ABSTRACT
Heparin, including unfractionated heparin (UFH), low-molecular-weight heparin (LMWH) and heparin derivatives, are commonly used in venous thromboembolism treatment and reportedly have beneficial effects on cancer survival. Heparin can affect the proliferation, adhesion, angiogenesis, migration and invasion of cancer cells via multiple mechanisms. The main mechanisms involve inhibition of heparanase, P-/L-selectin, angiogenesis, and interference with the CXCL12-CXCR4 axis. Here we summarize the current experimental evidence regarding the anti-cancer role of heparin and its derivatives, and conclude that there is evidence to support heparin’s role in inhibiting cancer progression, making it a promising anti-cancer agent.
Introduction
While humans enjoy an unprecedented level of technological advancement that supports increasing lifespan, researchers continue to struggle to find treatments to combat the rising incidence of cancers. Lung cancer is the leading cause of cancer death in men worldwide and has surpassed breast cancer to be the leading cause of cancer death in women in developed countries. However, the mortality rate of breast cancer is still the highest among all cancers in women of less developed countries. In addition, colorectal cancer, liver cancer, stomach cancer, cervical cancer, pancreatic cancer, and prostate cancer all present major worldwide threat to human health [Citation1]. Conventional cancer treatments including surgical resection, chemotherapy, target therapy, radiation therapy, and immunotherapy, have all achieved positive results. Nevertheless, these treatments are not effective for a substantial number of patients with advanced or drug-resistant cancer, and there is a pressing need to develop alternative treatments. A novel potential has arisen from the coincidental need to treat cancer patients for blood hypercoagulability. Patients with advanced cancer including multiple metastases are often required to spend long stretches of time in bed, significantly increasing the risk of venous thromboembolism. Heparin, a polydisperse mixture of glycosaminoglycans (GAGs) has strong anticoagulant effects, and along with its derivatives is widely used in anticoagulation treatment to prevent venous thromboembolism. Serendipitously, when treating at-risk cancer patients, heparin and related drugs have been found to have anti-cancer functions. In this article we review the current evidence that heparin and its derivatives have anti-cancer properties, and we highlight both the potential for heparin in cancer treatments, and the challenges to its successful application.
Chemical characteristics of heparin and its derivatives
Heparin is a complex mixture of natural GAG isolated from porcine intestine and is usually prepared as a sodium salt. Heparin is classified into two types, unfractionated heparin (UFH) and low-molecular-weight heparin (LMWH), with the latter type including a number of subtypes such as enoxaparin, nadroparin calcium, dalteparin sodium, and tinzaparin. With the development of modern biosynthesis methods, many new types of heparin have been synthetically modified by adding or replacing some heparin chemical groups ()[Citation2]. Summary of all abbreviations used in this review is presented in .
Figure 1. Molecular structure of heparin and its derivatives. (a) A representative monomeric chemical structure of glycosaminoglycan (GAG) and LMWH. (b) Chemical structure of PG545.
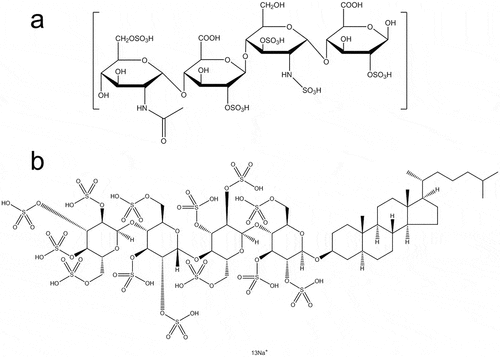
Heparin derivatives include heparin-like glycosaminoglycans (HLGAGs), sulfated non-anticoagulant heparin (S-NACH), low-molecular-weight heparin-taurocholate-tetramer deoxycholate (LHTD4), LHTD4/DCK (a complex of LHTD4 and deoxycholylethylamine DCK), high-molecular-weight Escherichia coli K5-derived heparin-like polysaccharide (K5-NSOS), LHsura (a complex of heparin and suramin fragment), and LHbisD4 (a conjugation of low molecular weight heparin and four bis-deoxycholates). Heparin binds with a wide range of proteins, so that it has a diverse ability to regulate protein functions.
Anti-cancer ability of heparin and its derivatives
While heparin and its derivatives can benefit cancer patients as anticoagulants, they directly impact on cancer progression via anti-metastatic effects [Citation3–Citation5]. Compared with UFH, LMWH can improve the 3-month and 6-month survival of cancer patients [Citation6–Citation11], and HLGAGs reportedly have a similar effect [Citation12,Citation13]. Initially it was thought that heparin’s anti-metastatic effects were via antithrombotic mechanisms, but more recent research suggests that the anti-cancer effect reflects an independent property [Citation14–Citation18]. A multicenter clinical trial exploring the influence of anticoagulant treatment in 277 small cell lung cancer patients showed that 5-week subcutaneous heparin treatment led to substantially improved survival rates compared to no treatment at 1, 2 and 3 years (40% vs. 30%, 11% vs. 9%, and 9% vs. 6%, respectively) [Citation19]. Another clinical study found that death rates in ovarian cancer patients at a 2-year postoperative follow-up were 24% following treatment with certoparin compared to 37.5% following treatment with unfractionated heparin (UFH), suggesting that LMWHs are better than unfractionated heparin (UFN) in improving survival rates [Citation20]. Additional studies have found evidence that heparin and its derivatives reduce the emergence of metastatic lesions and prolong survival in cancer patients.
