citation33 More recently, AnxA6 knockdown in triple-negative BT-549 breast cancer cells, lacking estrogen (ER) and progesterone receptors as well as ErbB2, reduced cell adhesion to fetuin-A, a prominent factor in serum considered to promote cell attachment of tumor cells.Citation35 The interaction of cells with immobilized fetuin-A seems to be mediated by cell surface annexins, including AnxA6 and AnxA2.Citation35-37 In follow-up studies, Sakwe and colleagues examined the migratory behavior of AnxA6-depleted BT-549 cells.Citation30 AnxA6 depletion in these cells was associated with increased cell proliferation, which was in line with tumor suppressor activities of AnxA6 in epidermal growth factor receptor (EGFR) overexpressing A431, ER-negative breast cancer cells (BCC), head and neck squamous cell carcinoma lines (HNSCC), but also commonly used HeLa and HEK293 cell lines with moderate EGFR levels.Citation28,38 In addition, AnxA6-depleted BT-549 showed normal adhesion to fibronectin (FN) and laminin, but cell spreading on fetuin-A coated surfaces was strongly inhibited. The latter correlated with reduced motility and invasiveness in vitro, altered vinculin staining and FAK signaling, indicating compromised focal adhesion assembly on fetuin-A coated surfaces.Citation30 Altogether these studies suggest that a pool of extracellular AnxA6 protein may bind to several proteoglycans or other proteins like fetuin-A to support the adhesive and migratory properties of cancer cells ().
Table 1. AnxA6 interactions relevant in cell migration and adhesion.
It is yet unclear if these interactions of AnxA6 on outer cell surface membranes are relevant for in vivo cell attachment, where enrichment of ECM proteins like FN, laminin, and collagen most likely favor integrin-mediated adhesion. Annexins are generally considered intracellular proteins and do not contain signal sequences that facilitate secretion. However, substantial amounts of some annexins, including AnxA1, AnxA2 and AnxA5, have been found outside cells, with significant in vivo evidence for their extracellular activities in adhesion, migration and various other biological processes.Citation19-22 The trafficking routes enabling these annexins to exit cells are not fully understood and several non-conventional secretion pathways have been discussed.Citation10,11,20-23 In contrast to the aforementioned annexins, very few studies have implicated extracellular AnxA6 locations. Probably unrelated to cell migration, AnxA6 was identified as a cell surface receptor for β-glucuronidase on liver membranes.Citation39 In ER-positive and ErbB2-amplified SKBR3 breast cancer cells, Ca2+ influx coupled to the interaction of AnxA6 with S100A8 and S100A9 proteins may facilitate translocation of AnxA6 from the inner to the outer leaflet of the plasma membrane, and is possibly relevant in neutrophil chemotaxis and adhesion as well as inflammation-related cancers.Citation40 Alternatively, in BT-549 breast cancer cells, it was proposed that AnxA6 and other annexins are secreted into exosomes via lysosomal exocytosis to contribute to focal adhesion organization and invasiveness (see above).Citation30
Indeed, exosomes carry a diverse set of bioactive molecules that can promote cell adhesion and motilityCitation41 and within those from smooth muscle cells, AnxA6 was also identified.Citation42 In fact, in the publicly available ExoCarta exosome database (www.exocarta.org), several annexins, including AnxA6, belong to the top 100 proteins often identified in exosomes. The mechanisms that could translocate AnxA6 into the lumen of exosomes are still unclear, but AnxA6 and several other annexins contain a KFERQ-like motif that facilitates chaperone-mediated autophagy, thereby translocating cytosolic proteins inside late endosomal/(pre-)lysosomal vesicles.Citation43 In fact, secretion of proteins via exosomes starts by accumulation in this compartment, followed by their transport and fusion with the plasma membrane. Autophagy and exosomes are increasingly recognized as determining factors for integrin trafficking and focal adhesion turnover, promoting cancer cell motility.Citation41,44,45 Most excitingly, recent proteomic studies on the signature of exosomes from cancer-associated fibroblasts identified AnxA6 complexed with LDL receptor-related protein 1 and thrombospondin-1 to support pancreatic cancer aggressiveness ().Citation46 These findings suggest that cellular export of AnxA6 through this route contributes to affect migratory cell behavior. Moreover, AnxA6 containing exosomes were restricted to serum from pancreatic ductal adenocarcinoma (PDA) patients, providing potential for AnxA6 to become a therapeutic target and marker for PDA grade.Citation46
Additionally, in a number of cell and animal models, AnxA6 and several other annexins were shown to be vital for membrane repair.Citation47-51 In zebrafish and live muscle fibers from mice, AnxA6 is rapidly recruited to damaged sarcolemma to contribute to the formation of a tight cap over a vesicle-rich repair zone.Citation47-49 In addition to membrane repair in muscle, AnxA6 also contributes to restore membrane lesions induced by bacterial pore-forming toxins.Citation50,51 It is tempting to speculate that during sarcolemma cap formation, outward vesiculation or shedding for the removal of toxin-induced poresCitation47-51 some AnxA6 proteins might ‘leak’ into the extracellular space. Although in vivo evidence for extracellular AnxA6 activity has yet to be provided, these opportunities for protein leakage into the extracellular milieu may transiently create AnxA6-enriched local microenvironments with consequences for cell adhesion and migration (). In fact, elevated AnxA6 levels in patient serum has recently been proposed as a marker for esophageal adenocarcinoma, an aggressive cancer type.Citation52
Annexin A6 and remodeling of plasma membrane domains
At the plasma membrane, the spatial organization of specific proteins and lipids into discrete regions creates microdomains with distinct signaling functions. This includes focal adhesions at the leading edge, where the recruitment and activation of integrins has been linked to cholesterol-rich membrane domains (lipid rafts).Citation5,6,53 In line with a function in microdomain formation, relevant for focal adhesions, AnxA6 binds cholesterol-enriched (ordered) membranes in vitro, translocates to cholesterol-rich and detergent-resistant membranes (lipid rafts) upon Ca2+ elevation and is enriched in cholesterol-rich caveolae, a specialized type of raft ().Citation54 In particular caveolae and its structural protein caveolin have been associated with directional migration and invasion, coordination of Rac/Rho activation, focal adhesion turnover, and enhanced metastatic potential in advanced stages of some cancers.Citation55-58 On the other hand, AnxA6 also localizes to non-raft (fluid) domains rich in negatively charged phospholipids such as clathrin-coated pits ().Citation59 These structures facilitate endocytosis of growth factor receptors such as the EGFR, which signals from endosomal compartments to accelerate wound re-epithelialisation, and promote cell migration and angiogenesis.Citation9,60 In addition, integrins are also endocytosed via clathrin-coated pits, which is critical for integrin recycling and focal adhesion turnover during directional migration.Citation2,3,61 Yet, although the membrane-binding properties of annexins had long been proposed to organize membrane domains, evidence from live cells that AnxA6 or any other annexin could alter microdomain properties, was missing.
We recently addressed this critical issue in the annexin field by labeling cells with the fluorescent probes di-4-ANEPPDHQ and laurdan, which enables visualization of liquid-ordered (cholesterol-rich, raft-like) and liquid-disordered (cholesterol-poor, non-raft) microdomains at the plasma membrane.Citation54 This approach enabled comparison of membrane order in AnxA6-deficient and AnxA6 expressing A431 cellsCitation26,34 as well as mesenchymal mouse embryonic fibroblasts from wild-type and AnxA6 knockout mice.Citation62 Strikingly, significant changes in membrane order, reflecting lipid re-distributions at the plasma membrane, and increased sensitivity of membrane order toward cholesterol-depleting agents were observed in cells with elevated AnxA6 levels.Citation54 Likewise, analysis of laurdan-stained T cells isolated from WT and AnxA6−/− mice supported AnxA6 to alter the membrane environment in the plasma membrane.Citation63 From these observations we predicted consequences in the distribution of cell surface proteins. Indeed, PALM super-resolution microscopy identified association of AnxA6 up/downregulation with differential and cell-specific differences in the cholesterol-sensitive microdomain distribution of membrane-anchored, fluorescent raft and non-raft marker proteins.Citation54
In spite of this, what are the underlying mechanisms that enable AnxA6 to alter microdomain formation? The well-established Ca2+-inducible AnxA6 translocation to the plasma membrane is probably one critical aspect to alter phospholipid and cholesterol distribution and organization. Here, AnxA6 preferentially binds negatively charged phospholipids (phosphatidylserine, phosphatidylinositol, phosphatidic acid), and has some affinity toward phosphatidylethanolamine and arachidonic acid.Citation24,25 In addition, acidic pH and Ca2+-independent affinity to cholesterol-rich membranes also contributes to AnxA6 membrane association (see above).Citation54,64,65 Although limited resolution of the overlay of AnxA6 immunostaining with laurdan staining did not yet enable us to identify a preference of AnxA6 translocation to raft or non-raft microdomains at the plasma membrane (Grewal, Rentero and Enrich, unpublished), it is likely that the recruitment of AnxA6 to specific sites will be determined by the differential affinity toward these various lipids, localized changes in Ca2+ and pH, as well as membrane-bound protein binding partners (see below). This could possibly stabilize microdomains for transient signaling events. In turn, once recruited to the membrane, pools of AnxA6 in specialized domains, for example focal adhesions, seem to contribute to induce lipid re-distributions and recruit signaling proteins (see below) to create microenvironments that allow signal transduction and actin-re-arrangements for dynamic focal adhesion assembly and disassembly.
