ABSTRACT
Targeting inflammation in cancer has shown promise to improve and complement current therapies. The tumor microenvironment plays an important role in cancer growth and metastasis and -tumor associated macrophages possess pro-tumoral and pro-metastatic properties. Annexin A1 (ANXA1) is an immune-modulating protein with diverse functions in the immune system and in cancer. In breast cancer, high ANXA1 expression leads to poor prognosis and increased metastasis. Here, we will review ANXA1 as a modulator of inflammation, and discuss its importance in breast cancer and highlight its new role in alternative macrophage activation in the tumor microenvironment. This review may provide an updated understanding into the various roles of ANXA1 which may enable future therapeutic developments for the treatment of breast cancer.
Introduction
Breast cancer is a diverse and heterogenous complex disease, morphologically characterized by different tumor cell mitosis, cell differentiation and nuclear pleomorphism, indicating aggressiveness and an independent prognostic biomarker [Citation1]. The estrogen receptor (ER), progesterone receptor (PR), and human epidermal growth factor receptor 2 (Her2), are established biomarkers in common clinical use as disease indicators and therapeutic targets [Citation2]. Although early detection has become possible, the increased mortality rate is primarily due to metastasis, which is the major factor to a poor prognostic in breast cancer patients.
A hallmark of cancer is the correlation between inflammation to various steps involved in tumorigenesis, including cellular transformation, promotion, proliferation, invasion, angiogenesis and metastasis [Citation3]. Inflammatory cells are commonly found to be activated within the tumor environment such as increased leukocytes infiltration, overexpression of cytokines, chemokines and growth factors that can stimulate proliferation, inhibit apoptosis and generate reactive oxygen species [Citation4]. Furthermore, accumulate evidence suggest that inflammation has been considered an important prognostic marker for breast cancer due the ability of these cells synthetize vascular endothelial grown factors and other proteins that can stimulate vascular endothelial cell mitosis and extracellular matrix remodelling [Citation5].
Breast carcinomas are infiltrated by various types of host leukocytes involving activation of humoral immunity to promote infiltration of T helper type 2 (Th2) cells and monocytes that differentiate into tumor-associated macrophages (TAM) at the tumor sites [Citation3]. The complex interplay between cancer cells and the host immune response has been well established, supporting the concept that disruption of endogenous mechanisms that promotes resolution of inflammation, could result in tumor progression [Citation3,Citation6]. Therefore, macrophages, as key regulators of host immunity, have a pivotal role on the tumor microenvironment [Citation7].
Evidence from experimental, clinical and epidemiological studies have demonstrated a strong association between TAM density and poor prognosis in several types of cancer, including breast cancer [Citation8], and these effects may be due the ability of TAM to secrete cytokines, growth factors, chemokines and proteins that can stimulate cancer proliferation and cell invasion [Citation9,Citation10]. Moreover, TAM-derived cytokines may promote the invasiveness of tumor cells by enhancing their adhesion to extracellular matrix in the tumor stroma [Citation11]. TAMs express several pro-angiogenic growth factors, cytokines and chemokines such as vascular endothelial growth factor (VEGF), epidermal growth factor (EGF), matrix metalloproteinase (MMP)-9, macrophage colony stimulating factor (M-CSF/CSF-1), tumour necrosis factor alpha (TNFα), C-C motif chemokine ligand (CCL)-2 and CCL5. These factors are produced by cancer cells themselves, and such autocrine signalling also contributes to cancer development [Citation12], however the underlying signals and mechanism which TAMs provide to the tumour cells to promote metastasis remain to be established.
ANXA1 structure and immunomodulatory function
ANXA1, a highly abundant 37-kDa protein member of the annexin superfamily of calcium- and phospholipid-binding proteins, was initially shown to be a glucocorticoid-regulated phospholipase A2 (PLA2) inhibitor [Citation13]. It is composed of 346 amino acids and consists of two different regions, the singular N-terminal domain, also called the tail, and the C-terminal domain, named the core domain [Citation14,Citation15]. ANXA1 represents 2–4% of the total cytosolic protein in several cell types and is also located in the nucleus [Citation16]. In the peripheral blood cells, ANXA1 is highly expressed in subcellular granules of neutrophils, eosinophils and monocytes, with small amounts expressed in subsets of T lymphocytes, mast and B cells. The biological effects of ANXA1 are mediated through a family of G-protein-coupled receptors (GPCRs) known as formyl peptide receptors (FPRs) that includes FPR1, FPR2 (also known as FPR2/ALX or formyl peptide receptor like-1) and FPR3 [Citation17]. The annexin superfamily of calcium and phospholipid-binding proteins has been implicated in many physiological processes, including differentiation, apoptosis, proliferation and inflammation [Citation18].
