Abstract
The formation of functional musculoskeletal system relies on proper connectivity between muscles and their corresponding tendon cells. In Drosophila, larval muscles are born during early embryonic stages, and elongate toward tendons that are embedded within the ectoderm in later. The Slit/Robo signaling pathway had been implicated in the process of muscle elongation toward tendons. Here we discuss our recent findings regarding the critical contribution of Slit cleavage for immobilization and stabilization of the Slit signal on the tendon cells. Slit cleavage produces 2 polypeptides, the N-terminal Slit-N, which is extremely stable, undergoes oligomerization, and associates with the tendon cell surfaces, and the C-terminal Slit-C, which rapidly degrades. Slit cleavage leads to immobilization of Slit signaling on tendons, leading to a short-range repulsion, which eventually arrest further muscle elongation. Robo2, which is co-expressed with Slit by the tendon cells facilitates Slit cleavage. This activity does not require the cytoplasmic signaling domain of Robo2. We suggest that Robo2-dependent Slit cleavage, and the formation of Slit-N oligomers on the tendon cell surfaces direct muscle elongation, and provide a stop signal for the approaching muscle, through binding to Robo and Robo3 receptors expressed by the muscles.
In a multicellular organism, proper development of organs or complex tissues depends on the correct migration/elongation of cells and recognition of their final targets. One important example is the musculoskeletal system, where, in order to perform properly, the muscles and tendons must connect in a specific pattern. Each of the Drosophila larval muscles forms during embryogenesis from a single founder cell expressing specific identity genes that fuses with fusion competent myoblasts, and elongates toward its presumptive tendons. Once the muscle has reached the tendon it stops elongating and forms the myotendinous connection.Citation12,13,17 How the different muscles are directed to their correct tendons and what signal arrests their elongation is not fully understood.
Much of what is known about direction of cell elongation and target recognition comes from neurons. In fact a number of signaling molecules known from neuronal directional elongation are also important for proper muscle patterning.Citation5-7,11 One such molecule is Slit. Slit is best characterized in the Drosophila embryonic CNS, where it is expressed by the midline glia and repels axons by binding to its receptor Robo, expressed by neuronal cells. In Drosophila there are 3 Robo receptors: Robo, Robo2, and Robo3. All three receptors can bind Slit and transduce a signal. The overall amount of Robo expressed by the axon determines the extent of its repulsion from the midline. Although in determining the distance from the midline all 3 Robos are interchangeable, for other roles, e.g., neuronal crossing of the midline, they maintain distinct functions.Citation2,3,15 Slit, Robo, and Robo2 are important players in the process of muscle elongation but the exact nature of their contribution has not been directly addressed.Citation7 Slit is expressed by the tendon cells while Robo is expressed in the elongating muscle. Previous work explaining muscle elongation toward tendons suggested that Slit contributes to muscle attraction rather than repulsion, and both Robo and Robo2 function in the elongating muscle to respond to the Slit signal.Citation7 Alternatively, our recent work suggests that Slit represents a short-range repellent and provides a stop signal for the approaching elongating muscle, and thus mechanistically contributes to the collapse of leading edge filopodia, similar to its function in neurons.Citation10
Slit contains a highly conserved cleavage site and undergoes cleavage in vivo in both vertebrates and invertebrates. Slit cleavage produces 2 polypeptides; the N-terminal (Slit-N) contains 4 Leucine-Rich-Repeats (LRR), which includes the Robo binding domain, and 5 EGF repeats; the C-terminal polypeptide includes a laminin G domain and additional 4 EGF repeats.Citation1,9,18 The functional importance of Slit cleavage has not been fully elucidated: Nguen Ba-Charvet et al.9 suggested that olfactory bulb and DRG axons respond differentially to the full-length or to Slit-N. In Drosophila CNS un-cleavable Slit fully rescued the neuronal embryonic CNS phenotype implicating that Slit cleavage is dispensable for proper Drosophila CNS development. In addition, Slit has been proposed to function as a dimer in a number of contexts. Structural crystallographic studies demonstrated the formation of Slit oligomers via the formation of disulfide bonds. The formation of Slit oligomers might promote Robo receptor aggregations.Citation14
In our recent work we describe a model where Slit cleavage represents a key process indispensable for muscle patterning. We propose that Slit cleavage is essential for promoting Slit protein stability, and immobilization on the tendon cell membrane. The immobilized cleaved Slit induces short-range repulsion that directs the elongating muscles to their final destination, and provides a stop signal once the entire muscle leading edge is in contact with Slit on the tendon cell membrane.Citation10 Moreover, we propose that, whereas Robo and Robo3 cooperate to respond to Slit signaling at the muscle leading edge, Robo2 is uniquely expressed by the tendon cells; its activity on these cells contributes to Slit cleavage and does not require its signaling cytoplasmic tail.