Annexin A6 - actin interactions contributing to domain formation
Besides re-organization of membrane lipids, focal adhesion dynamics are intimately linked to actin re-arrangements as the anchoring of actin filaments is critical for localized transduction of mechanical forces across the plasma membrane for forward movement. In this scenario, bridging molecules like talins and kindlins bind to integrins, and recruit cytoskeletal and signaling proteins.Citation66 However, knowledge on proteins that stabilize the association of these multifactorial focal adhesion protein complexes and actin filaments to lipids in membranes is still limited. Hence, the ability of AnxA6 to interact with cytoskeletal proteins has to be considered (). Indeed, AnxA6 binds actin filaments in a Ca2+-dependent mannerCitation12,13,25,67 interacts with α-actinin,Citation68 and co-localizes with F-actin at protrusions of the plasma membrane, including membrane ruffles,Citation25,67,69 and circular-dorsal ruffles (Reverter and Enrich, unpublished), that are essential for directed cell motility, cell spreading and adhesion.Citation70
Ca2+-dependent binding of AnxA6 to pointed ends of actin filaments and α-actinin could contribute to form reversible, membrane-cytoskeleton complexes.Citation67-69,71 In cells labeled with laurdan, membrane order of AnxA6 expressing cells showed an increased sensitivity toward actin depolymerizing agents.Citation54 Moreover, we observed F-actin accumulation at the plasma membrane upon expression of membrane-anchored AnxA6.Citation72 These striking reorganizations of cortical actin correlated with a role for AnxA6 in the maintenance of intracellular Ca2+ homeostasis via actin-dependent regulation of Ca2+ entry at the cell surface, with consequences for cell proliferation.Citation72 Given that lower Ca2+ levels at the leading edge are required for cell migrationCitation73 and the fact that AnxA6 interacts with other Ca2+ channels, including two pore channel 1/2 and ryanodine receptors in late endosomes (LE) and the endoplasmic reticulum,Citation74,75 one can contemplate that besides direct interactions of AnxA6 with cytoskeletal proteins, the ability of AnxA6 to modulate Ca2+ influx and homeostasis participates in regulating cytoskeletal re-arrangements in cell migration ().
In addition, upon Ca2+-mobilization, AnxA6 interacts with spectrin to trigger the recruitment of calpain I, which cleaves the spectrin-actin cytoskeleton, allowing the entry of endocytic vesicles at the plasma membrane.Citation59 Interestingly, calpain is a ubiquitous molecule and alternative mechanisms of calpain activation, including Ca2+ elevation, have also been proposed. Moreover, dissection of calpain-mediated proteolysis identified many targets in adhesion complexes, including talin, paxilin, α-actinin, ezrin, FAK and the cytosolic tails of β1 and β3 integrins. Additionally, calpain-mediated cleavage of ezrin is critical to limit fibroplasia late in the regenerative phase of skin repair,Citation76,77 Altogether, these findings intimately link calpains with the integrin signal transduction machinery driving cell motility.Citation76,77
In support of AnxA6 being involved in the generation of endocytic vesicles, possibly through spectrin/calpain-dependent mechanismsCitation59,78 AnxA6 stimulated vesicle budding in vitro,Citation79 and bound to the μ-subunits of the clathrin adaptor complex AP2 in a Ca2+-dependent manner.Citation80 These findings correlate with spectrin modulating integrin motility at the cell surface for adhesion and ECM degradation. In addition, loss of spectrin impairs cell adhesion and spreading, alters actin organization and focal adhesions, and modifies expression of integrins.Citation81,82 In line with these findings, the selective endocytosis of α integrins appears to be driven by their interaction with AP2.Citation83 Hence, AnxA6-mediated re-organization of the spectrin cytoskeleton might contribute to allow the internalization and recycling of integrins, but also growth factor receptors, signaling proteins like Src and FAK at the plasma membrane for focal adhesion turnover. One can even speculate that through its transient membrane binding behavior, AnxA6 may provide a platform for simultaneous, dynamic and reversible interactions with both actin and spectrin at the plasma membrane ().
AnxA6 and recruitment of signaling proteins
In addition to AnxA6 re-organizing membrane lipids and possibly serving as a membrane anchor for the actin cytoskeleton to transiently create and stabilize membrane microenvironments, a major scaffold function of AnxA6 is to facilitate localized recruitment of signaling proteins. AnxA6 binds to a panel of signaling proteinsCitation8,9,24,25 and some of those interaction partners have prominent roles in cancer cell migration (). In particular, AnxA6 interacts constitutively with PKCα and p120GAP in the cytosol, both negative regulators of the EGFR and Ras/mitogen activated protein kinase (MAPK) pathway.Citation24-26,28,38 Upon EGFR activation, AnxA6 promotes the Ca2+-dependent membrane recruitment of PKCα and p120GAP, leading to increased EGFR/PKCα and H-Ras/p120GAP assembly and consequently, EGFR and H-Ras inactivation. At the plasma membrane, AnxA6 probably stabilizes EGFR/PKCα and p120GAP/H-Ras complexes, as AnxA6 co-precipitates with EGFR in a Ca2+-inducible fashion and interacts with active (H-Ras-GTP), but not inactive H-Ras (H-Ras-GDP), respectively.Citation24-26,28,38 Together with AnxA6 co-purifying with Raf-1,Citation84 the scaffold function of AnxA6 likely involves multiple simultaneous protein–protein interactions, stabilizing compartmentalized multifactorial protein complexes that ensure EGFR and Ras signal termination.
In our previous studies, we solely investigated these interactions in the context of cancer cell growth. Indeed, we observed anti-proliferative properties of AnxA6 via PKCα- and p120GAP-mediated EGFR and H-Ras downregulation in EGFR overexpressing A431, BCCs and HNSCCs as well as ER-negative BCCs.Citation24-26,28,38 This is supported by earlier reports from Moss and coworkersCitation85 and our unpublished data showing reduced xenograft growth of A431 cells ectopically expressing AnxA6. Vice versa, increased growth of MDA-MB-231 and HeLa cell lines upon AnxA6 depletion was observed.Citation28,38 Altogether, these findings implicate AnxA6 as a tumor suppressor to inhibit growth of cancer cells through EGFR and Ras inactivation.Citation24,25 However, there is also substantial literature that points to the prominent crosstalk of de-regulated EGFR and Ras activity with integrin, FAK, Src, Rac/Rho GTPases signaling in cancer cell metastasis. It would go beyond the scope of this article to describe these complex networks in more detail and we recommend excellent review articles from others.Citation3-5,86-90 In addition, AnxA6 has also been described to interact with Pyk2, and the Src kinases Lck and FynCitation9,25,91,92 all of which with established links to migratory events in cancer cells.Citation87 Indeed, AnxA6 overexpression in A431 and Chinese hamster ovary (CHO) cells reduced migration in scratch- and transwell assays, which correlated with reduced invasion in 3D organotypic assays () and strongly reduced activation of FAK and Src.Citation29 In line with this, AnxA6 depletion in HeLa and MDA-MB-231 cells increased. wound closure and transwell migration/invasion.Citation29 Moreover, in wound-healing assays, upregulated AnxA6 levels in A431 cells were associated with increased potency of tyrosine kinase inhibitors targeting EGFR, including gefitinib and erlotinib, to inhibit cell migration (Hoque and Grewal, unpublished). It remains to be determined if these observations are also relevant in vivo or in other metastatic cancer models, where oncogenic EGFR and Ras activity are common and AnxA6 downregulation has been observed.Citation9,28,93
Figure 1. A 3-dimensional organotypic invasion assay highlighting the reduced invasiveness of AnxA6 expressing A431 cells. (A) The scheme shows an organotypic invasion assay for the three-dimensional assessment of tumor cell behavior. Primary human fibroblasts are embedded in a 3D collagen-I matrix, prepared from acid extracted rat tail collagen. Detached, polymerized matrix is allowed to contract for 12 d in complete media, until embedded fibroblasts have contracted the matrix to ∼1 cm in diameter. This forms a matrix with high in vivo fidelity. Tumor cells are then plated on top of the matrix in complete media and allowed to grow to confluence for ∼3–5 d. The matrix is then mounted on a metal grid, raising it to an air/liquid interface, which results in a chemoattractive gradient feeding the matrix from below. Media is changed every 2–3 days, and after 8–21 days, cultures can be fixed, processed (e.g. hematoxylin and eosin staining) and analyzed.Citation29,114,116,139 (B) Representative cutout images of AnxA6-deficient A431 wild-type cells (A431-WT) and AnxA6 expressing A431 cells (A431-A6) seeded on 3D-matrices and allowed to invade over an air-liquid interface for 12 days, prior to fixation and hematoxylin and eosin staining. The direction of invasion is indicated. Scale bars represent 100 μm. The reduced ability of A431-A6 cells to invade the matrix is apparent (for quantification see also ref. Citation29). (C) Representative images of whole matrix sections, demonstrating reduced invasion of AnxA6 expressing A431 cells. The enlarged sections shown in B are indicated. Scale bars represent 1 mm.
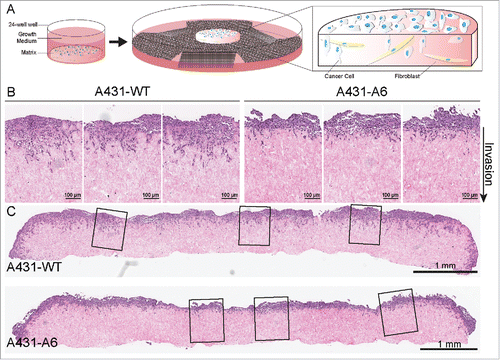
Given that signaling proteins at the plasma membrane are not randomly distributed, but targeted and localized to specific microdomains, AnxA6 may promote PKCα- and p120GAP-mediated EGFR/Ras inactivation at specialized sites critical for cell motility. In this context, the spatial distribution of Ras isoforms, small GTPases that oscillate between an active GTP-bound and inactive GDP-bound form, has been studied intensively.Citation94 Active and inactive Ras proteins are organized in nanoclusters, containing 6–7 Ras proteins per nanocluster, creating the signaling platforms essential for the recruitment of Ras regulators and effectors. The distribution and lateral movement of active and inactive Ras proteins in the plasma membrane is modulated by GTP/GDP exchange, thus depends on the activity of guanine nucleotide exchange factors and GAPs. In addition, interaction of membrane lipids with Ras proteins is critical for cluster formation. While H-Ras GDP clusters are mainly found in cholesterol-rich domains, H-Ras-GTP nanoclusters are associated with disordered and cholesterol-independent domains.Citation94 Hence, the distribution of cholesterol at the plasma membrane is highly relevant for the recruitment of H-Ras regulators such as p120GAP, but possibly also for membrane translocation of PKC to phosphorylate and regulate K-Ras activityCitation95 and consequently for signal termination. In addition, a distinct cohort of phospholipids, in particular phosphatidylserine, but also phosphatidic acid and phoshatidylinositides contribute to the spatial segregation of Ras isoforms. In line with the constant re-organization of membrane domains at the leading edge, the GDP/GTP cycle of Ras proteins ensures the transient nature of Ras nanoclusters. Interestingly, single molecule image analysis revealed that once recruited to the plasma membrane, the Ras binding domain of p120GAP became transiently immobile to interact with H- and K-Ras-GTP.Citation96 Hence, the binding affinity of AnxA6 toward the abovementioned phospholipids, but also cholesterol, might stabilize the recruitment and ‘immobilize’ p120GAP to active Ras-GTP clusters.