Since its discovery, nearly four decades ago, ANXA1 has been shown to be capable of modulating the immune system to affect a number of anti- and pro-inflammatory events. including inhibition of leukocyte activation and migration [Citation19], apoptosis and tissue growth [Citation20,Citation21] and ischemia-reperfusion injury [Citation22]. ANXA1 is also involved in an inflammation-pro-resolving process, which is associated with a wide range of cellular and molecular steps of the inflammatory response and is deeply involved in the endogenous mechanisms that are activated to bring about the resolution of inflammation. Endogenous and exogenous ANXA1 binding to FPRs on neutrophils results in decreased neutrophil rolling and adhesion to endothelium, increased detachment of adherent cells, and inhibition of neutrophil transmigration [Citation23]. In addition, ANXA1 induces apoptosis, leading the pro-survival signals that cause prolonged lifespan of neutrophils at the inflammatory site, promoting the recruitment and clearance of apoptotic neutrophils by resolving macrophages [Citation24]. ANXA1 regulates several mediators that are involved in the inflammatory response, such cyclooxygenase-2 (COX-2), inducible nitric oxide synthase (iNOS) and the production and secretion of pro-inflammatory cytokines by macrophages [Citation25,Citation26]. ANXA1 is important in the regulation of dendritic cell maturation and activation [Citation27] and plays important roles in the regulation of toll like receptor and type-1 interferon responses in macrophages [Citation28], enhancing the activation of transcription factors such as nuclear factor- kappa-B (NF-kB) [Citation27] and interferon response factor- 3 associating with upstream signal proteins TANK-binding kinase [Citation28]. ANXA1 may also play an important role in tumour development and progression [Citation29]. In this review, we will discuss the role of ANXA1 in breast cancer, in the context of inflammation and migration of cancer cells and its role in the tumour microenvironment.
ANXA1 and macrophages in the tumor microenvironment
Tumour-associated macrophages (TAM) are well-known active components in the process of tumour progression and invasion, and are key producers of many mediators such as chemokines, which take part in the activation and maintenance of the inflammatory process [Citation6]. Several clinical studies demonstrated that high numbers of intra-tumour macrophages correlate with high vessel density and tumour progression [Citation30]. Macrophages are heterogeneous cells that are released from the bone marrow as immature monocytes and after circulating in the blood vessels, migrate into target tissues to undergo final differentiation into mature macrophages [Citation31]. Monocytes/macrophages play a critical role for tissue remodelling, inflammation, and immunity including phagocytosis and secretion of cytokines, proteases, and growth factors [Citation7,Citation32]. The complex interplay between cancer cells and the host immune response has been well established, supporting the concept that disruption of endogenous mechanisms that promotes resolution of inflammation, could result in tumour progression [Citation9,Citation33,Citation34]. Therefore, macrophages, as key regulators of host immunity, have a pivotal role on the tumour microenvironment [Citation7,Citation33].
The paradoxical interaction between macrophages and cancer reflect the functional plasticity of these cells. Macrophages can differentiate into a spectrum of distinct functional phenotypes in response to signals present within individual microenvironments and includes the classically activated macrophages (M1), and alternatively activated macrophages (M2). M1 phenotype has potent microbicidal activity, promotes Th1 responses and they are characterized by the production of pro-inflammatory factors such as interleukin (IL)-12 and tumour necrosis factor-α (TNF-α), whereas M2 phenotype drives angiogenesis, tumour progression and promotes Th2-type adaptive immune response with increased secretion of cytokine IL-10 and transforming growth factor-β (TGF-β) [Citation7,Citation10].