Taken together, extant studies suggest that heparin and its derivatives confer a survival benefit in cancer, and optimizing the potential for effective treatment requires understanding the underlying mechanisms. A range of studies suggest heparins suppress tumor growth and metastasis by inhibiting tumor growth factors or angiogenesis, suppressing lymphatic vessel formation, reversing multidrug resistance, generating heparinase and thrombin, or inhibiting adherence of cancer cells to vascular endothelium [Citation21–Citation23]. Moreover, different heparin derivatives target specific biological mechanisms to inhibit tumors ().
Table 2. Heparin and its derivatives in different tumors and related mechanisms.
Table 1. The abbreviations and their corresponding full names in articles.
Heparins act as lymphatic vessel suppressants and angiogenesis inhibitors
Heparin’s biological activity includes inhibition of angiogenesis and lymphogenesis [Citation24], and a number of studies indicate that heparin and its derivatives function as tumor lymphatic vessel and angiogenesis suppressants across a range of cancers. These findings may indicate novel therapeutically-relevant mechanisms by which heparins suppress metastasis. Clinical studies have found that the incidence of cancer metastasis through the lymphatic vessels is 3–5 times higher than through the blood vessels. The vascular endothelial growth factor C (VEGF-C)/vascular endothelial growth factor receptor 3 (VEGFR-3) axis plays an important role in lymphangiogenesis. When VEGFR-3 is phosphorylated by its ligand, a series of downstream signaling pathways trigger lymphangiogenesis, including lymphatic endothelial cell proliferation, migration, and tubular formation [Citation25,Citation26]. Recently, researchers found that LHbisD4, a conjugation made up of LMWH and four bis-deoxycholates, inhibits the formation of new lymphatic vessels by suppressing the phosphorylation of VEGFR-3 induced by VEGF-C [Citation27]. In an in vitro study, researchers found that compared with LMWH, in the LHbisD4 treatment group the binding affinity with VEGF-C is significantly higher, but the proliferation, migration and formation of tubular structures are markedly lower. In a 4T1 mouse breast cancer model, LHbisD4 or saline were administrated via an oral route daily for 4 weeks. When the primary 4T1 tumor volume reached 150–200 mm3, the lymph node volume indicated that distant metastasis was significantly reduced in the LHbisD4 treatment group compared to the control group. Likewise, LHbisD4 orsaline were administrated via an oral route daily for 8 weeks in an MDA-MB-231 human breast cancer model. The results demonstrated that LHbisD4 stops cancer cells from metastasizing to lymph nodes, so that the volume of lymph nodes does not increase significantly. These findings indicate that heparin and its derivatives are promising candidates for blocking the VEGF-C/VEGFR-3 axis, which can act to reduce lymph node metastasis [Citation28,Citation29].
While there is encouraging evidence of heparin’s ability to inhibit lymphogenesis, other studies have focused on its ability to inhibit angiogenesis. For example, in murine squamous cell carcinoma LHTD4/DCK inhibits tumor growth significantly at a 5 mg/kg dose, with a final tumor volume of 346.9 ± 25.23 mm3 in the treatment group, compared with 2561.84 ± 161.65 mm3 in the control group [Citation30]. The mechanism underlying this effect appeared to be that LHTD4/DCK inhibited angiogenesis, as the mean blood vessel volume in the LHTD4/DCK group was 8.35 ± 0.4 mm3, much lower than 76.19 ± 3.9 mm3 in the control group [Citation30]. Yin et al. compared a LMWH and adriamycin combined therapy to adriamycin alone, and found that the combined therapy decreased the lung metastasis of breast cancer cells in C3 H mice, and that heparin inhibited vascular endothelial growth factor (VEGF) expression in tumor tissue and induced cancer cell apoptosis [Citation31]. Another study comparing the effects of LWMH with LHsura found that both inhibit angiogenesis, but the effect of LHsura is much stronger. In a study using human umbilical vein endothelial cells (HUVECs), it was found that LHsura inhibited proliferation, migration and the capillary-like structure formation induced by recombinant VEGF165, i.e., simvastatin [Citation32]. The tubular formation inhibitory rate following 50 μg/mL LHsura was 46.4%, compared with 78.6% following 50 μg/mL LMWH. There is a so-called heparin-binding domain (HBD) within the VEGF165 molecular structure, which is a 55-residue carboxy-terminal. These enhanced effects were due to the improved affinity of HBD for VEGF165 via conjugation with suramin fragments. In addition to inhibiting angiogenesis, heparin and its derivatives can also act to protect the endothelial barrier. For example, the LMWH tinzaparin was found to attenuate VEGF-induced endothelial barrier permeability in a manner that does not depend on its anticoagulant activity [Citation33].
Heparanase inhibitors as anti-cancer therapeutics
Heparanase is the only enzyme that can lyse heparin sulfate proteoglycan protein (HSPG), by breaking down the extracellular matrix (ECM) and basement membrane. Additionally, heparanase is involved in tumor angiogenesis, invasion and metastasis, and a number of studies suggest that heparanase is a viable target for cancer therapy. As a result, several heparin mimetics have been developed to treat cancer [Citation34–Citation36].