The ability of AnxA6 to act as a membrane targeting protein for p120GAP and being relevant for cell migration may indeed go beyond Ras inactivation. For instance, p120GAP interaction with p190-RhoGAP, FAK, the calpain subunit Capns1 and even integrins has been implicated in the regulation of Rho GTPase activity, Rho-mediated cytoskeletal rearrangements and integrin trafficking.Citation93,97-103 Thus, AnxA6-mediated recruitment of p120GAP could coordinate pathways to promote actin remodeling for the formation of focal adhesions, establishment of cell polarity, as well as integrin recycling.Citation93,97-103
In contrast to the advanced knowledge on Ras signaling platforms, the regulation of EGFR distribution at the cell surface is less clear, with substantial amounts of EGFR being found in non-raft domains, but also in cholesterol-rich microdomains.Citation9,104 These possibly distinct pools of EGFR seem to provide opportunity for signal diversity, with substantial cross-talk between EGFR, integrins, FAK, Src, and the actin cytoskeleton to integrate the signals required for cell migration.Citation9,86-90,104 Possibly AnxA6-mediated stabilization of EGFR/PKCα containing signal complexes, followed by EGFR inactivation contributes to downregulate EGFR-mediated signaling networks required for focal adhesion turnover during forward cellular movement.
Based on the development of PKCα-dependent signaling pathways upon AnxA6 overexpression in cells that do not express EGFR,Citation105 AnxA6-mediated membrane targeting of PKCα may also be relevant in migratory cell behavior unrelated to EGFR. Many studies have implicated stimulatory roles for PKCα in cancer cell motility and tumor cell invasion and metastasis. For example, PKCα is recruited to cholesterol-rich domains as well as focal adhesions in a Ca2+-dependent manner to associate with β1-integrins and control integrin trafficking, focal adhesion turnover for cell spreading and polarized cell trafficking.Citation106-109 PKCα also phosphorylates ezrin and thereby contributes to the accelerated motility of cancer cells.Citation110
Taken together, several cellular activities listed above implicate PKCα, but also p120GAP, to stimulate cell migration. Thus, the proposed model of AnxA6 inhibiting migratory behavior via PKCα and p120GAP-mediated EGFR/Ras inactivation may only be relevant in certain susceptible cell types with high AnxA6 levels, or in the context of co-operating genetic lesions such as EGFR overexpression. Indeed, in BT-549 cells, which express only moderate levels of AnxA6 and EGFR,Citation28 AnxA6 depletion promoted EGFR degradation, attenuated MAPK signaling, reduced cell motility and invasiveness and sensitized BT-549 cells to EGFR tyrosine kinase inhibitors.Citation30,111 Likewise, although our recent studies suggested that the absence of AnxA6 in T-lymphocytes destabilized the organization of the plasma membrane, T-cell migration and signaling was not affected in AnxA6-deficient T-lymphocytes.Citation63 Also, in chick midbrain neural crest cell development, AnxA6 depletion attenuated, while AnxA6 overexpression enhanced neural crest cell emigration, respectively.Citation112 However, opposing cellular activities as observed for AnxA6 are not uncommon, as loss of another scaffold protein, caveolin-1 (Cav-1), predicts poor clinical outcome in some cancers, while its overexpression is linked to aggressive and metastatic behavior in others.Citation55-58 Interestingly, as discussed below, the subcellular localization and ability of Cav-1 to form caveolae is strongly influenced by high/low AnxA6 expression levels in EGFR overexpressing A431, MDA-MB-468 and ER-negative MDA-MB-436 cells,Citation27 yet if these regulatory networks operate in other cells and tissues to possibly modulate cell migration has still to be determined.
The regulatory role AnxA6 in cholesterol homeostasis determines migratory behavior
As outlined above, the function of cholesterol as a membrane constituent comprises several decisive features relevant for the spatiotemporal coordination of signaling and trafficking events during cellular movement. As such, cholesterol depletion at the cell surface disrupts the integrity and dynamics of focal adhesion (dis-) assembly, caveolae formation, the recruitment and recycling of integrins, FN-dependent cell migration, and Rac/Rho GTPase activation.Citation1-5,53-58,113,114 Despite these observations, the cellular cholesterol pools delivered into focal adhesions and linking cholesterol metabolism with migratory behavior are not fully known. Rather than synthesizing cholesterol de novo, cells generally acquire cholesterol through low density lipoprotein (LDL) receptor-mediated endocytosis.Citation114,115 Internalized and esterified LDL-cholesterol finally reaches LE/(pre-) lysosomes, where LDL-derived cholesteryl esters are hydrolyzed. LE are the major sorting station for LDL-cholesterol, which is mainly delivered to the plasma membrane, or transported to the endoplasmic reticulum for feedback control or stored as cholesteryl esters in lipid droplets. Using Niemann Pick Type C1 (NPC1) mutant cells, which accumulate cholesterol in LE, we and others recently demonstrated that LE-cholesterol is crucial for cancer cell migration.Citation116,117 The underlying mechanisms involve compromised LE-cholesterol delivery to the plasma membrane, trans-Golgi network and recycling endosomes, as reduced cholesterol levels in these compartments interfered with focal adhesion and caveolae numbers, FN secretion and integrin recycling, with drastic effects on migratory cell behavior.Citation116-119
Most strikingly, in addition to AnxA6 directly affecting membrane organization, the actin cytoskeleton and recruitment of signaling proteins at the plasma membrane, we recently identified AnxA6 to indirectly regulate migratory behavior through inhibition of LE-cholesterol distribution ().Citation29 Using LDL or the amphipatic steroid U18666A, we initially identified translocation of AnxA6 proteins to cholesterol-enriched LE.Citation65,120 Moreover, elevated AnxA6 levels led to an NPC1-like phenotype, characterized by LE-cholesterol accumulation and a concomitant diminution of cholesterol at the plasma membrane, Golgi apparatus, and recycling endosomes.Citation24,25,27 In several studies we demonstrated that the cholesterol imbalance induced by AnxA6 (i) perturbed cholesterol-dependent Golgi recruitment of cytosolic phospholipase A2 to drive caveolin transport to the cell surfaceCitation27,118 but also led to mislocalization of several SNARE proteins: (ii) soluble N-ethylmaleimide-sensitive fusion protein 23 (SNAP23), syntaxin 4 (Stx4),Citation119 and (iii) Stx6,Citation116 all of which with critical roles in cell migration (see and below).
First, AnxA6-mediated inhibition of Cav-1 export from the Golgi significantly reduced the numbers of caveolae at the cell surface.Citation27 In fact, high/low AnxA6 levels inversely correlated with caveolae numbers in A431 cells and several BCCs.Citation27 Hence, AnxA6 expression levels could be one of the factors determining the ambivalent roles of Cav-1 in cancer progression. Cav-1 promotes migration in a number of tumor-derived cell lines, yet has also been identified to inhibit cancer cell migration.Citation55-58 These opposite activities may reflect differential cross-talk of Cav-1 with various members of the cellular networks at the cell surface that drive migration and invasion including growth factor receptors, FAK, Src, and Rac/Rho GTPases. Hence, differential AnxA6 levels in various cancers and its impact on the cholesterol-dependent Cav-1 cell surface delivery could explain some of the diversity observed for Cav-1 modulating signaling events relevant for cancer cell migration and invasion.Citation55-58
Second, high AnxA6 levels interfered with the clustering of SNAREs Stx4 and SNAP23 in cholesterol-rich domains at the cell surface.Citation119 These widely expressed t-SNAREs normally enable docking of secretory vesicles at the cell surface, but accumulated on Golgi membranes of AnxA6 expressing cells. Consequently, Stx4/SNAP23-dependent FN secretion was strongly inhibited in AnxA6 expressing cells and other NPC1-mutant models.Citation119 This LE-cholesterol related AnxA6 attribute could counteract the well-established fact that increased secretion of ECM proteins such as FN strongly support the signaling cascades that promote migration in oncogenic settings.Citation121 In addition, these findings are in line with earlier studies that suggested an inhibitory role for AnxA6 in the secretory pathway.Citation122-124 Thus, AnxA6-mediated inhibition of LE-cholesterol egress is likely to also affect other Stx4/SNAP23-dependent trafficking events often de-regulated in cancer cell migration, including α5 integrin recycling, Src and FAK activation at focal adhesions, and membrane type-1 matrix metalloproteinase delivery to invadopodia.Citation125
Third, the most compelling evidence that AnxA6 inhibits cell migration via the establishment of a cellular cholesterol imbalance came from our recent studies that identified AnxA6 overexpression to interfere with Stx6 localization and function,Citation29 a SNARE protein essential for the cell surface delivery of integrins, FAK signaling in focal adhesions, and directional migration toward FN.Citation126,127 Strikingly, elevated AnxA6 levels caused Stx6 and integrin accumulation in recycling endosomes ()Citation29 leading to decreased cell surface expression of αVβ3 and α5β1 integrins, reduced cell migration in wound healing, trans-well and 3D-migration/invasion assays ().Citation29 In line with these findings, individual cell tracking and cell spreading in AnxA6 expressing CHO and A431 cells was inhibited in a Stx6- and NPC1-dependent manner (). Several studies identified Stx6 to be upregulated in breast, liver, and prostate cancers,Citation127 and Stx6 elevation to correlate with metastatic esophageal squamous cell carcinoma.Citation128 With Stx6 overexpression promoting cell migration,Citation127 it remains to be determined if AnxA6 upregulation in these cancers could downregulate oncogenic roles of Stx6. Also, based on the findings described above, AnxA6 upregulation may not only affect Stx4/SNAP23 and Stx6, but also localization and functioning of other cholesterol-sensitive SNAREs.Citation129,130 This includes VAMP2, which participates in α5, α3, β1 integrins and FAK traffickingCitation125 as well as Stx12-dependent Src and β1 integrin transport to cholesterol-rich invadopodia for cell invasion.Citation131
Figure 2. Reduced cell surface expression of α5 integrins in AnxA6 overexpressing CHO cells. (A) Chinese hamster ovary wild-type (CHO-WT) and Annexin A6 overexpressing CHO cells (CHO-A6) were grown on coverslips, fixed with 4% paraformaldehyde and immunolabeled with anti-α5 integrin as described.Citation29,116 Arrowheads in control CHO-WT cells show strong staining of α5 integrins at the plasma membrane. Arrows point at the compact staining of α5 integrins in intracellular compartments, resembling recycling endosomes in CHO-A6 cells (for a more detailed characterization of α5 integrin distribution in CHO-WT and CHO-A6 cells see ref. Citation29). Scale bar is 10 µm.