The M2 phenotype can be further subdivided into M2a, M2b, M2c and M2d cells according to the different stimuli that activates them [Citation35,Citation36]. M2a and M2b macrophages promote immune regulatory functions and drive Th2 response, whereas M2c macrophages have a predominant role in suppressing inflammation and promoting tissue remodelling. M2d macrophages, also termed as tumour-associated macrophages (TAMs), are a major source of vascular endothelial growth factors, which accumulate at the tumour site by tumour-derived signals, such as macrophage colony stimulating factor (M-CSF/CSF-1), vascular epithelial growth factor (VEGF) and chemokines CCL2 and CCL5) [Citation7,Citation36]. The strong correlation between infiltrated TAMs and tumour cells are generally characterized as M2 phenotype and promote tumour growth and vasculature [Citation37,Citation38]. Moreover, many functions have been attributed to macrophages during tumour growth and progression, including extracellular matrix remodelling, promoting tumour cell invasion and metastasis, angiogenesis and lymphangiogenesis [Citation6]. In addition, in the tumour microenvironment, macrophages demonstrated an immunosuppressive activity through the expression of several molecules, including TGF-β, iNOS, arginase-1 and IL-10, known for their immunosuppressive role [Citation3,Citation7].
In vivo studies using murine models of breast cancer suggested that T cell suppression is dependent partially on TAM metabolic activities via arginase-1 or iNOS [Citation39,Citation40]. In addition, TAM can induce the expression of these molecules on the cancer cell surface, strongly providing a novel mechanism by which cancer cells escape the immune surveillance. It is tempting to speculate that the analysis of M1 and M2 TAMs spectrum is likely further controlled by temporal and spatial variables within the microenvironment. Therefore, tumours of different origin are heterogeneous in interaction with the host, based on their ability to release factors such as cytokines into macro and micro-environment to elicit specific host responses, which may be critical for the tumour-promoting activity. Furthermore, clinical studies have demonstrated that the presence of TAMs is strongly correlated with poor prognosis in breast cancer patients indicating advanced tumour progression and metastasis [Citation8], however the underlying mechanism by which TAMs enhance tumor metastasis remain to be established.
We have recently reported that ANXA1 N- terminal cleavage product Ac2-26 can promote M2 polarization through the activation of ERK, Akt and NF-κB (p65) and this is FPR2 dependent [Citation41]. This is in line with previous studies showing that ANXA1 and Lipoxin-A4 induce M2a/M2c-phenotype polarization. [Citation42], and FPR2-deficient macrophages sustain an M1 macrophage phenotype [Citation43]. ANXA1 can increase IL-10 production in macrophages and monocytes, with diminished TNFα and IL-6 production and M1-phenotype polarization [Citation44–Citation46]. In addition, ANXA1-deficient macrophages were shown to express higher levels of TNFα after stimulation with LPS [Citation28]. In our recent study, WT macrophages, but not ANXA1-deficient macrophages, polarized to an alternative M2 phenotype with IL-4, promoted the proliferation and migration of breast cancer cells in a coculture experiment. This therefore suggests that the effect of ANXA1 on macrophages appears to specify a commitment to the M2 lineage in the tumor microenvironment, facilitating tumor progression and metastasis [Citation47]. Thus, ANXA1 can act in two ways: either released from tumor cells or macrophages to subsequently bind to FPR2 on macrophages; or functioning as an intracellular protein in macrophages, resulting in M2 polarization. These studies demonstrate the complex biological functions of ANXA1 on macrophage polarization, and highlight the necessity for further investigation to understand their actions on the tumour microenvironment.
Whether the effects of ANXA1 on macrophage polarization are related to their shared abilities with chemokines (i.e., CCL2, CCL5) or other target molecules, that are required for M2 macrophage polarization-dependent promotion of tumour growth and invasion, remains to be established. It is important to note that ANXA1 is highly expressed in metastatic and TNBC cells, of which migration capability is shown to be dependent on TAM phenotypes and function.