Anticoagulant activity is a common side effect associated with heparin mimetics, but a promising heparin mimetic, PG545 was found to exhibit a strong anti-lymphoma effect and display only mild anticoagulant activity. To study the molecular mechanism underlying the pro-apoptotic effect of PG545, several molecules were measured. The results indicated that PG545 elicits apoptosis via activating the NFκB pathway, inducing endoplasmic reticulum (ER) stress and autophagy [Citation37]. Additionally, PG545 has been found to be a highly potent inhibitor of angiogenesis, tumor growth and metastasis in murine models of breast, liver, lung, prostate, colon, head and neck cancers and melanoma. Sorafenib, a tyrosine kinase inhibitor (TKI), is a well-established drug for treating kidney and liver cancer, but showed no antimetastatic ability in a liver cancer model. However, in combination with sorafenib, PG545 demonstrated enhanced antimetastatic activity and enhanced anti-cancer efficiency in a murine liver cancer model [Citation38].
Heparin inhibits the metastasis facilitating effect of platelets
It well known that platelets play a key role in the coagulation process, and there is evidence for platelet abnormity amongst cancer patients. Further studies show platelets act as a bridge connecting cancer cells to the endothelial layer, thereby enhancing cancer cell attachment and metastasis [Citation14,Citation22]. Some researchers hold that selectins in platelets trigger the first step of cell-cell interactions, which is relevant to the initiation of tumor metastasis [Citation10,Citation17]. A recent report found that E-cadherin expression in MPanc96-luc cells increased by 2.0 to 2.5-fold after incubation with either S-NACH or tinzaparin [Citation39]. E-cadherin is a marker protein involved in epithelial mesenchymal transition, a key process during malignant tumors’ development of invasion and migration ability. Thus, the above findings suggest the possibility that heparin has its anti-metastasis effect by decreasing the expression of E-cadherin.
The selectin family contains three members: P-, E-, and L-selectin. P-selectin is expressed in the storage granules of platelets and endothelial cells, resulting in rapid translocation on cell surfaces upon activation [Citation40]. When P-selectin is absent, the platelet-tumor cell microthrombi degree is minimal, and metastatic lesions in the lungs of mice are subsequently reduced [Citation17,Citation41,Citation42]. L-selectin actively recruits leukocytes and constructs a metastatic niche, while E-selectin is present on activated endothelial cells in the metastatic colonization of the liver [Citation43–Citation46]. Nevertheless, P-selectin has superior effects over L-selectin [Citation10]. Recent research suggests that metastatic lesions are attenuated in modified heparin analogues containing mostly P-selectin inhibitory activity and in P-selectin-deficient mice [Citation47]. In pancreatic cancer, S-NACH dose-dependently inhibits the adhesion and invasion of MPanc96 cancer cells to the endothelial layer of the umbilical cord vein [Citation48]. The adhesion and invasion of Mpanc96 cells are mediated by P-selectin and inhibited effectively by S-NACH. Given these results, heparin binds to P-selectin glycoprotein ligand-1 (PSGL-1) and thereby prevents platelets from binding to cancer cells () [Citation14–Citation17]. Despite the different structures, LMWHs, S-NACH and tinzaparin all target P-selectin to markedly inhibit cancer metastasis in a concentration-dependent manner, which is particularly the case for S-NACH [Citation10,Citation14,Citation18,Citation48]. Furthermore, a greater inhibitory effect was associated with a larger average molecular weight. Additionally, the adhesion between human colon adenocarcinoma LS180 cells and immobilized P-selectin is disrupted by heparin [Citation17]. Taken togehter, results from in vivo studies endorse heparin as an efficient inhibitor of selectin-mediated interactions between cancer cells and platelets, providing a possible mechanism for how heparin attenuates cancer metastasis.
Figure 2. (a): P-selectin is present in the α-granules of platelets; (b): P-selectin in α-granules is rapidly translocated to the cell surface after activation; (c): P-selectin binds to P-selectin ligand on the surface of cancer cells to form a platelet-cancer cell complex, mediating adhesion of cancer cells to endothelial cells; (d): Heparin binds to selectin, blocks the formation of complexes, and interrupts the adhesion of cancer cells.
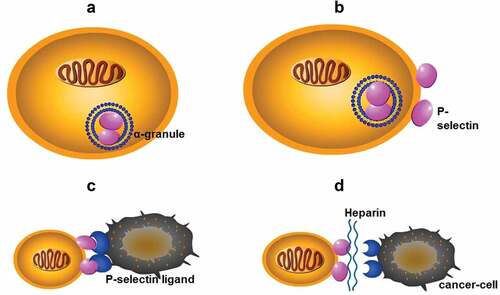
Integrins are receptor molecules involved in cell adhesion and signal transmission. Integrin expression by platelets is thought to be a mechanism by which platelets mediate the adhesion of cancer cells to the extracellular matrix, which promotes cancer metastasis. For example, integrin αIIbβ3 is expressed in platelets and is critical in the interaction of platelets with tumor cells [Citation49,Citation50]. Likewise, integrin αMβ2 (Mac-1) mediates the adherence of hematopoietic progenitor cells to the stromal compartment via binding to heparin and heparan sulfate (HS). Moreover, heparin and modified heparin with low anticoagulant activity can inhibit the adhesion of melanoma A375 cells to platelets, which is mediated by integrin αIIbβ3 [Citation51]. Integrin also inhibits the process by which heparin inhibits melanoma cells from adhering to endothelium. Integrin α4β1 (also known as very late antigen-4; VLA-4) binds to vascular cell adhesion protein-1 (VCAM-1) in B16F10 melanoma cells, so that cancer cells adhere to endothelial cells () [Citation52]. Furthermore, heparin can bind to VLA-4 in human melanoma MV3 cells, with binding affinity in the low micromolar range [Citation53]. Structural analysis confirms heparin can bind to integrin, and binding affinity is affected by molecular size, with some short heparin chain or pentasaccharide (Fondaparinux) unable to bind [Citation54]. Binding affinity is also affected by other factors, such as sulfation density [Citation55].