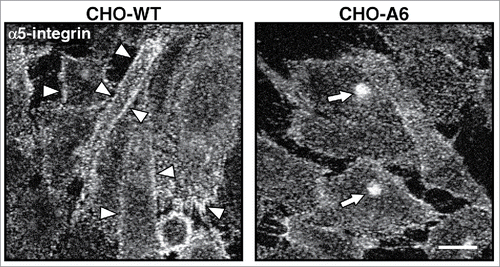
Figure 3. AnxA6 overexpression reduces cell velocity and spreading. (A) CHO-WT, CHO-A6, CHO-WT expressing scrambled or Stx6-targeting siRNA and (B) A431-WT and A431-A6 cells were plated on fibronectin and movement of cells was recorded by time-lapse video microscopy for 12 h. Individual cell migration tracks were marked and the speed (velocity) ± U18666A (U18), overexpression of wild-type NPC1 in CHO-WT, or dominant-negative NPC1-P692S mutant, which cannot bind cholesterolCitation29,116 in CHO-A6 was recorded. (C) CHO-WT and CHO-A6 cells plated onto fibronectin were stained for F-actin after 2 hours. The spreading area (μmCitation2) ± U18666A, overexpression of wildtype NPC1 in CHO-WT, or dominant-negative NPC1-P692S mutant in CHO-A6 was determined. Representative fields of both cell lines are shown. 100 cells per experiment were quantified. Scale bar is 10 µm. *, p < 0.05; **, p < 0.01; ***, p < 0.001. Error bars shown in this figure represent SD.
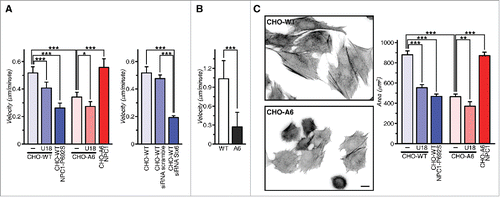
In strong support of elevated AnxA6 levels interfering with Cav-1, SNAP23/Stx4 and Stx6 functions via perturbed LE-cholesterol transport routes, ectopic NPC1 expression in AnxA6 expressing cells diminished LE-cholesterol accumulation, but also restored cellular distribution of Cav-1, SNAP23/Stx4 and Stx6, with a concomitant elevation of cell surface integrins.Citation27,29,119 Despite this approach rescuing the NPC1-mutant like phenotype of AnxA6 expressing cells, and also restoring cell velocity and spreading (), it remains to be identified how AnxA6 interferes with LE-cholesterol export. AnxA6 binds to cholesterol-rich membranes in vitro and cell cultureCitation25,54 and a tryptophan residue (W343) within the AnxA6 linker region was proposed important for AnxA6-cholesterol interactions.Citation132 More recently, proteome-wide mapping also identified AnxA6 as a potential cholesterol-binding protein.Citation129 These findings might reflect AnxA6 affinity to cholesterol-rich rafts and/or caveolae observed in many studies,Citation24,25,54 and earlier work from our laboratory, which identified AnxA6 recruitment to cholesterol-enriched LE.Citation65,120 We initially proposed that AnxA6 interfered with NPC1 activityCitation27 but several other cholesterol export pathways from this compartment exist,Citation114,115,130 and recent work from our laboratories identified that AnxA6 interacts with a member of the large Tre-2/Bub2/Cdc16 protein family, TBC1D15, a Rab7-GTPase activating protein (Enrich, Grewal and Rentero, unpublished). Moreover, preliminary studies suggest that AnxA6 recruits TBC1D15 to LE, which inhibits Rab7 GTPase activity and its ability to promote LE-cholesterol export (Enrich, Grewal, and Rentero, unpublished). These findings link AnxA6 and LE-cholesterol transport with increasing evidence that associate this member of the Rab protein family with actin-dependent cell migration and metastasis,Citation133-135 as well as the translocation of integrins, Src kinase and metalloproteases from LE to focal adhesions.Citation136-139
Conclusion
In summary, multiple avenues for AnxA6 being involved in cell motility have emerged and have been listed in : (1) Interaction of extracellular AnxA6 proteins with ECM proteins may affect adhesive and migratory cell properties; (2) AnxA6 translocation to the plasma membrane provides scaffolding functions that lead to lipid and actin cytoskeleton re-arrangements, and enable recruitment of signaling proteins with consequences for focal adhesion assembly and signaling; (3) AnxA6-mediated inhibition of LE-cholesterol export causes cellular cholesterol imbalance that interferes with multiple Cav-1 and SNARE functions required for cellular movement. In fact, the latter function of AnxA6 listed here (see (3) above) might emerge as an overarching theme how cholesterol homeostasis coordinates the multiple involvements of AnxA6 in cell motility and adhesion. Recruitment of AnxA6 to cholesterol-rich late endosomes will most likely govern the amounts of AnxA6 that translocate into exosomes. This could be a crucial factor determining the levels of secreted AnxA6 and its impact on migration and adhesion (see (1) above). In addition, the ability of AnxA6 to control LE-cholesterol export critically affects microdomain formation at the cell surface. While this may not directly impact on constitutive, growth factor or Ca2+-induced AnxA6 interactions with signaling proteins in the cytosol, it could be decisive to define membrane recruitment and targeting of these complexes to specific sites in specialized microdomains (see (2) above). In this manner, up- or downregulation of a relatively small pool of AnxA6 proteins in LE through metabolic adaptation of cholesterol amounts delivered to this compartment would control the localization and activity of the total pool of AnxA6 proteins throughout the cell. Understanding how the scaffolding and cholesterol transport functions of AnxA6 are coordinated will ultimately contribute to a better understanding of how cellular cholesterol distribution links with domain formation, actin dynamics and signaling complex formation in cellular events driving cell migration and invasion.
Given that many cancer cells, including A431 cells, show elevated LDL receptor levels and increased LDL uptake,Citation114,139-141 AnxA6 expression levels could become a determining factor that permits or inhibits scenarios enabling Cav-1, Stx6, Stx4 and SNAP23 to promote integrin recycling, ECM cell surface delivery, or aberrant Src and FAK signaling. Furthermore, AnxA6 expression in A431 cells inhibited EGFR/Ras via PKCα/p120GAP pathways,Citation26,28,38 while AnxA6-induced cholesterol imbalance triggered Cav-1, SNAP23/Stx4 and Stx6 mislocalization to reduce caveolae formation, FN secretion and integrin recycling in these cells.,Citation27,29,119 Hence, the cooperative and simultaneous involvement of multiple AnxA6 properties in different cellular sites could decrease the ability of A431 cells, and possibly other cancer cells, to migrate and invade in 2- and 3-dimensional environments. Given the increased complexity of cell and animals models available, future studies will have to aim and dissect the contribution of the scaffolding and cholesterol transport functions of AnxA6 and their relative contribution to metastatic behavior.
Abbreviations
AnxA6 | = | annexin A6 |
ARFs | = | ADP ribosylation factors |
BCC | = | breast cancer cells |
Ca2+ | = | calcium |
Cav-1 | = | caveolin-1 |
CHO | = | Chinese Hamster Ovary |
ECM | = | extracellular matrix |
EGFR | = | epidermal growth factor receptor |
ER | = | estrogen receptor |
Erk | = | extracellular signal-regulated kinase |
FAK | = | focal adhesion kinase |
FN | = | fibronectin |
HNSCCs | = | head and neck squamous cell carcinoma |
LDL | = | low density lipoprotein |
LE | = | late endosomes |
MAPK | = | mitogen-activated protein kinase |
NPC1 | = | Niemann Pick Type C1 |
p120GAP | = | p120 GTPase activating protein |
PKCα | = | protein kinase Cα |
SNAP23 | = | soluble N-ethylmaleimide-sensitive fusion protein 23 |
SNARE | = | soluble NSF attachment protein (SNAP) receptor |
Stx | = | syntaxin |
Disclosure of potential conflicts of interest
No potential conflicts of interest were disclosed.
Acknowledgements
We would like to thank all members of our laboratories, past and present, for their invaluable contributions and apologize to all those researchers whose work could not be discussed owing to space limitations.
Funding
T.G. is supported by the University of Sydney (U7007, U7042, U7113, RY253), Sydney, Australia. C.E. is supported by grants from the Ministerio de Economía y Competitividad (BFU2015–66785-P and CSD2009–00016) and Fundació Marató TV3 (PI042182), Spain. C.R. is thankful to CONSOLIDER-INGENIO (MINECO) research program for post-doctoral fellowship [grant number CSD2009–00016] and to Fundació Marató TV3. P.T. acknowledges support from the NHMRC, Australian Research Council and Cancer Institute New South Wales (CINSW).