ANXA1 pro-tumour evidence in breast cancer
The expression of ANXA1 has been studied in many types of cancer [Citation18]. ANXA1 is over-expressed in specific types of cancer, while others show consistent loss in expression. There is also increasing evidence to indicate that the changes in ANXA1 expression contribute to the development and progression of cancer. (Reviewed in [Citation18,Citation48]) In breast cancer, reports are conflicting, where ANXA1 expression has been shown to be increased in some ductal carcinomas [Citation49,Citation50], and decreased in others [Citation51,Citation52]. However, many reports have shown higher ANXA1 expression in basal-like breast cancer, particularly TNBC [Citation53,Citation54]. In addition, ANXA1 was high in patients with familial breast cancer associated with BRCA1/2 mutation, poorly differentiated, TNBC and cancers which developed at a young age [Citation55]. Higher levels of ANXA1 were observed in metastatic tumours and lymph nodes compared with primary tumours [Citation51] and thus, ANXA1 was implicated to have a possible role in malignant tumor transformation and metastasis [Citation53,Citation54]. ANXA1 was shown to be a predictor of survival in HER2+ patients, where high levels of ANXA1 was specifically associated with an increased mortality in HER2+ patients, and shown to be a predictor of survival [Citation55,Citation56].
The contributing roles of ANXA1 in breast cancer proliferation and growth are still unclear. ANXA1 has anti-proliferative functions, linked to constitutive activation of the mitogen-activated protein kinase (MAPK) pathway, a pathway associated with complex cellular processes like cell proliferation, differentiation and development [Citation57,Citation58]. ANXA1 contains a src-homology 2 domain and has been shown to bind to the Grb-2 adaptor protein, and can inhibit cyclin D1 and regulate extracellular signal–regulated kinase (ERK)-mediated disruption of the actin cytoskeleton [Citation59]. In breast cancer cell lines, silencing ANXA1 suppressed estrogen-dependent proliferation and growth inhibition. However, since ANXA1 expression is lost in ER+ cancers, its protective role against highly proliferative breast cancer cells in an environment with high estrogen levels may be lost [Citation60]. In addition, ANXA1 enhances mitogen (including estradiol, EGF and FCS) induced proliferation of breast cancer cells through FPR1 and FPR2 [Citation61].
Although several signalling mechanisms have been implicated in facilitating the dispersal of cancer, there has been a growing recognition that this is a selective process governed by directional migratory cues [Citation62]. In line with these findings, we previously demonstrated that ANXA1 enhances transcription factor NF-kB activation and promotes migration capability and metastasis through interactions with upstream proteins NF-kappa-B essential modulator (NEMO, or IKKγ) and Receptor-interacting serine/threonine-protein kinase 1 (RIP1) [Citation53]. In normal cells or tumour cells which express low levels of ANXA1, the inhibitor of kappa B (IκB) kinase (IKK) complex normally regulates IκB activation and phosphorylation, which in turn regulates NF-kB activity. In metastatic tumour cells that express high levels of ANXA1, ANXA1 can interact with NEMO and RIP1 in a complex and stabilizes the IKK complex, resulting in constitutive activation of NF-kB with high invasive and metastatic growth properties [Citation53]. (), Furthermore, ectopic over-expression of intracellular ANXA1 promoted cell migration and inhibited focal adhesion and stress fibre formation through ERK and RhoA activation [Citation63]. ANXA1 can promote metastasis through the enhancement of actin reorganization and transforming growth factor (TGF-β) signalling which activates epithelial mesenchymal transition [Citation54]. Proteomic studies on murine mammary gland cells expressing or lacking ANXA1 revealed that proteins involved in migration and motility were most up-regulated in ANXA1-deficient cells [Citation64] and cells expressing ANXA1 exhibited higher motility and wound healing rates. Further, ANXA1 was shown to increase NF-kB and c-Myc activity leading to the inhibition of microRNA mir196a transcription, inducing a negative feedback loop to promote breast cancer migration and metastasis [Citation53,Citation65], (), Similar observations were noted in other tumour cell models, in which ANXA1 was associated with an enhanced migratory profile, as well as elevated mTOR signalling (a central regulator of cell metabolism, proliferation and survival) and matrix metalloproteinase (MMP9) activity, which are important regulators of degradation of the extracellular matrix [Citation63,Citation66].