The experimental basis for this model relies on utilizing an array of Slit constructs knocked into the Slit locus and the analysis of the consequent muscle extension phenotype measured in fixed, as well as live embryos. We demonstrated that an un-cleavable Slit cannot rescue the slit muscle phenotype, implicating Slit cleavage as an essential process for proper muscle patterning. Next we showed that the cleaved form of Slit remains associated with the tendon-expressing cells, whereas the uncleavable form diffuses and rapidly degrades, implying that Slit cleavage is vital for inducing its specific localization at the tendon cell surfaces. Consistent with this idea the addition of a membrane-anchoring domain of the CD8 receptor to the uncleavable Slit potentiates its rescue ability. This implies that Slit cleavage is required for immobilization of the cleaved Slit-N polypeptide on the tendon cell membrane. We also found that Slit-N is significantly more stable than full length Slit. Furthermore, our recent work suggests that Slit-N, but not uncleavable Slit, preferentially oligomerizes, possibly explaining the enhanced stability of Slit-N polypeptides.19
In order to better understand the activity of Slit in the process of muscle elongation we followed the extension of individual fluorescently labeled muscles in both wild type (WT) and slit mutant live embryos. In WT embryos the 3 lateral transverse muscles elongate in a dorsal-ventral direction in the middle of the segment between 2 rows of Slit-expressing segment border tendon cells. Although the orientation of these muscles is at the dorsal-ventral direction they continuously send membrane extensions laterally toward the segment border tendon cells. These filopodia retract in wild type embryos so that the net muscle elongation is in the dorsal-ventral direction and the muscles are positioned in the center of the segment. In slit mutants these muscles exhibit multiple, misoriented lateral filopodia that do not retract and the final positioning of the muscles turned out to be very close to the posterior segment border, implying that Slit expressed at the segment borders normally induces retraction of the lateral membrane extensions of these muscles. Furthermore, when we followed the dynamics of the DA3 muscle, which elongates diagonally from the anterior to posterior segment border, we noticed that this muscle does not stop at the posterior segment border, and persists in elongating. This phenotype implied that normally Slit signaling is critical for arresting muscle elongation once it reaches the target tendon cell. The role of Slit is therefore critical for inhibiting the formation of un-oriented membrane extensions (similar to its role in neurons) and arresting filopodia formation once the muscle reaches its target tendon cell. To our knowledge this is the first description of Slit as representing a stop signal. Both functions depend on the ability of Slit to induce short-range, localized signals on the tendon cells, emphasizing the importance of Slit immobilization, a process that our data suggest depends on the formation of cleaved Slit-N stable polypeptide, which associates with the tendon cell membrane. A model describing how Slit-N inhibits muscle elongation and provides a stop signal is presented in .
In an attempt to identify the critical Robo receptor in muscles we tested the phenotype of the 3 Robo receptors.19 We found that robo2 mutant embryos exhibited a muscle phenotype reminiscent of that of slit mutants. Surprisingly, Robo2 is expressed by tendons and not by muscles. Furthermore, knock down of Robo2 in tendons recapitulates the muscle phenotype of robo2 mutants, and ectoderm expression of Robo2 partially rescued the robo2 muscle phenotype, implicating a function for Robo2 in tendons rather than in muscles. In contrast to Robo2 our results suggest that Robo and Robo3 are required in the muscles. Further elucidation of the specific role of Robo2 in tendons revealed that overexpression of Robo2 in the ectoderm caused a significant increase in Slit cleavage; also that this capability did not depend on Robo signaling because similar results were obtained using Robo2 that lacks the signaling cytoplasmic-domain. Based on these results and additional data we propose that Robo2 on the tendon cells binds and immobilizes full length Slit, making it available to an unknown protease that then cleaves Slit. The resulting Slit-N polypeptides then oligomerize and bind to an unknown membrane protein on the tendon cell membrane (). Alternatively, Slit cleavage is by default (in cells where the protease is present) but binding of the cleaved Slit-N to Robo2 and additional ECM components at the tendon cell membrane stabilizes the cleaved form. Both models predict that immobilization of the uncleavable Slit protein on the tendon cell membrane would rescue the muscle phenotype of robo2 mutants. Indeed we found that Slit-uncleavable, containing CD8 domain, rescued the robo2 phenotype similar to Slit-N expressed on tendons.