References
- Parsons JT, Horwitz AR, Schwartz MA. Cell adhesion: integrating cytoskeletal dynamics and cellular tension. Nat Rev Mol Cell Biol 2010; 11:633-43; PMID:20729930; https://doi.org/10.1038/nrm2957
- Caswell PT, Vadrevu S, Norman JC. Integrins: masters and slaves of endocytic transport. Nat Rev Mol Cell Biol 2009; 10:843-53; PMID:19904298; https://doi.org/10.1038/nrm2799
- Margadant C, Monsuur HN, Norman JC, Sonnenberg A. Mechanisms of integrin activation and trafficking. Curr Opin Cell Biol 2011; 23:607-14; PMID:21924601; https://doi.org/10.1016/j.ceb.2011.08.005
- Steeg PS. Targeting metastasis. Nat Rev Cancer 2016; 16:201-18; PMID:27009393; https://doi.org/10.1038/nrc.2016.25
- Astro V, de Curtis I. Plasma membrane-associated platforms: dynamic scaffolds that organize membrane-associated events. Sci Signal 2015; 8:re1; PMID:25759479; https://doi.org/10.1126/scisignal.aaa3312
- Langeberg LK, Scott JD. Signalling scaffolds and local organization of cellular behaviour. Nat Rev Mol Cell Biol 2015; 16:232-44; PMID:25785716; https://doi.org/10.1038/nrm3966
- Smith JM, Hedman AC, Sacks DB. IQGAPs choreograph cellular signaling from the membrane to the nucleus. Trends Cell Biol 2015; 25:171-84; PMID:25618329; https://doi.org/10.1016/j.tcb.2014.12.005
- Hoque M, Rentero C, Cairns R, Tebar F, Enrich C, Grewal T. Annexins – scaffolds modulating PKC localization and signaling. Cell Signal 2014; 26:1213-25; PMID:24582587; https://doi.org/10.1016/j.cellsig.2014.02.012
- Grewal T, Enrich C. Annexins–modulators of EGF receptor signaling and trafficking. Cell Signal 2009; 21:847-58; PMID:19385045; https://doi.org/10.1016/j.cellsig.2009.01.031
- Gerke V, Creutz CE, Moss SE. Annexins: linking Ca2+ signaling to membrane dynamics. Nat Rev Mol Cell Biol 2005; 6:449-61; PMID:15928709; https://doi.org/10.1038/nrm1661
- Gerke V, Moss SE. Annexins: from structure to function. Physiol Rev 2002; 82:331-71; PMID:11917092; https://doi.org/10.1152/physrev.00030.2001
- Hayes MJ, Rescher U, Gerke V, Moss SE. Annexin-actin interactions. Traffic 2004; 5:571-6; PMID:15260827; https://doi.org/10.1111/j.1600-0854.2004.00210.x
- Rescher U, Gerke V. Annexins—unique membrane binding proteins with diverse functions. J Cell Sci 2004; 117:2631-9; PMID:15169834; https://doi.org/10.1242/jcs.01245
- Perretti M, Flower RJ. Modulation of IL-1-induced neutrophil migration by dexamethasone and lipocortin 1. J Immunol 1993; 150:992-9; PMID:8423349
- Flower RJ, Rothwell NJ. Lipocortin-1: cellular mechanism and clinical relevance. Trends Pharmacol Sci 1994; 15:71-6; PMID:8184489; https://doi.org/10.1016/0165-6147(94)90281-X
- Chung CY, Murphy-Ullrich JE, Erickson HP. Mitogenesis, cell migration, and loss of focal adhesions by tenascin-C interacting with its cell surface receptor, annexin II. Mol Biol Cell 1996; 7:883-92; PMID:8816995; https://doi.org/10.1091/mbc.7.6.883
- Falcone DJ, Borth W, Khan KM, Hajjar KA. Plasminogen-mediated matrix invasion and degradation by macrophages is dependent on surface expression of annexin II. Blood 2001; 97:777-84; PMID:11157497; https://doi.org/10.1182/blood.V97.3.777
- Balch C, Dedman JR. Annexins II and V inhibit cell migration. Exp Cell Res 1997; 237:259-63; PMID:9434621; https://doi.org/10.1006/excr.1997.3817
- Qu J, Conroy LA, Walker JH, Wooding FB, Lucy JA. Phosphatidylserine-mediated adhesion of T-cells to endothelial cells. Biochem J 1996; 317:343-6; PMID:8713056; https://doi.org/10.1042/bj3170343
- Perretti M, D'Acquisto F. Annexin A1 and glucocorticoids as effectors of the resolution of inflammation. Nat Rev Immunol 2009; 9:62-70; PMID:19104500; https://doi.org/10.1038/nri2470
- Hedhli N, Falcone DJ, Huang B, Cesarman-Maus G, Kraemer R, Zhai H, Tsirka SE, Santambrogio L, Hajjar KA. The annexin A2/S100A10 system in health and disease: emerging paradigms. J Biomed Biotechnol 2012; 2012:406273; PMID:23193360; https://doi.org/10.1155/2012/406273
- Ueki H, Mizushina T, Laoharatchatathanin T, Terashima R, Nishimura Y, Rieanrakwong D, Yonezawa T, Kurusu S, Hasegawa Y, Brachvogel B, et al. Loss of maternal annexin A5 increases the likelihood of placental thrombosis and foetal loss. Sci Rep 2012; 2:827; PMID:23145320; https://doi.org/10.1038/srep00827
- Grewal T, Wason SJ, Enrich C, Rentero C. Annexins – insights from knockout mice. Biol Chem 2016; 397:1031-53; PMID:27318360; https://doi.org/10.1515/hsz-2016-0168
- Grewal T, Koese M, Rentero C, Enrich C. Annexin A6-regulator of the EGFR/Ras signalling pathway and cholesterol homeostasis. Int J Biochem Cell Biol 2010; 42:580-4; PMID:20044025; https://doi.org/10.1016/j.biocel.2009.12.020
- Enrich C, Rentero C, Vilà de Muga S, Reverter M, Mulay V, Wood P, Koese M, Grewal T. Annexin A6-Linking Ca(2+) signaling with cholesterol transport. Biochim Biophys Acta 2011; 1813:935-47; PMID:20888375; https://doi.org/10.1016/j.bbamcr.2010.09.015
- Grewal T, Evans R, Rentero C, Tebar F, Cubells L, de Diego I, Kirchhoff MF, Hughes WE, Heeren J, Rye KA, et al. Annexin A6 stimulates the membrane recruitment of p120GAP to modulate Ras and Raf-1 activity. Oncogene 2005; 24:5809-20; PMID:15940262; https://doi.org/10.1038/sj.onc.1208743
- Cubells L, Vilà de Muga S, Tebar F, Wood P, Evans R, Ingelmo-Torres M, Calvo M, Gaus K, Pol A, Grewal T, et al. Annexin A6-induced alterations in cholesterol transport and caveolin export from the Golgi complex. Traffic 2007; 8:1568-89; PMID:17822395; https://doi.org/10.1111/j.1600-0854.2007.00640.x
- Vilà de Muga S, Timpson P, Cubells L, Evans R, Hayes TE, Rentero C, Hegemann A, Reverter M, Leschner J, Pol A, et al. Annexin A6 inhibits Ras signalling in breast cancer cells. Oncogene 2009; 28:363-77; https://doi.org/10.1038/onc.2008.386
- García-Melero A, Reverter M, Hoque M, Meneses-Salas E, Koese M, Conway JR, Johnsen CH, Alvarez-Guaita A, Morales-Paytuvi F, Elmaghrabi YA, et al. Annexin A6 and late endosomal cholesterol modulate integrin recycling and cell migration. J Biol Chem 2016; 291:1320-35; https://doi.org/10.1074/jbc.M115.683557
- Sakwe AM, Koumangoye R, Guillory B, Ochieng J. Annexin A6 contributes to the invasiveness of breast carcinoma cells by influencing the organization and localization of functional focal adhesions. Exp Cell Res 2011; 317:823-37; PMID:21185831; https://doi.org/10.1016/j.yexcr.2010.12.008
- Tressler RJ, Yeatman T, Nicolson GL. Extracellular annexin VI is associated with divalent cation-dependent tumour cell-endothelial cell adhesion of metastatic RAW117 large-cell lymphoma cells. Exp Cell Res 1994; 215:395-400; PMID:7982479; https://doi.org/10.1006/excr.1994.1358
- Ishitsuka R, Kojima K, Utsumi H, Ogawa H, Matsumoto I. Glycosaminoglycan binding properties of annexin IV, V, and VI. J Biol Chem 1998; 273:9935-41; PMID:9545337; https://doi.org/10.1074/jbc.273.16.9935
- Takagi H, Asano Y, Yamakawa N, Matsumoto I, Kimata K. Annexin 6 is a putative cell surface receptor for chondroitin sulfate chains. J Cell Sci 2002; 115:3309-18; PMID:12140262
- Smythe E, Smith PD, Jacob SM, Theobald J, Moss SE. Endocytosis occurs independently of annexin VI in human A431 cells. J Cell Biol 1994; 124:301-6; PMID:7905003; https://doi.org/10.1083/jcb.124.3.301
- Sakwe AM, Koumangoye R, Goodwin SJ, Ochieng J. Fetuin-A ({alpha}2HS-glycoprotein) is a major serum adhesive protein that mediates growth signaling in breast tumour cells. J Biol Chem 2010; 285:41827-35; PMID:20956534; https://doi.org/10.1074/jbc.M110.128926
- Chen X, O'Neill KD, Chen X, Duan D, Wang E, Sturek MS, Edwards JM, Moe SM. Fetuin-A uptake in bovine vascular smooth muscle cells is calcium dependent and mediated by annexins. Am J Physiol Renal Physiol 2007; 292:F599-606; PMID:16968889; https://doi.org/10.1152/ajprenal.00303.2006
- Kundranda MN, Ray S, Saria M, Friedman D, Matrisian LM, Lukyanov P, Ochieng J. Annexins expressed on the cell surface serve as receptors for adhesion to immobilized fetuin-A. Biochim Biophys Acta 2004; 1693:111-23; PMID:15313013; https://doi.org/10.1016/j.bbamcr.2004.06.005
- Koese M, Rentero C, Kota BP, Hoque M, Cairns R, Wood P, Vilà de Muga S, Reverter M, Alvarez-Guaita A, Monastyrskaya K, et al. Annexin A6 is a scaffold for PKCα to promote EGFR inactivation. Oncogene 2013; 32:2858-72; PMID:22797061; https://doi.org/10.1038/onc.2012.303