Figure 1. The potential influence of ANXA1 on macrophage polarization and breast cancer migration. Monocytes differentiate into polarized macrophage phenotype when exposed to different stimuli. In the presence of granulocyte-macrophage colony stimulating factor (GM-CSF), interferon (IFN-γ), lipopolysaccharide (LPS) and other bacterial products monocytes differentiate into M1 macrophages phenotype. In the presence of macrophage colony stimulating factor (M-CSF), interleukin (IL-4, IL10) and immune-suppressive agents (steroids, prostaglandins), monocytes differentiate to M2-phenotype. ANXA1 may be required for M2 macrophage polarization-dependent promotion of tumour growth and invasion. On the cancer cell, ANXA1 can promote breast cancer migration through its association with NEMO and RIP1 to constitutively activate NF-kB, which in turn can enhance ERK activation to promote migration. It can also enhance TGF-β/SMAD-dependent signalling to induce an epithelial mesenchymal transition and promote migration capability. ANXA1 has also been shown to inhibit focal adhesions and stress fibre formation which is related to lower adhesion and faster migration. ANXA1 can regulate the expression of microRNAs such as miR196a, an anti-migratory microRNA, which forms a negative feedback loop to enhance breast cancer migration.
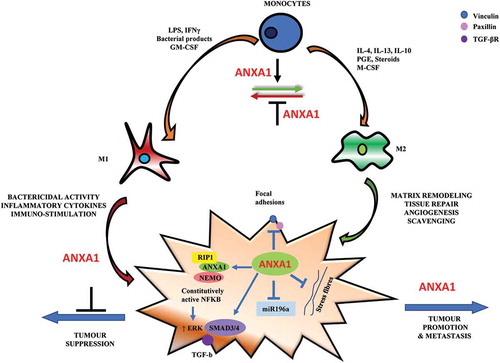
Although intracellular ANXA1 is involved in the invasion process by promoting actin reorganization, NF-kB translocation and MMP9 expression [Citation53,Citation63,Citation67], externalized ANXA1 is implicated in tumour growth by FPRs. ANXA1 triggers FPR2 and to a lesser extent FPR1 promoting mitogenesis by increasing cyclin D1 levels via activation of the phosphoinositide 3-kinase/protein kinase B signalling pathway [Citation61]. FPR2 has been shown to promote metastasis of colon cancer, however, the roles of FPR2 in breast cancer are not clear.
Conclusion
In conclusion, ANXA1 can act endogenously in the tumours to enhance migration and metastasis. ANXA1 can promote breast cancer migration through its association with NEMO and RIP1 to constitutively activate NF-kB [Citation52], which in turn can enhance ERK activation to promote migration [Citation62]. It can also enhance TGF-β-dependent signalling to induce an epithelial mesenchymal transition and promote migration capability [Citation53]. ANXA1 has also been shown to inhibit focal adhesions and stress fibre formation which is related to lower adhesion and faster migration [Citation63]. ANXA1 can also regulate the expression of microRNAs such as miR196a, an anti-migratory microRNA, which forms a negative feedback loop to enhance breast cancer migration [Citation65] (). In the presence of pro-inflammatory cytokines and other bacterial products, monocytes can differentiate into M1 macrophages phenotype. In the presence of anti-inflammatory cytokines and immune-suppressive agents (steroids, prostaglandins), monocytes can differentiate to M2-phenotype. ANXA1 and FPR2 may be important for M2 macrophage- dependent promotion of tumour growth and invasion. In view of this, it is important to look at the tumor microenvironment as a whole and not to focus on specific cell types in the development of novel anti-tumor therapies. Due to their overall pro-tumoral effects, targeting ANXA1 or FPR2 in macrophages could be a useful and promising strategy for new anti-tumoral therapies. Inhibition of FPR2 in macrophages using peptide antagonists or inhibiting ANXA1 expression therapeutically could gear macrophages to a more M1 phenotype, and subsequently inhibiting tumor growth and metastasis.
Disclosure statement
No potential conflict of interest was reported by the authors.
Additional information
Funding
References
- Panupinthu N, Lee HY, Mills GB. Lysophosphatidic acid production and action: critical new players in breast cancer initiation and progression. Br J Cancer. 2010;102:941–946.
- Pernas Simon S. Neoadjuvant therapy of early stage human epidermal growth factor receptor 2 positive breast cancer: latest evidence and clinical implications. Ther Adv Med Oncol. 2014;6:210–221.