Heparin and cytokines
Cytokines are small soluble proteins produced by cells that are involved in oncogenesis and the development of cancer. The possible influence of heparin and its derivatives on cytokines has been a focus in understanding how heparin affects cancer cells and cancer progression. Among the different types of cytokines, chemokines are low molecular weight proteins that induce white blood cell migration, which plays an important role in inflammation. They are small, secreted proteins that induce cell migration through activation of G protein-coupled receptors (GPCsR), and bind to extracellular matrix GAG in order to direct chemotaxis along a gradient of increasing chemokine concentration. A substantial number of studies highlight the involvement of chemokines and their receptors in cancer metastasis. The presentation of chemokines to their receptors relies on GAG components on the cell surface, and GAG-binding is essential for the cell migration stimulated by chemokines [Citation56]. Additional evidence suggests the activity of chemokines is directly regulated by GAGs [Citation57].
The arrangement of conserved cysteine residues near the amino terminus indicates that chemokines consist of four families, C, CC, CXC and CX3 C. Among them, CXC Cytokine Ligand 12 (CXCL12, formerly known as stromal cell-derived factor-1, SDF1) and CXC receptor 4 (CXCR4) comprise the CXCL12-CXCR4 axis, which is widely acknowledged as playing a vital role in cancer metastasis. The CXCL12-CXCR4 axis promotes cancer development mainly through two mechanisms: 1) CXCR4-expressing cells are located in CXCL12-expressing organs; and 2) Elevated CXCL12 levels regulate the survival, growth, metastasis and angiogenesis of cancer cells via paracrine signaling [Citation58,Citation59]. In fact, the CXCL12/CXCR4 axis is involved in various physiological functions, such as the entrance of neutrophils into infection sites, stem cell mobilization and directed migration [Citation60–Citation63]. Recent research indicates that heparin’s anti-metastatic ability may be underpinned by the modulation of the CXCR4-CXCL12 axis [Citation64]. For example, heparin and Tinzaparin reduced the pulmonary metastasis of breast cancer cells that were over expressing CXCR4 by interfering with the interaction of CXCL12 and its receptor CXCR4 [Citation65,Citation66]. In a study of human colon cancer cells HCT-116, their proliferation, adhesion and colony formation were promoted by CXCL12, and this process was inhibited by enoxaparin. Additionally, CXCR4 expression in hepatic sinusoidal endothelial cells is down-regulated along with the significant decrease of hepatic metastasis after enoxaparin treatment [Citation67]. Other evidence suggests that CXCR4 mediates the interactions between cancer cells and stroma cells by combining with its natural ligand CXCL12 [Citation68,Citation69]. The CXCL12-CXCR4 axis also mediates the migration of breast cancer cells and their seeding in distant organ tissues, but heparin blocks this interaction; the specific binding sites are shown in [Citation70]. LMWH binds to a heparin-binding site in CXCL12, making CXCL12 a dimerization shift from the monomer dimer equilibrium (), and LMWH consequently decreases CXCR4-CXCL12 interaction [Citation71].
Figure 5. CXCR4 on tumor cells surface binds to its ligand CXCL12 expressed in endothelial cell membrane to promote the metastasis of tumor cells. LMWH binds to CXCL12 to make it a dimerization state, blocking its binding to CXCR4 and inhibiting metastasis.
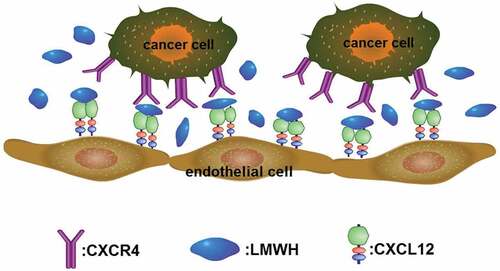
In a study that developed a transplant tumor model of 4T1 breast cancer in mice, treatment with 5 mg/kg/daily LHTD4, taurocholate (TCA) and a tetramer of deoxycholic acid (tetraDOCA) for 8 weeks significantly reduced the formation of metastases [Citation72]. LHTD4 inhibited the migration of MDA-MB-231 cancer cells by blocking the transforming growth factor beta 1 (TGF-β1) signaling pathway and the CXCL12-CXCR4 axis. Specifically, LHTD4 inhibits TGFβ1-mediated phosphorylation of TGF-TGFβ1R1 as well as TGFβ1-induced vimentin and SNAIL-1 expression. Meanwhile, LHTD4 blocks CXCL12-induced CXCR4 phosphorylation and subsequent ligand-receptor response, cell migration and invasion [Citation72].
Heparin promotes bone resorption by enhancing the activity of osteoclasts and inhibits bone formation by weakening the function of osteoblasts. During osteoclastic bone resorption, first TGFβ and then osteolytic factors (e.g. interleukin 11; IL-11) are released. TGFβ regulates several steps in cancer metastasis, including the establishment of bone metastatic lesions. Specifically, UFH is more effective than LMWH at stimulating osteoclast and inhibiting osteoblast activity [Citation73]. K5-NSOS can inhibit TGFβ–induced IL-11, and effectively decrease the osteolytic lesion area and metastatic tumor burden in bones, but markedly alleviates the body weight loss and tumor-related cachexia in a breast cancer bone metastasis mouse model [Citation74].