- Ramírez-Mata A, Michalak C, Mendoza-Hernández G, León-Del-Río A, González-Noriega A. Annexin VI is a mannose-6-phosphate endocytic receptor for bovine ß-glucuronidase. Exp Cell Res 2011; 317:2364-73; https://doi.org/10.1016/j.yexcr.2011.05.025
- Bode G, Lüken A, Kerkhoff C, Roth J, Ludwig S, Nacken W. Interaction between S100A8/A9 and annexin A6 is involved in the calcium-induced cell surface exposition of S100A8/A9. J Biol Chem 2008; 283:31776-84; PMID:18786929; https://doi.org/10.1074/jbc.M803908200
- Greening DW, Gopal SK, Mathias RA, Liu L, Sheng J, Zhu HJ, Simpson RJ. Emerging roles of exosomes during epithelial-mesenchymal transition and cancer progression. Semin Cell Dev Biol 2015; 40:60-71; PMID:25721809; https://doi.org/10.1016/j.semcdb.2015.02.008
- Kapustin AN, Shanahan CM. Emerging roles for vascular smooth muscle cell exosomes in calicification and coagulation. J Physiol 2016; 594:2905-14; PMID:26864864; https://doi.org/10.1113/JP271340
- Cuervo AM, Gomes AV, Barnes JA, Dice JF. Selective degradation of annexins by chaperone-mediated autophagy. J Biol Chem 2000; 275:33329-35; PMID:10938088; https://doi.org/10.1074/jbc.M005655200
- Sung BH, Ketova T, Hoshino D, Zijlstra A, Weaver AM. Directional cell movement through tissues is controlled by exosome secretion. Nat Commun 2015; 6:7164; PMID:25968605; https://doi.org/10.1038/ncomms8164
- Kenific CM, Wittman T, Debnath J. Autophagy in adhesion and migration. J Cell Sci 2016; 129:3685-93; PMID:27672021; https://doi.org/10.1242/jcs.188490
- Leca J, Martinez S, Lac S, Nigri J, Secq V, Rubis M, Bressy C, Sergé A, Lavaut MN, Dusetti N, et al. Cancer-associated fibroblast-derived annexin A6+ extracellular vesicles support pancreatic cancer aggressiveness. J Clin Invest 2016; 126:4140-56; PMID:27701147; https://doi.org/10.1172/JCI87734
- Roostalu U, Strähle U. In vivo imaging of molecular interactions at damaged sarcolemma. Dev Cell 2012; 22:515-29; PMID:22421042; https://doi.org/10.1016/j.devcel.2011.12.008
- Swaggart KA, Demonbreun AR, Vo AH, Swanson KE, Kim EY, Fahrenbach JP, Holley-Cuthrell J, Eskin A, Chen Z, Squire K, et al. Annexin A6 modifies muscular dystrophy by mediating sarcolemmal repair. Proc Natl Acad Sci USA 2014; 111:6004-9; PMID:24717843; https://doi.org/10.1073/pnas.1324242111
- Demonbreun AR, Quattrocelli M, Barefield DY, Allen MV, Swanson KE, McNally EM. An actin-dependent annexin complex mediates plasma membrane repair in muscle. J Cell Biol 2016; 213:705-18; PMID:27298325; https://doi.org/10.1083/jcb.201512022
- Babiychuk EB, Monastyrskaya K, Potez S, Draeger A. Intracellular Ca(2+) operates a switch between repair and lysis of streptolysin O-perforated cells. Cell Death Differ 2009; 16:1126-34; PMID:19325569; https://doi.org/10.1038/cdd.2009.30
- Potez S, Luginbühl M, Monastyrskaya K, Hostettler A, Draeger A, Babiychuk EB. Tailored protection against plasmalemmal injury by annexins with different Ca2+ sensitivities. J Biol Chem 2011; 286:17982-91; PMID:21454475; https://doi.org/10.1074/jbc.M110.187625
- Zaidi AH, Gopalakrishnan V, Kasi PM, Zeng X, Malhotra U, Balasubramanian J, Visweswaran S, Sun M, Flint MS, Davison JM, et al. Evaluation of a 4-protein serum biomarker panel-biglycan, annexin-A6, myeloperoxidase, and protein S100-A9 (B-AMP)-for the detection of esophageal adenocarcinoma. Cancer 2014; 120:3902-13; PMID:25100294; https://doi.org/10.1002/cncr.28963
- Gaus K, Le Lay S, Balasubramanian N, Schwartz MA. Integrin-mediated adhesion regulates membrane order. J Cell Biol 2006; 174:725-34; PMID:16943184; https://doi.org/10.1083/jcb.200603034
- Alvarez-Guaita A, Vilà de Muga S, Owen DM, Williamson D, Magenau A, García-Melero A, Reverter M, Hoque M, Cairns R, Cornely R, et al. Evidence for annexin A6-dependent plasma membrane remodeling of lipid domains. Br J Pharmacol 2015; 172:1677-90; PMID:25409976; https://doi.org/10.1111/bph.13022
- Nassar ZD, Hill MM, Parton RG, Parat MO. Caveola-forming proteins caveolin-1 and PTRF in prostate cancer. Nat Rev Urol 2013; 10:529-36; PMID:23938946
- Goetz J, Lajoie P, Wiseman S, Nabi I. Caveolin-1 in tumour progression: the good, the bad and the ugly. Cancer Metastasis Rev 2008; 27:715-35; PMID:18506396; https://doi.org/10.1007/s10555-008-9160-9
- Núñez-Wehinger S, Ortiz RJ, Díaz N, Díaz J, Lobos-González L, Quest AF. Caveolin-1 in cell migration and metastasis. Curr Mol Med 2014; 14:255-74; https://doi.org/10.2174/1566524014666140128112827
- van Golen KL. Is caveolin-1 a viable therapeutic target to reduce cancer metastasis?. Expert Opin Ther Targets 2006; 10:709-21; PMID:16981828; https://doi.org/10.1517/14728222.10.5.709
- Kamal A, Ying Y, Anderson RG. Annexin VI-mediated loss of spectrin during coated pit budding is coupled to delivery of LDL to lysosomes. J Cell Biol 1998; 142:937-47; PMID:9722607; https://doi.org/10.1083/jcb.142.4.937
- Jones S, Rappoport JZ. Interdependent epidermal growth factor receptor signaling and trafficking. Int J Biochem Cell Biol 2014; 51:23-8; PMID:24681003; https://doi.org/10.1016/j.biocel.2014.03.014
- Paul NR, Jacquemet G, Caswell PT. Endocytic trafficking of integrins in cell migration. Curr Biol 2015; 25:R1092-105; PMID:26583903; https://doi.org/10.1016/j.cub.2015.09.049
- Hawkins TE, Roes J, Rees D, Monkhouse J, Moss SE. Immunological development and cardiovascular function are normal in annexin VI null mutant mice. Mol Cell Biol 1999; 19:8028-32; PMID:10567528; https://doi.org/10.1128/MCB.19.12.8028
- Cornely R, Pollock AH, Rentero C, Norris SE, Alvarez-Guaita A, Grewal T, Mitchell T, Enrich C, Moss SE, Parton RG, et al. Annexin A6 regulates interleukin-2-mediated T-cell proliferation. Immunol Cell Biol 2016; 94:543-53; PMID:26853809; https://doi.org/10.1038/icb.2016.15
- Monastyrskaya K, Tschumi F, Babiychuk EB, Stroka D, Draeger A. Annexins sense changes in intracellular pH during hypoxia. Biochem J 2008; 409:65-75; PMID:17824845; https://doi.org/10.1042/BJ20071116
- de Diego I, Schwartz F, Siegfried H, Dauterstedt P, Heeren J, Beisiegel U, Enrich C, Grewal T. Cholesterol modulates the membrane binding and intracellular distribution of annexin 6. J Biol Chem 2002; 277:32187-94; PMID:12070178; https://doi.org/10.1074/jbc.M205499200
- Calderwood DA, Campbell ID, Critchley DR. Talins and kindlins: partners in integrin-mediated adhesion. Nat Rev Mol Cell Biol 2013; 14:503-17; PMID:23860236; https://doi.org/10.1038/nrm3624
- Hosoya H, Kobayashi R, Tsukita S, Matsumura F. Ca(2+)-regulated actin and phospholipid binding protein (68 kD-protein) from bovine liver: identification as a homologue for annexin VI and intracellular localization. Cell Motil Cytoskeleton 1992; 22:200-10; PMID:1423665; https://doi.org/10.1002/cm.970220307
- Mishra S, Chander V, Banerjee P, Oh JG, Lifirsu E, Park WJ, Kim DH, Bandyopadhyay A. Interaction of annexin A6 with alpha actinin in cardiomyocytes. BMC Cell Biol 2011; 12:7; PMID:21272378; https://doi.org/10.1186/1471-2121-12-7
- Goldberg M, Feinberg J, Lecolle S, Kaetzel MA, Rainteau D, Lessard JL, Dedman JR, Weinman S. Co-distribution of annexin VI and actin in secretory ameloblasts and odontoblasts of rat incisor. Cell Tissue Res 1991; 263:81-9; PMID:1826233; https://doi.org/10.1007/BF00318402
- Buccione R, Orth JD, McNiven MA. Foot and mouth: podosomes, invadopodia and circular dorsal ruffles. Nat Rev Mol Cell Biol 2004; 5:647-57; PMID:15366708; https://doi.org/10.1038/nrm1436
- Babiychuk EB, Draeger A. Biochemical characterization of detergent-resistent membranes: a systematic approach. Biochem J 2006; 397:407-16; PMID:16608442; https://doi.org/10.1042/BJ20060056
- Monastyrskaya K, Babiychuk EB, Hostettler A, Wood P, Grewal T, Draeger A. Plasma membrane-associated annexin A6 reduces Ca2+ entry by stabilizing the cortical actin cytoskeleton. J Biol Chem 2009; 284:17227-42; PMID:19386597; https://doi.org/10.1074/jbc.M109.004457
- Tsai FC, Kuo GH, Chang SW, Tsai PJ. Ca2+ signaling in cytoskeletal reorganization, cell migration and cancer metastasis. Biomed Res Int 2015; 2015:409245; PMID:25977921
- Lin-Moshier Y, Keebler MV, Hooper R, Boulware MJ, Liu X, Churamani D, Abood ME, Walseth TF, Brailoiu E, Patel S, et al. The Two-pore channel (TPC) interactome unmasks isoform-specific roles for TPCs in endolysosomal morphology and cell pigmentation. Proc Natl Acad Sci USA 2014; 111:13087-92; PMID:25157141; https://doi.org/10.1073/pnas.1407004111