- Mantovani A. Cancer: inflammation by remote control. Nature. 2005;435:752–753.
- Federico A, Morgillo F, Tuccillo C, et al. Chronic inflammation and oxidative stress in human carcinogenesis. Int J Cancer. 2007;121:2381–2386.
- Cole SW. Chronic inflammation and breast cancer recurrence. J Clin Oncol. 2009;27:3418–3419.
- Mantovani A, Sozzani S, Locati M, et al. Macrophage polarization: tumor-associated macrophages as a paradigm for polarized M2 mononuclear phagocytes. Trends Immunol. 2002;23:549–555.
- Sica A, Mantovani A. Macrophage plasticity and polarization: in vivo veritas. J Clin Invest. 2012;122:787–795.
- Bingle LBN, Lewis CE. The role of tumor-associated macrophages in tumor progression: implications for new anticancer therapies. J Pathol. 2002;196:254–265.
- Balkwill FR, Capasso M, Hagemann T. The tumor microenvironment at a glance. J Cell Sci. 2012;125:5591–5596.
- Murray PJ, Wynn TA. Protective and pathogenic functions of macrophage subsets. Nat Rev Immunol. 2011;11:723–737.
- Pittet MJ. Behavior of immune players in the tumour microenvironment. Curr Opin Oncol. 2009;21:53–59.
- Lichtenberger BM, Tan PK, Niederleithner H, et al. Autocrine VEGF signaling synergizes with EGFR in tumor cells to promote epithelial cancer development. Cell. 2010;140:268–279.
- Blackwell GJ, Carnuccio R, Di Rosa M, et al. Macrocortin: a polypeptide causing the anti-phospholipase effect of glucocorticoids. Nature. 1980;287:147–149.
- Hall SC, Smith DM, Masiarz FR, et al. Mass spectrometric and Edman sequencing of lipocortin I isolated by two-dimensional SDS/PAGE of human melanoma lysates. Proc Natl Acad Sci USA. 1993;90:1927–1931.
- Rosengarth A, Luecke H. A calcium-driven conformational switch of the N-terminal and core domains of annexin A1. J Mol Biol. 2003;326:1317–1325.
- Christmas P, Callaway J, Fallon J, et al. Selective secretion of annexin 1, a protein without a signal sequence, by the human prostate gland. J Biol Chem. 1991;266:2499–2507.
- Ye RD, Boulay F, Wang JM, et al. International union of basic and clinical pharmacology. LXXIII. Nomenclature for the formyl peptide receptor (FPR) family. Pharmacol Rev. 2009;61:119–161.
- Lim LH, Pervaiz S. Annexin 1: the new face of an old molecule. FASEB J. 2007;21:968–975.
- Chatterjee BE, Yona S, Rosignoli G, et al. Annexin 1-deficient neutrophils exhibit enhanced transmigration in vivo and increased responsiveness in vitro. J Leukoc Biol. 2005;78:639–646.
- Petrella A, Festa M, Ercolino SF, et al. Induction of annexin-1 during TRAIL-induced apoptosis in thyroid carcinoma cells. Cell Death Differ. 2005;12:1358–1360.
- Scannell M, Maderna P. Lipoxins and annexin-1: resolution of inflammation and regulation of phagocytosis of apoptotic cells. ScientificWorldJournal. 2006;6:1555–1573.
- La M, D’Amico M, Bandiera S, et al. Annexin 1 peptides protect against experimental myocardial ischemia-reperfusion: analysis of their mechanism of action. FASEB J. 2001;15:2247–2256.
- Lim LH, Solito E, Russo-Marie F, et al. Promoting detachment of neutrophils adherent to murine postcapillary venules to control inflammation: effect of lipocortin 1. Proc Natl Acad Sci USA. 1998;95:14535–14539.
- Scannell M, Flanagan MB, deStefani A, et al. Annexin-1 and peptide derivatives are released by apoptotic cells and stimulate phagocytosis of apoptotic neutrophils by macrophages. J Immunol. 2007;178:4595–4605.
- Minghetti L, Nicolini A, Polazzi E, et al. Down-regulation of microglial cyclo-oxygenase-2 and inducible nitric oxide synthase expression by lipocortin 1. Br J Pharmacol. 1999;126:1307–1314.