Heparin prevents cancer relapse by inhibiting cancer stem cells
Inhibiting cancer stem cells (CSCs) is a critical mechanism being proposed for preventing cancer relapse and targeting CSCs is expected to be a promising approach for cancer treatment. Various mechanisms are under consideration in identifying how heparin or heparin-like molecules modulate CSCs. For instance, G2.2, a sulfated nonsaccharide GAG mimetic of heparin hexasaccharide was found to selectively inhibit colonic CSCs in vitro, in vivo and ex vivo. The CSC self-renewal inhibiting function of G2.2 was mediated through p38 MAP kinase activation [Citation75].
As shown in , heparin can inhibit cancer metastasis by blocking the binding of CXCL12 and CXCR4. CXCL12/CXCR4 also mediates the sequestration of the quiescent leukemic stem cells (LSCs) in marrow. These LSCs will cause chemotherapy resistance in acute myelogenous leukemia (AML). CX-01 is a low-anticoagulant heparin derivative that can block CXCL12/CXCR4 activity and therefore disrupt the LSCs in marrow, and CX-01 has been clinically verified to promote chemotherapy efficiency in the treatment of AML [Citation76]. In addition to affecting AML, the same mechanisms support the therapeutic potential of CX-01 for myelodysplastic syndrome, multiple myeloma, and lymphoma.
GAGs HS and chondroitin sulfate (CS) have been reported to regulate self-renewal and pluripotency of CSCs thorough cellular signaling. Recent evidence demonstrates that exogenous CS enhanced hepatoma sphere formation by blocking specific protein binding to CD44, while the addition of exogenous heparin inhibited sphere formation, indicating that heparin and its derivatives are potential candidates for reducing hepatoma stem cells [Citation77].
Other studies focused on the safety of heparin and its derivatives. M. Reza Sadaie [Citation78] demonstrated that heparin and its derivatives could influence the release and expansion of bone CSCs. Bone morphogenetic proteins (BMPs) either potentiate or inhibit the growth of bone CSCs. Heparin and its derivatives bind to BMPs and modulate CSCs positively or negatively, which subsequently has either positive or negative effects on tumorigenesis.
Conclusions and prospects
Heparin is used in the prevention and treatment of venous thromboembolism for cancer patients owing to its strong anticoagulant activity. Clinical studies suggest anticoagulant therapy with heparin leads to better prognosis and survival for patients with diversiform tumors. These findings have revealed that heparin is not only an effective anticoagulant, but may also be an undiscovered novel anti-cancer agent. A growing body of evidence suggests that heparin can decelerate the development of cancer and metastases. However, for both UFH and LMWH, the risk of bleeding caused by anticoagulants limits the application of traditional heparin in cancer treatment, leading to the development of a number of synthetic heparin derivatives. Compared with traditional heparin, these new synthetic heparin derivatives possess much lower anticoagulant activity, but maintain the basic structure and biological functions of heparin. Moreover, the conjugated group also increases the affinity of heparin to its target molecule. As outlined in this article, various types of heparin and its derivatives can inhibit tumor cell proliferation, migration, invasion, and enhance the chemosensitivity of tumor cells. Heparin derivatives modulate hematogenous metastasis by inhibiting the interaction between platelets and tumor cells, and control lymphatic metastasis by inhibiting lymphangiogenesis. Furthermore, heparin derivatives bind to a range of heparin-binding proteins to block signal pathways including TGF-β1, integrins, CXCL12-CXCR4 axis, and VEGF-C/VEGFR-3 axis. Moreover, several mimetics of heparin have been developed as anti-cancer agents. For instance, G2.2 was found to selectively inhibit colonic CSCs in vivo [Citation75]. Due to their potential anti-metastatic ability and good biocompatibility, heparin and its derivatives have been used in the construction process in nanomedicine, with results indicating that nanoparticles based on heparin and its derivatives are promising agents for postoperative chemotherapy [Citation79]. Heparin and its derivatives such as LMWH and S-NACH can enhance the uptake of chemotherapeutics, as demonstrated in an in vivo study with a xenograft cancer model [Citation80]. Consequently, heparin and its derivatives should be developed as promising new adjuvant anti-cancer drugs which can operate via a range of pathways to affect multiple stages of tumor progression.
Disclosure statement
The author reports no conflicts of interest in this work.
Additional information
Funding
References
- Torre LA, Bray F, Siegel RL, et al. Global cancer statistics, 2012. CA Cancer J Clin. 2015;65:87–108.
- Casu B. Structure and biological activity of heparin. Adv Carbohydr Chem Biochem. 1985;43:51–134.
- Klerk CP, Smorenburg SM, Otten HM, et al. The effect of low molecular weight heparin on survival in patients with advanced malignancy. J Clin Oncol. 2005;23:2130–2135.
- Kuderer NM, Khorana AA, Lyman GH, et al. A meta-analysis and systematic review of the efficacy and safety of anticoagulants as cancer treatment: impact on survival and bleeding complications. Cancer. 2007;110:1149–1161.