- Díaz-Muñoz M, Hamilton SL, Kaetzel MA, Hazarika P, Dedman JR. Modulation of Ca2+ release channel activity from sarcoplasmic reticulum by annexin VI (67-kDa calcimedin). J Biol Chem 1990; 265:15894-9
- Carragher NO, Frame MC. Calpain: a role in cell transformation and migration. Int J Biochem Cell Biol 2002; 34:1539-43; PMID:12379276; https://doi.org/10.1016/S1357-2725(02)00069-9
- Wells A, Huttenlocher A, Lauffenburger DA. Calpain proteases in cell adhesion and motility. Int Rev Cytol 2005; 245:1-16; PMID:16125543; https://doi.org/10.1016/S0074-7696(05)45001-9
- Pons M, Grewal T, Rius E, Schnitgerhans T, Jäckle S, Enrich C. Evidence for the involvement of annexin 6 in the trafficking between the endocytic compartment and lysosomes. Exp Cell Res 2001; 269:13-22; PMID:11525635; https://doi.org/10.1006/excr.2001.5268
- Lin HC, Sudhof TC, Anderson RG. Annexin VI is required for budding of clathrin-coated pits. Cell 1992; 70:283-91; PMID:1386288; https://doi.org/10.1016/0092-8674(92)90102-I
- Creutz CE, Snyder SL. Interactions of annexins with the mu subunits of the clathrin assembly proteins. Biochemistry 2005; 44:13795-806; PMID:16229469; https://doi.org/10.1021/bi051160w
- Ng BF, Selvaraj GK, Santa-Cruz Mateos C, Grosheva I, Alvarez-Garcia I, Martín-Bermudo MD, Palacios IM. α-Spectrin and integrins act together to regulate actomyosin and columnarization, and to maintain a monolayered follicular epithelium. Development 2016; 143:1388-99; PMID:26952981; https://doi.org/10.1242/dev.130070
- Ponceau A, Albigès-Rizo C, Colin-Aronovicz Y, Destaing O, Lecomte MC. αll-spectrin regulates invadosome stability and extracellular matrix degradation. PLoS One 2015; 10:e0120781; PMID:25830635; https://doi.org/10.1371/journal.pone.0120781
- De Franceschi N, Arjonen A, Elkhatib N, Denessiouk K, Wrobel AG, Wilson TA, Pouwels J, Montagnac G, Owen DJ, Ivaska J. Selective integrin endocytosis is driven by interactions between the integrin α-chain and AP2. Nat Struct Mol Biol 2016; 23:172-9; PMID:26779610; https://doi.org/10.1038/nsmb.3161
- Pons M, Tebar F, Kirchhoff M, Peiró S, de Diego I, Grewal T, Enrich C. Activation of Raf-1 is defective in annexin 6 overexpressing Chinese hamster ovary cells. FEBS Lett 2001; 501:69-73; PMID:11457458; https://doi.org/10.1016/S0014-5793(01)02635-7
- Theobald J, Hanby A, Patel K, Moss SE. Annexin VI has tumour-suppressor activity in human A431 squamous epithelial carcinoma cells. Br J Cancer 1995; 71:786-8; PMID:7710945; https://doi.org/10.1038/bjc.1995.152
- Appert-Collin A, Hubert P, Crémel G, Bennasroune A. Role of ErbB receptors in cancer cell migration and invasion. Front Pharmacol 2015; 6:283; PMID:26635612; https://doi.org/10.3389/fphar.2015.00283
- Kim LC, Song L, Haura EB. Src kinases as therapeutic targets in cancer. Nat Rev Clin Oncol 2009; 6:587-95; PMID:19787002; https://doi.org/10.1038/nrclinonc.2009.129
- Kinbara K, Goldfinger LE, Hansen M, Chou FL, Ginsberg MH. Ras GTPases: integrin's friends or foes?. Nat Rev Mol Cell Biol 2003; 4:767-76; PMID:14570053; https://doi.org/10.1038/nrm1229
- Eberwein P, Laird D, Schulz S, Reinhard T, Steinberg T, Tomakidi P. Modulation of focal adhesion constituents and their down-stream events by EGF: On the cross-talk of integrins and growth factor receptors. Biochim Biophys Acta 2015; 1853:2183-98; PMID:26079101; https://doi.org/10.1016/j.bbamcr.2015.06.004
- Michael N, Jura N. Src defines a new pool of EGFR substrates. Nat Struct Mol Biol 2015; 22:945-7; PMID:26643845; https://doi.org/10.1038/nsmb.3137
- Chow A, Davis AJ, Gawler DJ. Identification of a novel complex containing annexin VI, Fyn, Pyk2, and the p120(GAP) C2 domain. FEBS Lett 2000; 469:88-92; PMID:10708762; https://doi.org/10.1016/S0014-5793(00)01252-7
- Dubois T, Soula M, Moss SE, Russo-Marie F, Rothhut B. Potential interaction between annexin VI and a 56-kDa protein kinase in T cells. Biochem Biophys Res Commun 1995; 212:270-8; PMID:7541988; https://doi.org/10.1006/bbrc.1995.1966
- Grewal T, Enrich C. Molecular mechanisms involved in Ras inactivation: the annexin 6-p120GAP complex. Bioessays 2006; 28:1211-20; PMID:17120209; https://doi.org/10.1002/bies.20503
- Zhou Y, Hancock JF. Ras nanoclusters: versatile lipid-based signaling platforms. Biochim Biophys Acta 2015; 1853:841-9; PMID:25234412; https://doi.org/10.1016/j.bbamcr.2014.09.008
- Alvarez-Moya B, López-Alcalá C, Drosten M, Bachs O, Agell N. K-Ras4B phosphorylation at Ser181 is inhibited by calmodulin and modulates K-Ras activity and function. Oncogene 2010; 29:5911-22; PMID:20802526; https://doi.org/10.1038/onc.2010.298
- Murakoshi H, Iino R, Kobayashi T, Fujiwara T, Ohshima C, Yoshimura A, Kusumi A. Single-molecule imaging analysis of Ras activation in living cells. Proc Natl Acad Sci USA 2004; 101:7317-22; PMID:15123831; https://doi.org/10.1073/pnas.0401354101
- Kulkarni SV, Gish G, van der Geer P, Henkemeyer M, Pawson T. Role of p120 Ras-GAP in directed cell movement. J Cell Biol 2000; 149:457-70; PMID:10769036; https://doi.org/10.1083/jcb.149.2.457
- Endo M, Yamashita T. Inactivation of Ras by p120GAP via focal adhesion kinase dephosphorylation mediates RGMa-induced growth cone collapse. J Neurosci 2009; 29:6649-62; PMID:19458235; https://doi.org/10.1523/JNEUROSCI.0927-09.2009
- Pamonsinlapatham P, Gril B, Dufour S, Hadj-Slimane R, Gigoux V, Pethe S, L'hoste S, Camonis J, Garbay C, Raynaud F, et al. Capns1, a new binding partner of RasGAP-SH3 domain in K-Ras(V12) oncogenic cells: modulation of cell survival and migration. Cell Signal 2008; 20:2119-26; PMID:18761085; https://doi.org/10.1016/j.cellsig.2008.08.005
- Leblanc V, Tocque B, Delumeau I. Ras-GAP controls Rho-mediated cytoskeletal reorganization through its SH3 domain. Mol Cell Biol 1998; 18:5567-78; PMID:9710640; https://doi.org/10.1128/MCB.18.9.5567
- Mai A, Veltel S, Pellinen T, Padzik A, Coffey E, Marjomäki V, Ivaska J. Competitive binding of Rab21 and p120RasGAP to integrins regulates receptor traffic and migration. J Cell Biol 2011; 194:294-306; https://doi.org/10.1083/jcb.201012126
- Tomar A, Lim ST, Lim Y, Schlaepfer DD. A FAK-p120RasGAP-p190RhoGAP complex regulates polarity in migrating cells. J Cell Sci 2009; 122:1852-62; PMID:19435801; https://doi.org/10.1242/jcs.046870
- Grewal T, Koese M, Tebar F, Enrich C. Differential regulation of RasGAPs in cancer. Genes Cancer 2011; 2:288-97; PMID:21779499; https://doi.org/10.1177/1947601911407330
- Sigismund S, Argenzio E, Tosoni D, Cavallaro E, Polo S, Di Fiore PP. Clathrin-mediated internalization is essential for sustained EGFR signaling but dispensible for degradation. Dev Cell 2008; 15:209-19; PMID:18694561; https://doi.org/10.1016/j.devcel.2008.06.012
- Rentero C, Evans R, Wood P, Tebar F, Vilà de Muga S, Cubells L, de Diego I, Hayes TE, Hughes WE, Pol A, et al. Inhibition of H-Ras and MAPK is compensated by PKC-dependent pathways in annexin A6 expressing cells. Cell Signal 2006; 18:1006-16; PMID:16183252; https://doi.org/10.1016/j.cellsig.2005.08.008
- Ng T, Shima D, Squire A, Bastiaens PI, Gschmeissner S, Humphries MJ, Parker PJ. PKCalpha regulates beta1 integrin-dependent cell motility through association and control of integrin traffic. EMBO J 1999; 18:3909-23; PMID:10406796; https://doi.org/10.1093/emboj/18.14.3909
- Ways DK, Kukoly CA, deVente J, Hooker JL, Bryant WO, Posekany KJ, Fletcher DJ, Cook PP, Parker PJ. MCF-7 breast cancer cells transfected with protein kinase C-alpha exhibit altered expression of other protein kinase C isoforms and display a more aggressive neoplastic phenotype. J Clin Invest 1995; 95:1906-15; PMID:7706498; https://doi.org/10.1172/JCI117872
- Tan M, Li P, Sun M, Yin G, Yu D. Upregulation and activation of PKC alpha by ErbB2 through Src promotes breast cancer cell invasion that can be blocked by combined treatment with PKC alpha and Src inhibitors. Oncogene 2006; 25:3286-95; PMID:16407820; https://doi.org/10.1038/sj.onc.1209361
- Wang Y, Arjonen A, Pouwels J, Ta H, Pausch P, Bange G, Engel U, Pan X, Fackler OT, Ivaska J, et al. Formin-like 2 promotes β1-integrin trafficking and invasive motility downstream of PKCα;. Dev Cell 2015; 34:1-9; PMID:26151901; https://doi.org/10.1016/j.devcel.2015.06.022