- Blume KE, Soeroes S, Waibel M, et al. Cell surface externalization of annexin A1 as a failsafe mechanism preventing inflammatory responses during secondary necrosis. J Immunol. 2009;183:8138–8147.
- Huggins A, Paschalidis N, Flower RJ, et al. Annexin-1-deficient dendritic cells acquire a mature phenotype during differentiation. FASEB J. 2009;23:985–996.
- Bist P, Shu S, Lee H, et al. Annexin-A1 regulates TLR-mediated IFN-beta production through an interaction with TANK-binding kinase 1. J Immunol. 2013;191:4375–4382.
- Jorge YC, Mataruco MM, Araujo LP, et al. Expression of annexin-A1 and galectin-1 anti-inflammatory proteins and mRNA in chronic gastritis and gastric cancer. Mediators Inflamm. 2013;2013:152860.
- Sica A, Schioppa T, Mantovani A, et al. Tumour-associated macrophages are a distinct M2 polarised population promoting tumour progression: potential targets of anti-cancer therapy. Eur J Cancer. 2006;42:717–727.
- Davies LC, Jenkins SJ, Allen JE, et al. Tissue-resident macrophages. Nat Immunol. 2013;14:986–995.
- Gordon S, Martinez FO. Alternative activation of macrophages: mechanism and functions. Immunity. 2010;32:593–604.
- Balkwill F, Mantovani A. Inflammation and cancer: back to Virchow? Lancet. 2001;357:539–545.
- Gajewski TF, Meng Y, Harlin H. Immune suppression in the tumor microenvironment. J Immunother. 2006;29:233–240.
- Sironi M, Martinez FO, D’Ambrosio D, et al. Differential regulation of chemokine production by Fcgamma receptor engagement in human monocytes: association of CCL1 with a distinct form of M2 monocyte activation (M2b, Type 2). J Leukoc Biol. 2006;80:342–349.
- Martinez FO, Sica A, Mantovani A, et al. Macrophage activation and polarization. Front Biosci. 2008;13:453–461.
- Sica A, Allavena P, Mantovani A. Cancer related inflammation: the macrophage connection. Cancer Lett. 2008;267:204–215.
- Qian BZ, Pollard JW. Macrophage diversity enhances tumor progression and metastasis. Cell. 2010;141:39–51.
- Bronte V, Zanovello P. Regulation of immune responses by L-arginine metabolism. Nat Rev Immunol. 2005;5:641–654.
- Movahedi K, Laoui D, Gysemans C, et al. Different tumor microenvironments contain functionally distinct subsets of macrophages derived from Ly6C(high) monocytes. Cancer Res. 2010;70:5728–5739.
- Ampomah PB, Moraes LA, Lukman HM, et al. Formyl peptide receptor 2 is regulated by RNA mimics and viruses through an IFN-beta-STAT3-dependent pathway. FASEB J. 2018;32:1468–1478.
- Li Y, Cai L, Wang H, et al. Pleiotropic regulation of macrophage polarization and tumorigenesis by formyl peptide receptor-2. Oncogene. 2011;30:3887–3899.
- Liu Y, Chen K, Wang C, et al. Cell surface receptor FPR2 promotes antitumor host defense by limiting M2 polarization of macrophages. Cancer Res. 2013;73:550–560.
- Ferlazzo V, D’Agostino P, Milano S, et al. Anti-inflammatory effects of annexin-1: stimulation of IL-10 release and inhibition of nitric oxide synthesis. Int Immunopharmacol. 2003;3:1363–1369.
- Cooray SN, Gobbetti T, Montero-Melendez T, et al. Ligand-specific conformational change of the G-protein-coupled receptor ALX/FPR2 determines proresolving functional responses. Proc Natl Acad Sci USA. 2013;110:18232–18237.
- Locatelli I, Sutti S, Jindal A, et al. Endogenous annexin A1 is a novel protective determinant in nonalcoholic steatohepatitis in mice. Hepatology. 2014;60:531–544.
- Moraes LA, Kar S, Foo SL, et al. Annexin-a1 enhances breast cancer growth and migration by promoting alternative macrophage polarization in the tumour microenvironment. Sci Rep. 2017;7:17925.