Figure 1. Slit cleavage directs muscle elongation A. upper panel shows the details of Slit cleavage into Slit-Nterminal (Slit-N) and Slit C-terminal (Slit C). The lower panels describe consecutive stages in the elongation of the 3 lateral transvers muscles deduced form the live imaging of these muscles: whereas the leading edges of these muscles are always at their dorsal or ventral edges, lateral filopodia are inhibited by the tendon cells at the segmental borders, leading to net extension in dorsal-ventral direction. When the leading edges are heading the cluster of tendons located in the middle of the segment their elongation is arrested. B. Robo2 binds to Slit in “cis” at the surfaces of the tendon cells. This facilitates Slit cleavage and the formation of Slit-N oligomers, which then associate with the tendon cell membrane possibly by binding to Robo2 and other receptors. Slit-N oligomers signal to the muscle through Robo (Robo+Robo3) receptors at the muscle side and induce repulsion or stop signal.
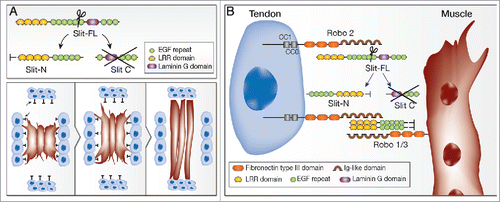
This model raises 3 major questions, namely, what is the identity of the protease and does it exhibit specific tissue distribution; how the cleaved Slit-N associates with the tendon cell membrane; and what is the identity of the receptors responding to the Slit signal.
Although the specific cleavage site of Slit has been characterized, the nature of the protease is still elusive. However, our recent data imply that the protease might be ubiquitous because overexpression of either Robo, Robo2, or Robo2 lacking the cytoplasmic domain in the entire embryonic ectoderm, using the 69B driver which induces expression in tendon cells, promoted enhanced Slit cleavage. Regarding Slit-N immobilization on the tendon cell membrane, we consider several mechanisms, all of which depend on a tendon-specific Slit co-receptor that would bind Slit-N and prevent its diffusion. The proteoglycan Syndecan had been shown to bind Slit in both vertebrates and fly CNS.Citation16 Syndecan is expressed by tendon cells, thus might contribute to Slit immobilization. Multiplexin, a collagenXVIII ortholog, has been recently shown to bind Slit in the fly embryonic heart, and since it is also expressed by tendon cells it might contribute to Slit immobilization on tendons.Citation4 Finally, Robo2 itself may have a role in Slit immobilization. Robo2 had been suggested to act non-autonomously in several setups, e.g., in chordotonal organsCitation8 and in the midlineCitation3; however, the molecular explanation for this activity is still elusive. It is possible that, similarly to tendons, Robo2 contributes to modulation of Slit, affecting its final distribution and possibly cleavage.
Over the last decades it has become clear that a relatively small number of signaling pathways are reused throughout development to direct numerous distinct processes. Regulation at the level of the ligand, receptor, or receptor downstream signaling might differ between distinct tissues: for example, in Slit/Robo signaling Slit cleavage might distinguish between short and long range signaling; axons may respond to Slit long-range repulsion as a result of Slit diffusion from the midline whereas muscles respond to immobilized Slit. The latter setup enables Slit to direct multiple types of muscles to their target tendons in a small field of cells. In addition to directing axonal and muscle tracts Slit is involved in various developmental processes in which it appears to signal between neighboring cells that contact with each other, e.g., heart cardioblasts or trachea cells. In these systems controlling Slit distribution might be a critical factor. Taken together, our studies imply that Slit protein stability and distribution represent an important factor in controlling its spatial and temporal activity and that Slit cleavage and oligomerization are critical in directing Slit spatial activity. We suggest this novel regulatory mechanism might apply to other processes where Slit represents the critical signaling cue.
Disclosure of Potential Conflicts of Interest
No potential conflicts of interest were disclosed.
Acknowledgments
We thank Tali Wiesel from the Weizmann Institute Graphics Department for designing the model.
Funding
This study was supported by the Israeli Science Foundation (ISF, grant number 71/12 to T.V.).