- Ng T, Parsons M, Hughes WE, Monypenny J, Zicha D, Gautreau A, Arpin M, Gschmeissner S, Verveer PJ, Bastiaens PI, et al. Ezrin is a downstream effector of trafficking PKC-integrin complexes involved in the control of cell motility. EMBO J 2001; 20:2723-41; PMID:11387207; https://doi.org/10.1093/emboj/20.11.2723
- Koumangoye RB, Nangami GN, Thompson PD, Agboto VK, Ochieng J, Sakwe AM. Reduced annexin A6 expression promotes the degradation of activated epidermal growth factor receptor and sensitizes invasive breast cancer cells to EGFR-targeted tyrosine kinase inhibitors. Mol Cancer 2013; 12:167; PMID:24354805; https://doi.org/10.1186/1476-4598-12-167
- Wu CY, Taneyhill LA. Annexin a6 modulates chick cranial neural crest emigration. PLoS One 2012; 7:e44903; PMID:22984583; https://doi.org/10.1371/journal.pone.0044903
- Navarro-Lérida I, Sánchez-Perales S, Calvo M, Rentero C, Zheng Y, Enrich C, Del Pozo MA. A palmitoylation switch mechanism regulates Rac1 function and membrane organization. EMBO J 2012; 31:534-51; https://doi.org/10.1038/emboj.2011.446
- Hoque M, Rentero C, Conway JR, Murray RZ, Timpson P, Enrich C, Grewal T. The cross-talk of LDL-cholesterol with cell motility: insights from the Niemann Pick Type C1 mutation and altered integrin trafficking. Cell Adh Migr 2015; 9:384-91; PMID:26366834; https://doi.org/10.1080/19336918.2015.1019996
- Ikonen E. Cellular cholesterol trafficking and compartmentalization. Nat Rev Mol Cell Biol 2008; 9:125-38; PMID:18216769; https://doi.org/10.1038/nrm2336
- Reverter M, Rentero C, Garcia-Melero A, Hoque M, Vilà de Muga S, Alvarez-Guaita A, Conway JR, Wood P, Cairns R, Lykopoulou L, et al. Cholesterol regulates Syntaxin 6 trafficking at trans-Golgi network endosomal boundaries. Cell Rep 2014; 7:883-97; PMID:24746815; https://doi.org/10.1016/j.celrep.2014.03.043
- Kanerva K, Uronen RL, Blom T, Li S, Bittman R, Lappalainen P, Peranen J, Raposo G, Ikonen E. LDL cholesterol recycles to the plasma membrane via a Rab8a-Myosin5b-actin-dependent membrane transport route. Dev Cell 2013; 27:249-62; PMID:24209575; https://doi.org/10.1016/j.devcel.2013.09.016
- Cubells L, Vilà de Muga S, Tebar F, Bonventre JV, Balsinde J, Pol A, Grewal T, Enrich C. Annexin A6-induced inhibition of cytoplasmic phospholipase A2 is linked to caveolin-1 export from the Golgi. J Biol Chem 2008; 283:10174-83; PMID:18245088; https://doi.org/10.1074/jbc.M706618200
- Reverter M, Rentero C, Vilà de Muga S, Alvarez-Guaita A, Mulay V, Cairns R, Wood P, Monastyrskaya K, Pol A, Tebar F, et al. Cholesterol transport from late endosomes to the Golgi regulates t-SNARE trafficking, assembly, and function. Mol Biol Cell 2011; 22:4108-23; PMID:22039070; https://doi.org/10.1091/mbc.E11-04-0332R
- Grewal T, Heeren J, Mewawala D, Schnitgerhans T, Wendt D, Salomon G, Enrich C, Beisiegel U, Jäckle S. Annexin VI stimulates endocytosis and is involved in the trafficking of low density lipoproteins to the prelysosomal compartment. J Biol Chem 2000; 275:33806-13; PMID:10940299; https://doi.org/10.1074/jbc.M002662200
- Ivaska J. Unanchoring integrins in focal adhesions. Nat Cell Biol 2012; 14:981-3; PMID:23033047; https://doi.org/10.1038/ncb2592
- Creutz CE. The annexins and exocytosis. Science 1992; 258:924-31; PMID:1439804; https://doi.org/10.1126/science.1439804
- Donnelly SR, Moss SE. Annexins in the secretory pathway. Cell Mol Life Sci 1997; 53:533-8; PMID:9230932; https://doi.org/10.1007/s000180050068
- Podszywalow-Bartnicka P, Kosiorek M, Piwocka K, Sikora E, Zablocki K, Pikula S. Role of annexin A6 isoforms in catecholamine secretion by PC12 cells: distinct influence on calcium response. J Cell Biochem 2010; 111:168-78; PMID:20506562; https://doi.org/10.1002/jcb.22685
- Williams KC, McNeilly RE, Coppolino MG. SNAP23, Syntaxin4, and vesicle-associated membrane protein 7 (VAMP7) mediate trafficking of membrane type 1-matrix metalloproteinase (MT1-MMP) during invadopodium formation and tumour cell invasion. Mol Biol Cell 2014; 25:2061-70; PMID:24807903; https://doi.org/10.1091/mbc.E13-10-0582
- Tiwari A, Jung JJ, Inamdar SM, Brown CO, Goel A, Choudhury A. Endothelial cell migration on fibronectin is regulated by syntaxin 6-mediated alpha5beta1 integrin recycling. J Biol Chem 2011; 286:36749-61; PMID:21880737; https://doi.org/10.1074/jbc.M111.260828
- Riggs KA, Hasan N, Humphrey D, Raleigh C, Nevitt C, Corbin D, Hu C. Regulation of integrin endocytic recycling and chemotactic cell migration by syntaxin 6 and VAMP3 interaction. J Cell Sci 2012; 125:3827-39; PMID:22573826; https://doi.org/10.1242/jcs.102566
- Du J, Liu X, Wu Y, Zhu J, Tang Y. Essential role of Stx6 in esophageal squamous cell carcinoma growth and migration. Biochem Biophys Res Commun 2016; 472:60-7; PMID:26906622; https://doi.org/10.1016/j.bbrc.2016.02.061
- Hulce JJ, Cognetta AB, Niphakis MJ, Tully SE, Cravatt BF. Proteome-wide mapping of cholesterol-interacting proteins in mammalian cells. Nat Methods 2013; 10:259-64; PMID:23396283; https://doi.org/10.1038/nmeth.2368
- Enrich C, Rentero C, Hierro A, Grewal T. Role of cholesterol in SNARE-mediated trafficking on intracellular membranes. J Cell Sci 2015; 128:1071-81; PMID:25653390; https://doi.org/10.1242/jcs.164459
- Williams KC, Coppolino MG. SNARE-dependent interaction of Src, EGFR and beta1 integrin regulates invadopodia formation and tumour cell invasion. J Cell Sci 2014; 127:1712-25; PMID:24496451; https://doi.org/10.1242/jcs.134734
- Domon M, Nasir MN, Matar G, Pikula S, Besson F, Bandorowicz-Pikula J. Annexins as organizers of cholesterol- and sphingomyelin-enriched membrane microdomains in Niemann-Pick type C disease. Cell Mol Life Sci 2012; 69:1773-85; PMID:22159585; https://doi.org/10.1007/s00018-011-0894-0
- Borg M, Bakke O, Progida C. A novel interaction between Rab7b and actomyosin reveals a dual role in intracellular transport and cell migration. J Cell Sci 2014; 127:4927-39; PMID:25217632; https://doi.org/10.1242/jcs.155861
- da Silva SD, Marchi FA, Xu B, Bijian K, Alobaid F, Mlynarek A, Rogatto SR, Hier M, Kowalski LP, Alaoui-Jamali MA. Predominant rab-GTPase amplicons contributing to oral squamous cell carcinoma progression to metastasis. Oncotarget 2015; 6:21950-63; PMID:26110570; https://doi.org/10.18632/oncotarget.4277
- Steffan JJ, Dykes SS, Coleman DT, Adams LK, Rogers D, Carroll JL, Williams BJ, Cardelli JA. Supporting a role for the GTPase Rab7 in prostate cancer progression. PLoS One 2014; 9:e87882; PMID:24505328; https://doi.org/10.1371/journal.pone.0087882
- Rainero E, Norman JC. Late endosomal and lysosomal trafficking during integrin-mediated cell migration and invasion: cell matrix receptors are trafficked through the late endosomal pathway in a way that dictates how cells migrate. Bioessays 2013; 35:523-32; PMID:23605698; https://doi.org/10.1002/bies.201200160
- Tu C, Ortega-Cava CF, Winograd P, Stanton MJ, Reddi AL, Dodge I, Arya R, Dimri M, Clubb RJ, Naramura M, et al. Endosomal-sorting complexes required for transport (ESCRT) pathway-dependent endosomal traffic regulates the localization of active Src at focal adhesions. Proc Natl Acad Sci USA 2010; 107:16107-12; PMID:20805499; https://doi.org/10.1073/pnas.1009471107
- Timpson P, Jones GE, Frame MC, Brunton VG. Coordination of cell polarization and migration by the Rho family GTPases requires Src kinase activity. Curr Biol 2001; 11:1836-46; PMID:11728306; https://doi.org/10.1016/S0960-9822(01)00583-8
- Muller PA, Caswell PT, Doyle B, Iwanicki MP, Tan EH, Karim S, Lukashchuk N, Gillespie DA, Ludwig RL, Gosselin P, et al. Mutant p53 drives invasion by promoting integrin recycling. Cell 2009; 139:1327-41; PMID:20064378; https://doi.org/10.1016/j.cell.2009.11.026
- Freed-Pastor WA, Mizuno H, Zhao X, Langerod A, Moon SH, Rodriguez-Barrueco R, Barsotti A, Chicas A, Li W, Polotskaia A, et al. Mutant p53 disrupts mammary tissue architecture via the mevalonate pathway. Cell 2012; 148:244-58; PMID:22265415; https://doi.org/10.1016/j.cell.2011.12.017
- Cruz PM, Mo H, McConathy WJ, Sabnis N, Lacko AG. The role of cholesterol metabolism and cholesterol transport in carcinogenesis: a review of scientific findings, relevant to future cancer therapeutics. Front Pharmacol 2013; 4:119; PMID:24093019; https://doi.org/10.3389/fphar.2013.00119