- Lim LH, Pervaiz S. Annexin 1: the new face of an old molecule. FASEB J. 2007;21:968–975.
- Ahn SH, Sawada H, Ro JY, et al. Differential expression of annexin I in human mammary ductal epithelial cells in normal and benign and malignant breast tissues. Clin Exp Metastasis. 1997;15:151–156.
- Pencil SD, Toth M. Elevated levels of annexin I protein in vitro and in vivo in rat and human mammary adenocarcinoma. Clin Exp Metastasis. 1998;16:113–121.
- Shen DJNF, Elshimali Y, Lonsberry V, et al. Decreased expression of annexin A1 is correlated with breast cancer development and progression as determined by a tissue microarray analysis. Hum Pathol. 2006;37:1583–1591.
- Maschler S, Gebeshuber CA, Wiedemann EM, et al. Annexin A1 attenuates EMT and metastatic potential in breast cancer. EMBO Mol Med. 2010;2:401–414.
- Bist P, Leow SC, Phua QH, et al. Annexin-1 interacts with NEMO and RIP1 to constitutively activate IKK complex and NF-kappaB: implication in breast cancer metastasis. Oncogene. 2011;30:3174–3185.
- de Graauw M, van Miltenburg MH, Schmidt MK, et al. Annexin A1 regulates TGF-beta signaling and promotes metastasis formation of basal-like breast cancer cells. Proc Natl Acad Sci USA. 2010;107:6340–6345.
- Sobral-Leite M, Wesseling J, Smit VT, et al. Annexin A1 expression in a pooled breast cancer series: association with tumor subtypes and prognosis. BMC Med. 2015;13:156.
- Yom CK, Han W, Kim SW, et al. Clinical significance of annexin A1 expression in breast cancer. J Breast Cancer. 2011;14:262–268.
- Alldridge LC, Harris HJ, Plevin R, et al. The annexin protein lipocortin 1 regulates the MAPK/ERK pathway. J Biol Chem. 1999;274:37620–37628.
- Zhang W, Liu HT. MAPK signal pathways in the regulation of cell proliferation in mammalian cells. Cell Res. 2002;12:9–18.
- Alldridge LC, Bryant CE. Annexin 1 regulates cell proliferation by disruption of cell morphology and inhibition of cyclin D1 expression through sustained activation of the ERK1/2 MAPK signal. Exp Cell Res. 2003;290:93–107.
- Ang EZ, Nguyen HT, Sim HL, et al. Annexin-1 regulates growth arrest induced by high levels of estrogen in MCF-7 breast cancer cells. Mol Cancer Res. 2009;7:266–274.
- Khau T, Langenbach SY, Schuliga M, et al. Annexin-1 signals mitogen-stimulated breast tumor cell proliferation by activation of the formyl peptide receptors (FPRs) 1 and 2. FASEB J. 2011;25:483–496.
- Strieter RM. Chemokines: not just leukocyte chemoattractants in the promotion of cancer. Nat Immunol. 2001;2:285–286.
- Bist P, Phua QH, Shu S, et al. Annexin-A1 controls an ERK-RhoA-NFkappaB activation loop in breast cancer cells. Biochem Biophys Res Commun. 2015;461:47–53.
- Swa HL, Blackstock WP, Lim LH, et al. Quantitative proteomics profiling of murine mammary gland cells unravels impact of annexin-1 on DNA damage response, cell adhesion, and migration. Mol Cell Proteomics. 2012;11:381–393.
- Yuan Y, Anbalagan D, Lee LH, et al. ANXA1 inhibits miRNA-196a in a negative feedback loop through NF-kB and c-Myc to reduce breast cancer proliferation. Oncotarget. 2016;7:27007–27020.
- Bhardwaj A, Ganesan N, Tachibana K, et al. Annexin A1 preferentially predicts poor prognosis of basal-like breast cancer patients by activating mTOR-S6 signaling. PLoS One. 2015;10:e0127678.
- Kang H, Ko J, Jang SW. The role of annexin A1 in expression of matrix metalloproteinase-9 and invasion of breast cancer cells. Biochem Biophys Res Commun. 2012;423:188–194.