References
- Brose K, Bland KS, Wang KH, Arnott D, Henzel W, Goodman CS, Tessier-Lavigne M, Kidd T. Slit proteins bind Robo receptors and have an evolutionarily conserved role in repulsive axon guidance. Cell 1999; 96:795-806; PMID:10102268; http://dx.doi.org/10.1016/S0092-8674(00)80590-5
- Evans TA, Bashaw GJ. Functional diversity of Robo receptor immunoglobulin domains promotes distinct axon guidance decisions. Curr Biol 2010; 20:567-72
- Evans TA, Santiago C, Arbeille E, Bashaw GJ. Robo2 acts in trans to inhibit Slit-Robo1 repulsion in pre-crossing commissural axons. eLife 2015; 4:e08407; PMID: 26186094
- Harpaz N, Ordan E, Ocorr K, Bodmer R, Volk T. Multiplexin promotes heart but not aorta morphogenesis by polarized enhancement of slit/robo activity at the heart lumen. PLoS Genet 2013; 9:e1003597; PMID:23825967; http://dx.doi.org/10.1371/journal.pgen.1003597
- Kidd T, Bland KS, Goodman CS. Slit is the midline repellent for the robo receptor in Drosophila. Cell 1999; 96:785-94; PMID:10102267; http://dx.doi.org/10.1016/S0092-8674(00)80589-9
- Kidd T, Brose K, Mitchell KJ, Fetter RD, Tessier-Lavigne M, Goodman CS, Tear G. Roundabout controls axon crossing of the CNS midline and defines a novel subfamily of evolutionarily conserved guidance receptors. Cell 1998; 92:205-15; PMID:9458045; http://dx.doi.org/10.1016/S0092-8674(00)80915-0
- Kramer SG, Kidd T, Simpson JH, Goodman CS. Switching repulsion to attraction: changing responses to slit during transition in mesoderm migration. Science 2001; 292:737-40; PMID:11326102; http://dx.doi.org/10.1126/science.1058766
- Kraut R, Zinn K. Roundabout 2 regulates migration of sensory neurons by signaling in trans. Curr Biol 2004; 14:1319-29; PMID:15296748; http://dx.doi.org/10.1016/j.cub.2004.07.052
- Nguyen Ba-Charvet KT, Brose K, Ma L, Wang KH, Marillat V, Sotelo C, Tessier-Lavigne M, Chedotal A. Diversity and specificity of actions of Slit2 proteolytic fragments in axon guidance. J. Neurosci 2001; 21:4281-9
- Ordan E, Brankatschk M, Dickson B, Schnorrer F, Volk T. Slit cleavage is essential for producing an active, stable, non-diffusible short-range signal that guides muscle migration. Development 2015; 142:1431-6; PMID:25813540; http://dx.doi.org/10.1242/dev.119131
- Rothberg JM, Jacobs JR, Goodman CS, Artavanis-Tsakonas S. slit: an extracellular protein necessary for development of midline glia and commissural axon pathways contains both EGF and LRR domains. Genes Dev 1990; 4:2169-87; PMID:2176636; http://dx.doi.org/10.1101/gad.4.12a.2169
- Schejter ED, Baylies MK. Born to run: creating the muscle fiber. Curr. Opin. Cell Biol 2010; 22:566-74
- Schweitzer R, Zelzer E, Volk T. Connecting muscles to tendons: tendons and musculoskeletal development in flies and vertebrates. Development 2010; 137:2807-17; PMID:20699295; http://dx.doi.org/10.1242/dev.047498
- Seiradake E, von Philipsborn AC, Henry M, Fritz M, Lortat-Jacob H, Jamin M, Hemrika W, Bastmeyer M, Cusack S, McCarthy AA. Structure and functional relevance of the Slit2 homodimerization domain. EMBO Rep 2009; 10:736-41; PMID:19498462; http://dx.doi.org/10.1038/embor.2009.95
- Spitzweck B, Brankatschk M, Dickson BJ. Distinct protein domains and expression patterns confer divergent axon guidance functions for Drosophila Robo receptors. Cell 2010; 140:409-20; PMID:20144763; http://dx.doi.org/10.1016/j.cell.2010.01.002
- Steigemann P, Molitor A, Fellert S, Jackle H, Vorbruggen G. Heparan sulfate proteoglycan syndecan promotes axonal and myotube guidance by slit/robo signaling. Curr. Biol 2004; 14:225-30
- Volk T. Singling out Drosophila tendon cells: a dialogue between two distinct cell types. Trends Genet 1999; 15:448-53; PMID:10529807; http://dx.doi.org/10.1016/S0168-9525(99)01862-4
- Wang KH, Brose K, Arnott D, Kidd T, Goodman CS, Henzel W, Tessier-Lavigne M. Biochemical purification of a mammalian slit protein as a positive regulator of sensory axon elongation and branching. Cell 1999; 96:771-84; PMID:10102266; http://dx.doi.org/10.1016/S0092-8674(00)80588-7
- Ordan E, Volk T. Development 2015; 142:3512-8.