- Altinbas M, Coskun HS, Er O, et al. A randomized clinical trial of combination chemotherapy with and without low-molecular-weight heparin in small cell lung cancer. J Thromb Haemost. 2004;2:1266–1271.
- Icli F, Akbulut H, Utkan G, et al. Low molecular weight heparin (LMWH) increases the efficacy of cisplatinum plus gemcitabine combination in advanced pancreatic cancer. J Surg Oncol. 2007;95:507–512.
- Prandoni P, Lensing AW, Buller HR, et al. Comparison of subcutaneous low-molecular-weight heparin with intravenous standard heparin in proximal deep-vein thrombosis. Lancet. 1992;339:441–445.
- Gould MK, Dembitzer AD, Doyle RL, et al. Low-molecular-weight heparins compared with unfractionated heparin for treatment of acute deep venous thrombosis. A meta-analysis of randomized, controlled trials. Ann Intern Med. 1999;130:800–809.
- Yoshitomi Y, Nakanishi H, Kusano Y, et al. Inhibition of experimental lung metastases of Lewis lung carcinoma cells by chemically modified heparin with reduced anticoagulant activity. Cancer Lett. 2004;207:165–174.
- Borsig L, Wong R, Hynes RO, et al. Synergistic effects of L- and P-selectin in facilitating tumor metastasis can involve non-mucin ligands and implicate leukocytes as enhancers of metastasis. Proc Natl Acad Sci U S A. 2002;99:2193–2198.
- Green D, Hull RD, Brant R, et al. Lower mortality in cancer patients treated with low-molecular-weight versus standard heparin. Lancet. 1992;339:1476.
- Hettiarachchi RJ, Smorenburg SM, Ginsberg J, et al. Do heparins do more than just treat thrombosis? The influence of heparins on cancer spread. Thromb Haemost. 1999;82:947–952.
- Kakkar AK, Levine MN, Kadziola Z, et al. Low molecular weight heparin, therapy with dalteparin, and survival in advanced cancer: the fragmin advanced malignancy outcome study (FAMOUS). J Clin Oncol. 2004;22:1944–1948.
- Mousa SA, Mohamed S. Inhibition of endothelial cell tube formation by the low molecular weight heparin, tinzaparin, is mediated by tissue factor pathway inhibitor. Thromb Haemost. 2004;92:627–633.
- Wahrenbrock M, Borsig L, Le D, et al. Selectin-mucin interactions as a probable molecular explanation for the association of Trousseau syndrome with mucinous adenocarcinomas. J Clin Invest. 2003;112:853–862.
- Hejna M, Raderer M, Zielinski CC. Inhibition of metastases by anticoagulants. J Natl Cancer Inst. 1999;91:22–36.
- Borsig L, Wong R, Feramisco J, et al. Heparin and cancer revisited: mechanistic connections involving platelets, P-selectin, carcinoma mucins, and tumor metastasis. Proc Natl Acad Sci U S A. 2001;98:3352–3357.
- Stevenson JL, Varki A, Borsig L. Heparin attenuates metastasis mainly due to inhibition of P- and L-selectin, but non-anticoagulant heparins can have additional effects. Thromb Res. 2007;120(Suppl 2):S107–11.
- Lebeau B, Chastang C, Brechot JM, et al. Subcutaneous heparin treatment increases survival in small cell lung cancer. “Petites Cellules” Group. Cancer. 1994;74:38–45.
- von Tempelhoff GF, Harenberg J, Niemann F, et al. Effect of low molecular weight heparin (Certoparin) versus unfractionated heparin on cancer survival following breast and pelvic cancer surgery: A prospective randomized double-blind trial. Int J Oncol. 2000;16:815–824.
- Heinmoller E, Schropp T, Kisker O, et al. Tumor cell-induced platelet aggregation in vitro by human pancreatic cancer cell lines. Scand J Gastroenterol. 1995;30:1008–1016.
- Mousa SA, Petersen LJ. Anti-cancer properties of low-molecular-weight heparin: preclinical evidence. Thromb Haemost. 2009;102:258–267.
- Smorenburg SM, Van Noorden CJ. The complex effects of heparins on cancer progression and metastasis in experimental studies. Pharmacol Rev. 2001;53:93–105.
- Achen MG, Mann GB, Stacker SA. Targeting lymphangiogenesis to prevent tumour metastasis. Br J Cancer. 2006;94:1355–1360.
- Tammela T, Alitalo K. Lymphangiogenesis: molecular mechanisms and future promise. Cell. 2010;140:460–476.
- Stacker SA, Achen MG, Jussila L, et al. Lymphangiogenesis and cancer metastasis. Nat Rev Cancer. 2002;2:573–583.
- Choi JU, Chung SW, Al-Hilal TA, et al. A heparin conjugate, LHbisD4, inhibits lymphangiogenesis and attenuates lymph node metastasis by blocking VEGF-C signaling pathway. Biomaterials. 2017;139:56–66.
- He Y, Kozaki K, Karpanen T, et al. Suppression of tumor lymphangiogenesis and lymph node metastasis by blocking vascular endothelial growth factor receptor 3 signaling. J Natl Cancer Inst. 2002;94:819–825.
- Roberts N, Kloos B, Cassella M, et al. Inhibition of VEGFR-3 activation with the antagonistic antibody more potently suppresses lymph node and distant metastases than inactivation of VEGFR-2. Cancer Res. 2006;66:2650–2657.
- Alam F, Al-Hilal TA, Chung SW, et al. Oral delivery of a potent anti-angiogenic heparin conjugate by chemical conjugation and physical complexation using deoxycholic acid. Biomaterials. 2014;35:6543–6552.
- Yin W, Zhang J, Jiang Y, et al. Combination therapy with low molecular weight heparin and Adriamycin results in decreased breast cancer cell metastasis in C3H mice. Exp Ther Med. 2014;8:1213–1218.
- Park J, Kim J-Y, Hwang SR, et al. Chemical Conjugate of Low Molecular Weight Heparin and Suramin Fragment Inhibits Tumor Growth Possibly by Blocking VEGF165. Mol Pharm. 2015;12:3935–3942.
- Kevane B, Egan K, Allen S, et al. Endothelial barrier protective properties of low molecular weight heparin: A novel potential tool in the prevention of cancer metastasis? Res Pract Thromb Haemost. 2017;1:23–32.
- Al Nahain A, Ignjatovic V, Monagle P, et al. Anticoagulant heparin mimetics via RAFT polymerization. Biomacromolecules. 2020;21:1009–1021.
- Alekseeva A, Mazzini G, Giannini G, et al. Structural features of heparanase-inhibiting non-anticoagulant heparin derivative Roneparstat. Carbohydr Polym. 2017;156:470–480.
- Gomes AM, Kozlowski EO, Borsig L, et al. Antitumor properties of a new non-anticoagulant heparin analog from the mollusk Nodipecten nodosus: effect on P-selectin, heparanase, metastasis and cellular recruitment. Glycobiology. 2015;25:386–393.
- Weissmann M, Bhattacharya U, Feld S, et al. The heparanase inhibitor PG545 is a potent anti-lymphoma drug: mode of action. Matrix Biol. 2019;77:58–72.
- Ferro V, Liu L, Johnstone KD, et al. Discovery of PG545: a highly potent and simultaneous inhibitor of angiogenesis, tumor growth, and metastasis. J Med Chem. 2012;55:3804–3813.
- Alyahya R, Sudha T, Racz M, et al. Anti-metastasis efficacy and safety of non-anticoagulant heparin derivative versus low molecular weight heparin in surgical pancreatic cancer models. Int J Oncol. 2015;46:1225–1231.
- Kansas GS. Selectins and their ligands: current concepts and controversies. Blood. 1996;88:3259–3287.
- Kim YJ, Borsig L, Varki NM, et al. P-selectin deficiency attenuates tumor growth and metastasis. Proc Natl Acad Sci U S A. 1998;95:9325–9330.
- Ludwig RJ, Boehme B, Podda M, et al. Endothelial P-selectin as a target of heparin action in experimental melanoma lung metastasis. Cancer Res. 2004;64:2743–2750.
- Laubli H, Borsig L. Selectins promote tumor metastasis. Semin Cancer Biol. 2010;20:169–177.
- Laubli H, Spanaus KS, Borsig L. Selectin-mediated activation of endothelial cells induces expression of CCL5 and promotes metastasis through recruitment of monocytes. Blood. 2009;114:4583–4591.
- Khatib AM, Kontogiannea M, Fallavollita L, et al. Rapid induction of cytokine and E-selectin expression in the liver in response to metastatic tumor cells. Cancer Res. 1999;59:1356–1361.
- Brodt P, Fallavollita L, Bresalier RS, et al. Liver endothelial E-selectin mediates carcinoma cell adhesion and promotes liver metastasis. Int J Cancer. 1997;71:612–619.
- Hostettler N, Naggi A, Torri G, et al. P-selectin- and heparanase-dependent antimetastatic activity of non-anticoagulant heparins. Faseb J. 2007;21:3562–3572.
- Sudha T, Phillips P, Kanaan C, et al. Inhibitory effect of non-anticoagulant heparin (S-NACH) on pancreatic cancer cell adhesion and metastasis in human umbilical cord vessel segment and in mouse model. Clin Exp Metastasis. 2012;29:431–439.
- Amirkhosravi A, Mousa SA, Amaya M, et al. Inhibition of tumor cell-induced platelet aggregation and lung metastasis by the oral GpIIb/IIIa antagonist XV454. Thromb Haemost. 2003;90:549–554.
- Burdick MM, Konstantopoulos K. Platelet-induced enhancement of LS174T colon carcinoma and THP-1 monocytoid cell adhesion to vascular endothelium under flow. Am J Physiol Cell Physiol. 2004;287:C539–47.
- Zhang C, Liu Y, Gao Y, et al. Modified heparins inhibit integrin alpha(IIb)beta(3) mediated adhesion of melanoma cells to platelets in vitro and in vivo. Int J Cancer. 2009;125:2058–2065.
- Bendas G, Borsig L. Cancer cell adhesion and metastasis: selectins, integrins, and the inhibitory potential of heparins. Int J Cell Biol. 2012;2012:676731.
- Schlesinger M, Simonis D, Schmitz P, et al. Binding between heparin and the integrin VLA-4. Thromb Haemost. 2009;102:816–822.
- Schlesinger M, Naggi A, Torri G, et al. Blocking of integrin-mediated human MV3 melanoma cell binding by commercial and modified heparins. Int J Clin Pharmacol Ther. 2010;48:448–450.
- Schlesinger M, Schmitz P, Zeisig R, et al. The inhibition of the integrin VLA-4 in MV3 melanoma cell binding by non-anticoagulant heparin derivatives. Thromb Res. 2012;129:603–610.
- von Delius S, Ayvaz M, Wagenpfeil S, et al. Effect of low-molecular-weight heparin on survival in patients with advanced pancreatic adenocarcinoma. Thromb Haemost. 2007;98:434–439.
- Handel TM, Johnson Z, Crown SE, et al. Regulation of protein function by glycosaminoglycans–as exemplified by chemokines. Annu Rev Biochem. 2005;74:385–410.
- Teicher BA, Fricker SP. CXCL12 (SDF-1)/CXCR4 pathway in cancer. Clin Cancer Res off J Am Assoc Cancer Res. 2010;16:2927–2931.
- Benovic JL, Marchese A. A new key in breast cancer metastasis. Cancer Cell. 2004;6:429–430.
- White FA, Bhangoo SK, Miller RJ. Chemokines: integrators of pain and inflammation. Nat Rev Drug Discov. 2005;4:834–844.
- Kucia M, Jankowski K, Reca R, et al. CXCR4-SDF-1 signalling, locomotion, chemotaxis and adhesion. J Mol Histol. 2004;35:233–245.
- Kucia M, Ratajczak J, Ratajczak MZ. Bone marrow as a source of circulating CXCR4+ tissue-committed stem cells. Biol Cell. 2005;97:133–146.
- Kucia M, Reca R, Miekus K, et al. Trafficking of normal stem cells and metastasis of cancer stem cells involve similar mechanisms: pivotal role of the SDF-1-CXCR4 axis. Stem Cells. 2005;23:879–894.
- Simka M. Anti-metastatic activity of heparin is probably associated with modulation of SDF-1-CXCR4 axis. Med Hypotheses. 2007;69:709.
- Harvey JR, Mellor P, Eldaly H, et al. Inhibition of CXCR4-mediated breast cancer metastasis: a potential role for heparinoids? Clinical cancer research: an official. J Am Ass Cancer Res. 2007;13:1562–1570.
- Mellor P, Harvey JR, Murphy KJ, et al. Modulatory effects of heparin and short-length oligosaccharides of heparin on the metastasis and growth of LMD MDA-MB 231 breast cancer cells in vivo. Br J Cancer. 2007;97:761–768.
- Ma L, Qiao H, He C, et al. Modulating the interaction of CXCR4 and CXCL12 by low-molecular-weight heparin inhibits hepatic metastasis of colon cancer. Invest New Drugs. 2012;30:508–517.
- Smith MC, Luker KE, Garbow JR, et al. CXCR4 regulates growth of both primary and metastatic breast cancer. Cancer Res. 2004;64:8604–8612.
- Marchesi F, Monti P, Leone BE, et al. Increased survival, proliferation, and migration in metastatic human pancreatic tumor cells expressing functional CXCR4. Cancer Res. 2004;64:8420–8427.
- Ziarek JJ, Veldkamp CT, Zhang F, et al. Heparin oligosaccharides inhibit chemokine (CXC motif) ligand 12 (CXCL12) cardioprotection by binding orthogonal to the dimerization interface, promoting oligomerization, and competing with the chemokine (CXC motif) receptor 4 (CXCR4) N terminus. J Biol Chem. 2013;288:737–746.
- Veldkamp CT, Peterson FC, Pelzek AJ, et al. The monomer-dimer equilibrium of stromal cell-derived factor-1 (CXCL 12) is altered by pH, phosphate, sulfate, and heparin. Protein Sci. 2005;14:1071–1081.
- Alam F, Al-Hilal TA, Park J, et al. Multi-stage inhibition in breast cancer metastasis by orally active triple conjugate, LHTD4 (low molecular weight heparin-taurocholate-tetrameric deoxycholate). Biomaterials. 2016;86:56–67.
- Handschin AE, Trentz OA, Hoerstrup SP, et al. Effect of low molecular weight heparin (dalteparin) and fondaparinux (Arixtra) on human osteoblasts in vitro. Br J Surg. 2005;92:177–183.
- Pollari S, Kakonen RS, Mohammad KS, et al. Heparin-like polysaccharides reduce osteolytic bone destruction and tumor growth in a mouse model of breast cancer bone metastasis. Mol Cancer Res. 2012;10:597–604.
- Boothello RS, Patel NJ, Sharon C, et al. A unique nonsaccharide mimetic of heparin hexasaccharide inhibits colon cancer stem cells via p38 MAP kinase activation. Mol Cancer Ther. 2019;18:51–61.
- Kovacsovics TJ, Mims A, Salama ME, et al. Combination of the low anticoagulant heparin CX-01 with chemotherapy for the treatment of acute myeloid leukemia. Blood Adv. 2018;2:381–389.
- Lin S-C,Wu C-PT, seng T, et al. Role of syndecan-1 and exogenous heparin in hepatoma sphere formation. Biochem Cell Biol. 2020;98(2):112-119.
- Sadaie RM. Can heparins stimulate bone cancer stem cells and interfere with tumorigenesis? Ther Adv Drug Saf. 2011;2:271–282.
- Sun H, Cao D, Wu H, et al. Development of low molecular weight heparin based nanoparticles for metastatic breast cancer therapy. Int J Biol Macromol. 2018;112:343–355.
- Phillips PG, Yalcin M, Cui H, et al. Increased tumor uptake of chemotherapeutics and improved chemoresponse by novel non-anticoagulant low molecular weight heparin. Anticancer Res. 2011;31:411–419.